Target screening of chemicals of concern in recycled water†
Received
10th December 2014
, Accepted 4th May 2015
First published on 5th May 2015
Abstract
The results of a characterisation study of water samples collected from an Advanced Water Recycling Plant (AWRP) operating in Perth, Western Australia are presented. The AWRP treats secondary wastewater by ultrafiltration, reverse osmosis (RO) and ultraviolet radiation (UV) to produce recycled water for groundwater replenishment. Water samples collected after RO and UV treatment were characterised by liquid chromatography-high resolution mass spectrometry, using an established protocol for target screening. The target screening of 291 compounds detected a total of 13 chemicals in post-RO and post-UV water, including 2 corrosion inhibitors (4+5-methyl-1H-benzotriazole, benzotriazole), 3 pesticides (metolachlor, propiconazol, prosulfocarb), 3 pharmaceuticals (lamotrigin, metformin, tramadol), 1 personal care product (galaxalidone), 3 artificial sweeteners (saccharin, acesulfame, sucralose) and 1 flame retardant (triethyl phosphate). The corrosion inhibitors benzotriazole and 4+5-methyl-1H-benzotriazole, and the pharmaceutical metformin were detected in hundreds of ng L−1, while the other compounds were present in low ng L−1 concentrations. Analysis of UV treated water samples showed that UV treatment also helped to reduced UV degradable compounds such as the corrosion inhibitors (>50% removal), triethyl phosphate (~50% removal) and the artificial sweetener acesulfame (~95% removal). Overall, the detection of 13 chemicals through target screening analyses did not account for the residual dissolved organic carbon (DOC) in post RO water, the majority of which is still an intriguing unknown. However, the target screening did show that there were no obvious “known” anthropogenic contaminants contributing to the majority of the DOC in post-RO and post-UV treated water. Calculated risk quotients (RQ) for all detected chemicals in UV treated water were 2 to 6 order of magnitude below 1, implying an high degree of safety associated with human consumption of recycled water. Overall the chemicals screening provided further evidence of the overall safety of the use of recycled wastewater treated by RO and UV as a potable water source.
Water impact
While research into the chemical safety of recycled water has focussed on measuring chemicals in recycled water, it is not clear what percentage of residual dissolved organic carbon (DOC) in recycled water results from anthropogenic chemicals. Here we show that anthropogenic chemicals do not contribute significantly to residual DOC in recycled water after RO and UV treatment. A risk assessment of the chemicals detected in the recycled water also demonstrates the high degree of safety associated with human consumption of recycled water. However, the study also highlights that end-product compliance testing for all potential chemicals is time consuming and expensive. Further research into the use of bioassays as a monitoring tool for water recycling is recommended.
|
Introduction
In recent years, Western Australia has experienced a significant reduction in rainwater precipitation levels which has corresponded to a reduction in water available from dams and groundwater for drinking water production.1 Use of treated wastewater as a drinking water source is becoming increasingly attractive, both in Australia and worldwide, and demonstrating that specific treatment technologies produce safe drinking water is of high importance, particularly focussing on chemical removal using reverse osmosis (RO) followed by ultraviolet irradiation (UV) for disinfection. Research into the safety of recycled water has focussed on monitoring and characterising residual concentrations of inorganic and organic micropollutants in the finished water.2–4 Chemicals in wastewaters that potentially pose health concerns include heavy metals, organic compounds with suspected carcinogenic properties (e.g. N-nitrosamines and halogenated disinfection by-products), pharmaceuticals and personal care products (e.g. endocrine disrupting compounds, cytostatics and antibiotics), pesticides and their degradation products, and other unregulated trace organic compounds (i.e. plasticisers, surfactants, musk fragrances, artificial sweeteners) derived from both domestic and industrial activities.4,5 Therefore residual dissolved organic carbon (DOC) present in RO-treated wastewater may consist of anthropogenic organic compounds, in addition to residual organic matter originally present in drinking water and wastewater, or chemicals used during RO treatment or leached from RO membranes.6,7 Very few attempts to characterise this residual DOC have been reported to date,8,9 although a recent assessment of 375 chemicals in recycled water suggested that only ~2–5% of DOC in the RO treated water could be attributed to regularly detected (>25% detection) anthropogenic chemicals.10 In this work we present the results of a characterisation study of recycled water collected from an Advanced Water Recycling Plant (AWRP) located in Perth (WA) after both RO and UV treatment over four days. The water samples were extracted using mixed bed solid-phase extraction cartridges and then characterised by liquid chromatography-high resolution mass spectrometry.
While almost 400 chemicals were screened previously in RO treated wastewater from a Perth wastewater treatment plant,5 particular emphasis in this work was given to polar chemicals amendable by LC-MS. The target screening in this study assessed the occurrence of 291 chemicals including pharmaceuticals (88 compounds), pharmaceutical-metabolites (27 compounds), illicit drugs and metabolites (14 compounds), pesticides (79 compounds), pesticide-metabolites (51 compounds), biocides and metabolites (11 compounds), artificial sweeteners (6 compounds), personal care products (3 compounds), corrosion inhibitor and metabolites (5 compounds), industrial chemicals (5 compounds) and miscellaneous (2 compounds). Chemicals were selected based on 1) prior knowledge of their occurrence in wastewater inflow and outflow; 2) existing studies of recycled water and surface waters from previous surveys and the literature.4,5,10,11 A snapshot of the chemicals and their transformation products and metabolites assessed in the target screening analysis is given in Fig. 1 and the full list of the chemicals is reported in Table S1 available in the ESI.† Eighty five percent (248 of 291) of the target compounds were analysed in the Perth AWRP for the first time in this study. The contribution of detected chemicals to the residual dissolved organic carbon (DOC) measured in UV treated water was also assessed. For a screening health risk assessment, risk quotients (RQ) were calculated by comparing median and maximum concentrations of chemicals measured in UV treated water with the corresponding health values.
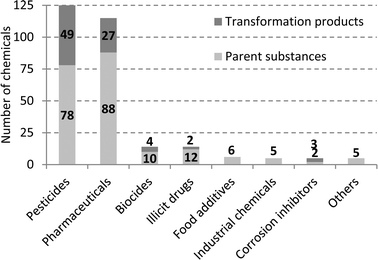 |
| Fig. 1 Parent compounds, transformation products and metabolites assessed in the target screening analysis. | |
2. Experimental
2.1 Sampling
Samples were collected on four days (16/01/12 to 19/01/12), from an AWRP in Perth, Western Australia. Details of the AWRP have been previously published,11–13 but briefly, the AWRP receives secondary wastewater (WW) from Beenyup WWTP and produces high purity recycled water that is then injected into a deep drinking water aquifer. Beenyup WWTP receives predominantly urban residential wastewater, and the raw WW is screened to remove large material, before grit removal and primary sedimentation. The primary treated WW then undergoes conventional activated sludge treatment with biological nutrient removal before clarification. Most secondary WW from Beenyup WWTP is discharged into the Indian Ocean, while a small portion (7 ML d−1) is fed into the AWRP. Treatment at the AWRP consists of chloramination to minimise biofouling on membranes, ultrafiltration, reverse osmosis, and UV disinfection. A caustic dosing between the UV reactors is also present to adjust the pH to neutral conditions before the product water is degassed, stored and reinjected into the groundwater. After UF/RO/UV treatment, about 4.5 ML d−1 are reinjected into the groundwater aquifer, while the RO reject (about 2.5 ML d−1) is sent back to the head of the WWTP. A schematic of the treatment train at Beenyup WWTP-AWRP including sampling points is shown in Fig. 2.
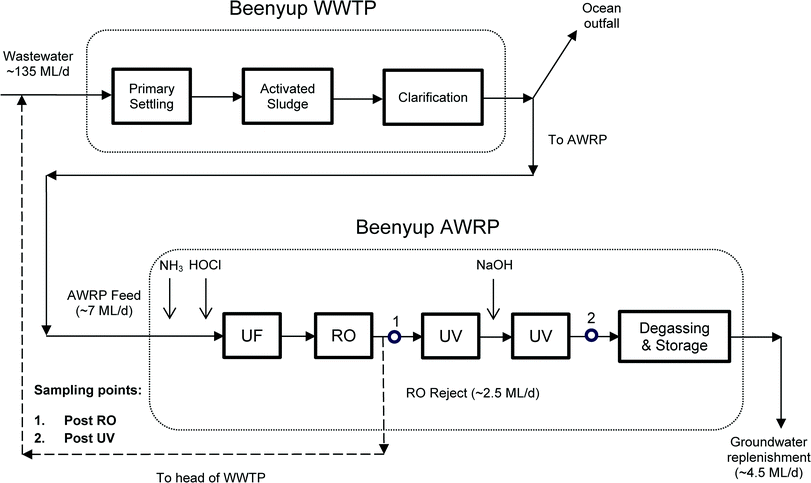 |
| Fig. 2 Schematic of Beenyup Wastewater Treatment Plant (WWTP) and Beenyup Advanced Water Recycling Plant (AWRP). Sampling points (i.e. 1–2) are also indicated. UF: ultrafiltration; RO: reverse osmosis; UV: UV disinfection; ML/d: megalitres per day. | |
Grab samples were collected directly after RO and UV treatment (i.e. after caustic dosing, see Fig. 2) in 2 L amber glass bottles, previously annealed at 550 °C overnight to ensure thermal degradation of and residual organic material. Bottles were also rinsed with the sample prior to sample collection. Sample were chilled with ice packs during transport to the CWQRC laboratory, and then stored at 4 °C until extraction. Prior to processing though solid-phase extraction (SPE), all samples were re-equilibrated to room temperature and then filtered through 0.45 μm Microfiberglass Duo-Fine® Filter cartridges (PALL Life Sciences, East Hills, USA) pre-conditioned with 10 L of ultrapure water. To avoid cross contamination, a single filter cartridge was dedicated to each type of water processed. Quality control samples consisted of post RO water and laboratory ultrapure water, fortified with different concentrations of standards and surrogate standards. Laboratory blanks were also processed along with the batch of samples and analysed for quality control purpose.
2.2 Sample extraction
Because of the low concentrations expected in the post RO and post UV water samples, solid-phase extraction (SPE) as described by Kern et al.14 was used to concentrate the analytes from the samples. Briefly, the pH of each sample (2 L) was adjusted to 6.5–6.7 by adding 1 mL of ammonium acetate buffer (1 mol L−1) and formic acid or ammonia solutions as required. For accurate quantification using LC-MS analysis, 100 ng of a surrogate standard mix containing 113 isotopically labelled substances were spiked to each sample. The layered ‘mixed bed’ cartridges for SPE consisted of 200 mg of OASIS HLB material (30 micro-M; Waters AG, USA) and 350 mg of mixed phase consisting of 100 mg Strata-X-AW (Phenomenex, USA), 100 mg Strata-X-CW (Phenomenex, USA) and 150 mg Isolute ENV+ (Separtis GmbH, Germany). An automated Aspec XLi extractor (Gilson, USA) was used for conditioning and elution of the cartridges. For conditioning, 5 mL of methanol and 10 mL of ultrapure water were dispensed at 2 mL min−1. After conditioning, samples were loaded onto the SPE cartridges using two 8-channel off-line peristaltic pumps (Gilson) at a flow rate of 5 mL min−1. Prior to elution, cartridges were completely dried using a vacuum manifold. The elution of the analytes from the SPE stationary phase was achieved by applying a basic solution (8 mL of ethylacetate/methanol containing 0.5% ammonia hydroxide (v/v)), followed by an acidic solution (4 mL of ethylacetate/methanol containing 1.7% formic acid (v/v)) dispensed at 2 mL min−1. The eluates were concentrated to about 100 μL using a dry block heater (30 °C) fitted with nitrogen blowdown (Ratek 30D, Australia), before being rediluted to 1 mL using ultra pure water. Finally, the extracts were filtered directly into a 2 mL brown glass vial using a syringe fitted with a 0.45 μm regenerated cellulose membrane filter (Infochroma AG, Switzerland). Samples extracts chilled with ice packs were shipped using an international express delivery service to EAWAG laboratories in Dübendorf (Switzerland) for analysis.
2.3 Chromatographic separation
For the reversed phase chromatographic separation, an aliquot of the extract (20 μL) was injected onto a XBridge C18 column (Waters USA, 2.1 × 50 mm, 3.5 μm particle diameter) using a guard column (2.1 × 10 mm) of the same stationary phase. The eluent consisted of nanopure water (eluent A) and methanol (eluent B), both containing 0.1% (v/v) formic acid. The LC gradient used for the separation was as follows: 0–4 min, eluent B was increased from 10–50%; 4–17 min, eluent B was increased from 50–95%, then continued at 95% for 8 minutes. Prior to the next injection, the column was re-equilibrated with 90% eluent A and 10% eluent B for 5 min. The eluent flow rate was 0.2 mL min−1 at a temperature of 30 °C.
2.4 Detection and quantification using high resolution mass spectrometry (HRMS)
Analytes were detected using a high resolution mass spectrometer (Q Exactive; Thermo Fisher Scientific Corporation, USA). Ionisation of analytes was achieved using electrospray ionisation (ESI†) operated in both positive (+eV) and negative (−eV) modes. The ESI† and HRMS settings are reported in Table S2, available in the ESI.† A screening analysis was conducted where the selected target analytes were recorded using Q Exactive mass spectrometer full-scan spectra from 100–1000 m/z with a mass resolution (R) of 140
000 (@ 200 m/z) in positive and negative ionisation mode. For confirmation, all target analytes were fragmented in the HCD collision cell (high energy collision dissociation) using a data-dependent MS2 fragmentation approach. The top 5 MS2 spectra were measured in the Orbitrap mass analyser with a resolution of 17
500, normalized collision energies ranged from 20–100%.
2.5 Data processing and quantification
A target screening was conducted on the Q Exactive raw data files using the software package enviMass 1.2.15 Peak lists for each sample were generated from raw data files using the freely available peak picking software Formulator (rev3, Thermo Fisher Scientific). Parameters for both the enviMass 1.2 and the Formulator software are listed in Table S3–S4 available in the ESI.† Peak lists were then loaded into the enviMass1.2 software for a qualitative target screening of 291 target substances. Positive detects were manually reprocessed and quantified using the Xcalibur 2.2 QuanBrowser Software (Thermo Fisher Scientific). Quantification was performed with seven extracted standard samples containing all spiked target compounds with concentrations of 1, 5, 10, 25, 50, 100 ng L−1 and a blind sample (extracted nanopure water with spiked internal standards mix).
For quality control, the relative SPE recoveries of the method were determined. One matrix sample constituted of post RO water was split in two equal portions. The first portion was spiked with 100 ng L−1 of standard mix and labelled surrogates while the second portion was treated as a blank and spiked with the labelled surrogates only, both samples were treated exactly as the other samples in the batch. Relative deviations of recoveries within ±30% were accepted (see Table S5 available in the ESI†).
All detected substances were confirmed using the data-dependend MS2 spectra which were compared against single substance injection MS2 spectra acquired in house with varying collision energies.
3. Results and discussion
3.1 Compounds detected in RO treated water
The concentrations of detected chemicals in RO treated water are reported in Table 1. Only 13 of the 291 chemicals targeted (i.e. ~4.5%) were detected in RO water, although these chemicals were frequently detected in either 3 or 4 of the 4 sampling events. The chemicals detected included two corrosion inhibitors (4+5-methyl-1H-benzotriazole and benzotriazole), three pesticides (metolachlor, propiconazol, prosulfocarb), three pharmaceuticals (lamotrigin, metformin, tramadol), 1 personal care product metabolite (galaxalidone), three artificial sweeteners (acesulfame, saccharin and sucralose) and 1 industrial chemical (triethyl phosphate). Most chemicals were detected at a concentration less than 50 ng L−1. However, the corrosion inhibitors chemicals benzotriazole and 4+5-methyl-1H-benzotriazole, the pharmaceutical metformin and the industrial chemical triethyl phosphate were detected at much higher concentrations, between 65 and 1300 ng L−1.
Table 1 Concentration range (min–max) and median concentration (ng L−1) of detected target compounds in post RO and post UV water samples collected from Beenyup AWRP on four different days (16/01/12–19/01/12). The average (%) UV removal with the relative standard deviation is also reported
Chemicals |
Post RO samples |
Post UV samples |
Concentration range min–max |
Median |
Concentration range min–max |
Median |
Average (%) UV removal (±RSD) |
LOQ.
Tentatively quantified; N/A: not available.
Outlier.
|
4+5-Methyl-1H-benzotriazole |
750–900 |
775 |
350–400 |
375 |
53 ± 6 |
Benzotriazole |
750–1300 |
800 |
375–550 |
412 |
51 ± 5 |
Galaxalidone |
15–52.5 |
29 |
5–30 |
14 |
37 ± 48 |
Lamotrigin |
5–5 |
5 |
2.5–5 |
2.5 |
N/A |
Metolachlor |
<1a–7.5 |
3.75 |
0.5b–5 |
2.5 |
N/A |
Metformin |
65–105 |
99 |
87.5–110 |
95 |
N/A |
Propiconazole |
5–8 |
7.5 |
2.5–7.5 |
6.25 |
N/A |
Prosulfocarb |
2.5b–5 |
5 |
<5a–5 |
5 |
N/A |
Tramadol |
0.5b–475c |
0.5b |
0.5b–100c |
N/A |
N/A |
Acesulfame |
25–35 |
26 |
<1a–2.5 |
N/A |
95 ± 2 |
Saccharin |
<1a–2.5 |
2.5 |
<1a–5 |
5 |
N/A |
Sucralose |
2.5–10 |
10 |
2.5–10 |
8.75 |
N/A |
Triethyl phosphate |
200–200 |
200 |
100–100 |
100 |
50 ± N/A |
Benzotriazole and its derivatives, including 4+5-methyl-1H-benzotriazole are high volume production chemicals, with an estimated worldwide production of benzotriazoles in excess of 9000 tons per year.16,17 They are commonly used in paints and polymers as UV absorbers, detergents, antifreeze, brake fluids and in aircraft de-icing fluids as corrosion inhibitors18 and they are extensively found in WW and the environment.19 They have been found to be acutely toxic to specific species,20 but their chronic toxicity is not well studied. Benzotriazole and 4+5-methyl-1H-benzotriazole were consistently detected in relatively high concentration ranges in RO treated water, and this is expected given the μg L−1 concentrations previously detected in Beenyup secondary treated WW,12 the relatively low MW (<150 Da) and the high water solubility and mobility (log
Kow = 1.23 for benzotriazole and log
Kow = 1.89 for 4+5-methyl-1H-benzotriazole, see Table S5 available in the ESI†). These results are also consistent with our previous findings, which showed benzotriazole and 4+5-methyl-1H-benzotriazole concentrations in RO treated water averaging 974 (±28) ng L−1 and 416 (±34) ng L−1, respectively.12
Galaxolidone, is a metabolite of galaxolide, a polycyclic musk widely used as a fragrance in personal care and consumer products including cosmetics, cleaning agents, detergents, air fresheners and perfumes.21 Galaxolidone results from the degradation of the parent compound galaxolide during biological activated sludge.22,23 Given their high log
Kow (5.9 and 5.3 respectively), both galaxolide and galaxolidone can concentrate in blood, fat24 and breast milk.25 Synthetic musks can affect androgen and progesterone receptors and also stimulate estrogenic receptors in humans.26 Polycyclic musks have been reported in water bodies and biota previously.27 The median concentration of galaxolidone in RO treated water was 29 ng L−1. However, galaxalidone was also detected in the CWQRC laboratory blanks at 10 ng L−1 (data not shown). This contamination could have resulted from an accidental exposure of the laboratory blank to the chemical at the time of sample collection or during the sample preparation process. Galaxolide and its metabolite galaxalidone will preferentially absorb onto plastic and glass (i.e. SPE equipment) and therefore are prone to cross-contamination. Despite this cross-contamination issue, it is still likely that low ng L−1 concentration of galaxolidone are present in RO treated water. The parent compound galaxolide was previously measured in wastewater and recycled samples from Beenyup WWTP and AWRP.11 Even though galaxolidone is neutral, based on the scheme proposed by Bellona et al.7 the physical-chemical properties of this chemical (MW > Molecular weight cut off (MWCO) of the membrane, approximately 150–200 Da, and log
Kow > 2), a moderate to high rejection is expected during RO treatment. Given the high volume of usage and high concentration of galaxolide in WW11 and the high hydrophobicity of galaxolidone, it may have accumulated on the membranes and eventually achieved breakthrough from diffusion phenomena.7
The median concentration of the industrial chemical triethyl phosphate in RO treated water was 200 ng L−1. Triethyl phosphate is a common flame retardant, a polymer resin modifier, a plasticizer (e.g. for unsaturated polyesters) and an intermediate in the manufacture of pesticides and other chemicals.28–30 It is used as an industrial catalyst (in acetic anhydride synthesis) and as a solvent (e.g. cellulose acetate), a stabilizer for peroxides, and a strength agent for rubber and plastics including vinyl polymers and unsaturated polyesters.28–30 Previously, we have detected a range of phosphate chemicals including triethyl phosphate, tris(chloropropyl) phosphate, tris(dichloropropyl) phosphate in wastewater samples from Beenyup WWTP. Furthermore, while all phosphate chemicals were below detection in samples collected post-RO treatment (LOD = 100 ng L−1), they were all detected in the RO reject water.11 Further research is needed to better understand the occurrence of triethyl phosphate in wastewater and assess the rejection of this class of chemicals during UF/RO treatment. However, given the small molecular weight, the high pKa and the low log
Kow (see Table S5†) a poor rejection is expected for this compound.7
Out of 79 pesticides, 51 pesticides metabolites, 9 biocides and 2 biocides metabolites targeted, only 2 pesticides (metolachlor and prosulfocarb) and 1 biocide (propiconazole) were detected at very low concentrations in RO treated water. Interestingly, the pesticide metolachlor was also detected in our previous work,10 at similar concentrations, possibly indicating breakthrough during RO treatment. However, in this study metolachlor was detected in 100% of the samples tested (4 RO treated samples and 4 UV treated samples, see Table 1) compared to only 3% of the samples (1 sample out of 33 analysed) tested previously.10 The high frequency in the detection observed for metolachlor in this work may be due to the much lower LOD (1 ng L−1) achieved in this study compared to the LOD (60 ng L−1) achieved in the previous study. Propiconazole, the biocide detected in this work, was not detected previously,10 again possibly because the LOD (5 ng L−1) achieved in this study was much lower than the LOD (100 ng L−1) achieved in the previous study. Prosulfocarb was not analysed previously and therefore a comparison is not possible. All three pesticides have MW that is greater than the MWCO of the RO membranes and also possess log
Kow > 2. In this scenario, good removal is expected, although membrane breakthrough could be caused by partitioning/diffusion within the membrane.7
Only 3 pharmaceuticals of the 88 pharmaceuticals and 27 pharmaceutical metabolites tested were detected in RO treated water. Lamotrigine is an anticonvulsant drug used in the treatment of epilepsy and bipolar disorder.31 It is also used off-label as an adjunct in treating depression. Tramadol is used similarly to codeine and it is a synthetic analgesic used to treat moderate to moderately-severe pain. The drug has a wide range of applications, including treatment of rheumatoid arthritis, restless legs syndrome and fibromyalgia.32 Metformin is very commonly used for treatment of type 2 diabetes in obese and overweight people and it is listed as one (of only two) oral antidiabetics in the World Health Organization Model List of Essential Medicines.33 All 3 pharmaceuticals are registered for use and commonly sold in Australia.34 Concentration of lamotrigine and tramadol in RO treated water were below 5 ng L−1, with the exception of tramadol, which was measured at 475 ng L−1 in one sample (19/01/2012). The reason for such high concentration post RO treatment is not known. However, given that contamination from this compound (100 ng L−1) was also seen in one laboratory blank, this single high detection level should be treated as an outlier. In contrast the median concentration of metformin over all RO treated water samples was 99 ng L−1. Metformin, is commonly found in water bodies due to its high volume usage.35 Moreover, given its relatively low molecular weight and high solubility (see Table S5†), RO rejection is expected to be relatively poor. All 3 pharmaceuticals have been previously tested in Beenyup WWTP and Beenyup AWRP but were not detected in RO treated water possibly due to higher LOD.11
The 3 artificial sweeteners detected in RO treated water were acesulfame, sucralose and saccharin. While the presence of artificial sweeteners, a common constituent of low calorie food and beverages, in the aquatic environment has been reported in previous studies overseas,36–43 little has been reported regarding their presence within Australian waters.11 Recent studies have shown that artificial sweeteners are quite stable and persistent in the environment, and are excreted predominantly unchanged as waste from the body.36,39,40 Sucralose in particular, is resistant towards biodegradation, and as a result is persistent in WWTP.36,37,43 To the best of our knowledge, little has been reported regarding the behavior of artificial sweeteners during RO treatment. Acesulfame (MW = 162 Da) and saccharin (MW = 183 Da) both have molecular weights close to the MWCO of the RO membrane. Moreover both have high water solubility,42,44,45 meaning they are unlikely to adsorb on membranes and therefore poor rejection is expected7 (see Table S5†). Furthermore, the presence of μg L−1 concentrations in secondary WW feed to Beenyup AWRP may also play an important role in the detection of these sweeteners post RO treatment, as high concentrations in secondary WW have been linked to detection in RO treated water, even when RO rejection is high.10 Artificial sweeteners represent an ideal marker for wastewater contamination and the study of their behavior during RO treatment could significantly aid wastewater recycling and future management of groundwater replenishment.
3.2 Compounds detected in UV treated water
At Beenyup AWRP, the last treatment barrier is UV for pathogen inactivation. This barrier employs ITT Wedeco units (low pressure lamps, UV-C at 254 nm, 4 UV units in series, dose of up to 50 mJ cm−2 for each unit). Analysis of samples post-UV treatment showed that the concentration of some UV degradable compounds was reduced. Table 1 presents the concentration of chemicals detected in UV treated water as well the observed average removal after the UV treatment, calculated using the percentage difference in concentration between RO treated water and UV treated water for matched samples.
For both benzotriazole and 4+5-methyl-1H-benzotriazole the UV treatment led to average removal of about 50% of the initial concentrations. This is in agreement with previous research showing benzotriazoles are prone to degradation by UV light (direct photolysis); benzotriazole and its derivatives are known UV absorbers,46,47 so degradation and reactivity of this class of chemicals was expected to be significant. For galaxolidone, a moderate but highly variable removal was achieved (average UV removal = 37 ± 48%). A significant and consistent reduction of the concentration of the artificial sweeteners acesulfame (average UV removal: 95 ± 2%) was also observed. The kinetic and the mechanism of degradation of this compound has previously been described in full,48 confirming the effectiveness of UV treatment to reduce the concentration of this compound in receiving waters. For triethyl phosphate the median UV removal was 50%. For the remaining compounds detected in RO treated water, it was not always clear whether UV treatment reduced concentrations in the final product water as most concentrations were low and near the LOQ of the compound or in some case, the concentration detected post UV was higher than the concentration detected post RO.
Tertiary treatments such as UV for virus inactivation, as well as advanced oxidation processes including use of strong oxidants such as ozone, H2O2 + UV and combination of them, usually result in the incomplete mineralisation of micropollutants, and the formation of mixtures of transformation by-products.49–51 The chemical structures, but more importantly the toxicological properties, of the transformation by-products arising from incomplete oxidation of micropollutants remains unknown for a wide range of chemicals although research in this area has been rapidly developing in the last decade.49–52 Nowadays, there is general consensus that whenever an oxidation process is applied to polish the final water, transformation by-products should be also assessed through non-target analysis and included in the risk assessment where possible. Further research into the integrated use of target/non-target chemical screening and bioassays as a monitoring tool for water recycling is recommended.
3.3 Contribution of anthropogenic chemicals to the dissolved organic carbon
Previous research characterized RO water in Perth, Western Australia for 375 anthropogenic chemicals, with 108 chemicals detected on at least one occasion, and 30 chemicals detected in more than 25% of all samples.5,10 However, assessment of the contribution of these detected chemicals to the DOC measured in RO treated water was only able to attribute 2.5 to 5% of the DOC to anthropogenic chemicals.10 One of the objectives of this present work was to assess whether analysis of an extended list of polar organic chemicals could help to account for the remaining DOC. In this study, the RO treated water was analysed for 291 chemicals, of which 248 chemicals have not been analysed previously at Beenyup AWRP. Out of these 291 chemicals, only 13 compounds (~4.5%) were detected. The contribution of anthropogenic chemicals to the residual DOC in post UV treated water was estimated using the same methodology described in Linge et al., in which DOC contribution is calculated using the percentage carbon in each detected molecule.10 Overall, the detection of these 13 compounds in the RO effluent could not account for the residual DOC in RO water, with the detected chemicals contributing between 0.6 μg L−1 (median value) to 1.3 μg L−1 (maximum value) of DOC. The DOC measured at Beenyup AWRP plant over the 4 days of sampling (16/01/12–19/01/12) averaged 58 μg L−1 and therefore, the total contribution of DOC from these anthropogenic chemicals remains very small (1.0–2.3%).
3.4 Screening health risk assessment
A screening health risk assessment was conducted using the concept of the risk quotient (RQ), which is calculated as the ratio between the reported concentration of each chemical and the appropriate health values.5,10 Median and maximum concentrations of chemicals detected in post UV treated water were used to generate median and maximum RQs (Table 2). A risk quotient below one implies no health impact is expected. Health values for benzotriazole, metolachlor and metformin were taken from the Australian Guidelines for Water Recycling,53 while a health value for propiconazole was taken from the Australian Drinking Water Guidelines.54 The health value for tolyltriazoles (4+5-methyl-1H-benzotriazole) is the value used for recycled water at Beenyup AWRP.55 No Australian derived water guidelines exist for the other chemicals detected in this study and therefore other approaches were used to determine health values. For the metabolite galaxolidone, the AGWR guideline for the parent compound galaxolide (1750 μg L−1) was used, with the addition of an extra safety factor of ten.53 For triethyl phosphate, a health value of 1000 μg L−1 was used, as it belongs to the same class of phosphate flame retardants, including tri(dichlorisopropyl) phosphate, triphenyl phosphate; tris(2 chloroethyl)phosphate.53 For the pharmaceuticals lamotrigine and tramadol, health values were calculated from the lowest daily therapeutic dose (LDTD)56 using the approach outlined in the Australian Guidelines for Water Recycling:53 | Health value (μg L−1) = (LDTD (mg per day) × P × 103)/(SF × V (L per day)) | (1) |
where P = proportion of LDTD estimated to come from drinking water (100%); 103 = unit conversion mg L−1 to μg L−1; SF: safety factor (1000 for most pharmaceuticals); V = volume of water drunk (2 L per day). Health values for the artificial sweeteners, acesulfame, saccharine and sucralose,44 and the pesticide prosulfocarb57 were all derived from acceptable daily intake values (ADI, expressed as mg kg−1 body weight, assuming body weight = 70 kg) as outlined in the Australian Guidelines for Water Recycling.53
Table 2 Screening health risk assessment for UV treated water reporting median and maximum risk quotients (RQmed and RQmax)
Chemicals |
Health value (μg L−1) |
RQmed |
RQmax |
Ref. |
Galaxolidone, a metabolite of galaxolide, currently does not have a guideline value. The drinking water guideline for galaxolide (1750 μg L−1) was used instead with an extra safety factor of ten.
Drinking water guideline calculated from the lowest daily therapeutic dose (LDTD).
Drinking water guideline calculated from the acceptable daily intake (ADI).
Drinking water guideline derived from similar phosphate flame retardants.
|
4+5-Methyl-1H-benzotriazole |
20 |
0.019 |
0.020 |
55
|
Benzotriazole |
20 |
0.021 |
0.028 |
53
|
Galaxolidone |
175a |
0.000008 |
0.00002 |
53
|
Lamotrigine |
12.5b |
0.0002 |
0.0004 |
56
|
Metolachlor |
300 |
0.000008 |
0.00002 |
53
|
Metformin |
250 |
0.0004 |
0.0004 |
53
|
Propiconazole |
100 |
0.00006 |
0.000075 |
54
|
Prosulfocarb |
0.175c |
0.028 |
0.028 |
57
|
Tramadol |
200b |
N/A |
0.0000125 |
56
|
Acesulfame |
315c |
N/A |
0.0000079 |
44
|
Saccharin |
133c |
0.000037 |
0.000037 |
44
|
Sucralose |
525c |
0.000016 |
0.000019 |
44
|
Triethyl phosphate |
1000d |
0.0001 |
0.0001 |
53
|
For all chemicals both RQmed and RQmax were between 2 and 6 orders of magnitude below 1 implying a high degree of safety associated with human consumption of recycled water.
4. Conclusions
The target screening conducted in RO and UV treated water samples from Beenyup AWRP has shown the presence of small (MW < 200 Da), hydrophilic species such as corrosion inhibitors (i.e. benzotriazole and 4+5-methyl-1H-benzotriazole), pharmaceuticals (i.e. metformin), artificial sweeteners (i.e. acesulfame and saccharin) and industrial chemicals (i.e. triethyl phosphate). These chemicals were consistently found in all samples and could potentially be used as treatment performance indicators in future studies. Very low concentrations (ng L−1) of pesticides (metolachlor, propiconazole and prosulfocarb), along with other pharmaceuticals (lamotrigine and tramadol) were also detected. The break-through during RO treatment of some of these relatively large (MW > 250 Da) and hydrophobic (log
Kow > 2) chemicals could be due to diffusion/partitioning within the membrane. The UV treatment installed at Beenyup AWRP helped to reduced UV degradable compounds such as the corrosion inhibitors (>50% removal), the flame retardant triethyl phosphate (~50% removal) and the artificial sweetener acesulfame (~95% removal). Overall, the contribution of the detected anthropogenic chemicals to the DOC measured in post UV treated water was found to be minimal (1.0–2.3%). The target screening analysis also show that a number of anthropogenic chemicals (i.e. 278 out of 291 compounds, >95.5%) such as pesticides, biocides, industrial chemicals, pharmaceuticals and metabolites were not detectable in RO and UV treated water. A screening heath risk assessment showed that RQ were generally 2 to 6 orders of magnitude below 1, implying a high degree of safety associated with human consumption of recycled water. Overall this chemical screening provides further evidence of the overall safety of the use of recycled wastewater treated by RO and UV as a potable water source, and it has confirmed that anthropogenic chemicals constitute a relatively small percentage of DOC in RO treated WW. However, the study also highlights the limitations of the traditional approach for assessing chemical safety, focused on end-product compliance testing for all potential chemicals. This approach is time consuming, expensive, and relies on the availability of appropriate health values for all chemicals tested. Given the time consuming nature of trace chemical analysis, further research into the use of bioassays as a monitoring tool for water recycling is recommended.11,58–61 Bioanalytical tools can screen for a wide range of contaminants and transformation products, based on biological effect, rather than monitoring specific chemicals, and may provide an efficient high-throughput tool broad screen assessment of water quality or hazard identification, and risk characterisation.
Acknowledgements
This work was undertaken in alignment with ARC Linkage Project 0989326 “Treating wastewater for potable reuse: removal of chemicals of concern using advanced oxidation processes” (Partner Organisations: Water Corporation of Western Australia, GHD Pty Ltd and WaterRA). We thank all project partners, especially the staff at the Water Corporation of Western Australia (B. Edwards, M. Handyside, P. Blair and S. Hamilton) for coordinating the site visits and sample collection. We also acknowledge the contribution of two anonymous reviewers to revision and improvement of the manuscript.
References
-
Water Forever: Towards Climate Resilience. ISBN 1 74043 521 4, Water Corporation of Western Australia, Perth, Western Australia, 2009 Search PubMed.
- A. J. Watkinson, E. J. Murby and S. D. Costanzo, Water Res., 2007, 41, 4164–4176 CrossRef CAS PubMed.
- J. E. Drewes, M. Reinhard and P. Fox, Water Res., 2003, 37, 3612–3621 CrossRef CAS.
-
J. E. Drewes, D. Sedlak, S. Snyder and E. Dickenson, Development of Indicators and Surrogates for Chemical Contaminant Removal during Wastewater Treatment and Reclamation WRF03-014, WateReuse Foundation, Alexandria, 2008 Search PubMed.
-
P. Van Buynder, R. Lugg, C. Rodriguez, M. Bromley, J. Filmer, P. Blair, M. Handyside, S. Higginson, N. Turner, O. Lord, P. Taylor, K. Courtney, C. Newby, A. Heitz, K. Linge, J. Blythe, F. Busetti and S. Toze, Premier's Collaborative Research Program (2005–2008). Characterising Treated Wastewater For Drinking Purposes Following Reverse Osmosis Treatment. Technical Report, Department of Health, Western Australia, 2009 Search PubMed.
- J. E. Drewes and P. Fox, Water Environ. Res., 2000, 72, 353–362 CrossRef CAS.
- C. Bellona, J. E. Drewes, P. Xu and G. Amy, Water Res., 2004, 38, 2795–2809 CrossRef CAS PubMed.
- B. B. Levine, K. Madireddi, V. Lazarova, M. K. Stenstrom and M. Suffet, Water Sci. Technol., 1999, 40, 293–301 CrossRef CAS.
- M. Reinhard, N. L. Goodman, P. L. McCarty and D. G. Argo, J. - Am. Water Works Assoc., 1986, 78, 163–174 CAS.
- K. L. Linge, P. Blair, F. Busetti, C. Rodriguez and A. Heitz, J. Water Supply: Res. Technol.--AQUA, 2012, 61, 494–505 CrossRef CAS.
- J. Y. M. Tang, F. Busetti, J. W. A. Charrois and B. I. Escher, Water Res., 2014, 60, 289–299 CrossRef CAS PubMed.
- C. Loi, F. Busetti, K. L. Linge and C. Joll, J. Chromatogr. A, 2013, 1299, 48–57 CrossRef CAS PubMed.
- S. Allard, J. W. A. Charrois, C. A. Joll and A. Heitz, J. Chromatogr. A, 2012, 1238, 15–21 CrossRef CAS PubMed.
- S. Kern, K. Fenner, H. P. Singer, R. P. Schwarzenbach and J. Hollender, Environ. Sci. Technol., 2009, 43, 7039–7046 CrossRef CAS.
-
M. Loos, M. Ruff and H. Singer, enviMass version 1.0 target screening software, Eawag: Swiss Federal Institute of Aquatic Science and Technology, Dübendorf, Switzerland, 2011 Search PubMed.
- S. Weiss and T. Reemtsma, Anal. Chem., 2005, 77, 7415–7420 CrossRef CAS PubMed.
- D. S. Hart, L. C. Davis, L. E. Erickson and T. M. Callender, Microchem. J., 2004, 77, 9–17 CrossRef CAS PubMed.
- S. Weiss, J. Jakons and T. Reemtsma, Environ. Sci. Technol., 2006, 40, 7193–7199 CrossRef CAS.
- T. Reemtsma, U. Miehe, U. Duennbier and M. Jekel, Water Res., 2010, 44, 596–604 CrossRef CAS PubMed.
-
X. Wu, N. Chou, D. Lupher and L. Davis, Proceedings of the 1998 Conference on Hazardous Waste Research, Snowbird, Utah, 1998 Search PubMed.
- D. Fernandes, G. Dimastrogiovanni, M. Blázquez and C. Porte, Environ. Pollut., 2013, 174, 214–221 CrossRef CAS PubMed.
- J. L. Reiner and K. Kannan, Chemosphere, 2006, 62, 867–873 CrossRef CAS PubMed.
- K. Bester, J. Environ. Monit., 2005, 7, 43–51 RSC.
- G. G. Rimkus and M. Wolf, Chemosphere, 1996, 33, 2033–2043 CrossRef CAS.
- K. Kannan, J. L. Reiner, S. H. Yun, E. E. Perrotta, L. Tao, B. Johnson-Restrepo and B. D. Rodan, Chemosphere, 2005, 61, 693–700 CrossRef CAS PubMed.
-
A. Kortenkamp, O. Martin, M. Faust, R. Evans, R. McKinlay, F. Orton and E. Rosivatz, State of the art assessment of endocrine disrupters Final Report, European Commission, 2011 Search PubMed.
- H. Ruedel, W. Boehmer and C. Schroeter-Kermani, J. Environ. Monit., 2006, 8, 812–823 RSC.
- X.-W. Wang, J.-F. Liu and Y.-G. Yin, J. Chromatogr. A, 2011, 1218, 6705–6711 CrossRef CAS PubMed.
- I. van der Veen and J. de Boer, Chemosphere, 2012, 88, 1119–1153 CrossRef CAS PubMed.
- H. Takigami, G. Suzuki, Y. Hirai and S.-I. Sakai, Chemosphere, 2009, 76, 270–277 CrossRef CAS PubMed.
- L. Barbosa, M. Berk and M. Vorster, J. Clin. Psychiatry, 2003, 64, 403–407 CrossRef CAS.
- M. O. Rojas-Corrales, E. Berrocoso, J. Gibert-Rahola and J. A. Mico, J. Clin. Psychiatry, 2004, 18, 404–411 CAS.
-
WHO Model List of Essential Medicines, 18th edn, World Health Organisation, Geneva, Switzerland, 2013, http://apps.who.int/iris/bitstream/10665/93142/1/EML_18_eng.pdf?ua=1 Search PubMed.
-
Therapeutic Goods Administration, http://www.tga.gov.au/, Australian Government, Department of Health, Canberra, 2014 Search PubMed.
- M. Scheurer, A. Michel, H. J. Brauch, W. Ruck and F. Sacher, Water Res., 2012, 46, 4790–4802 CrossRef CAS PubMed.
- I. J. Buerge, H. R. Buser, M. Kahle, M. D. Muller and T. Poiger, Environ. Sci. Technol., 2009, 43, 4381–4385 CrossRef CAS.
- I. Ferrer and E. M. Thurman, J. Chromatogr. A, 2010, 1217, 4127–4134 CrossRef CAS PubMed.
- R. Loos, B. M. Gawlik, K. Boettcher, G. Locoro, S. Contini and G. Bidoglio, J. Chromatogr. A, 2009, 1216, 1126–1131 CrossRef CAS PubMed.
- N. Lubick, Environ. Sci. Technol., 2008, 42, 3125 CrossRef CAS.
- N. Lubick, Environ. Sci. Technol., 2009, 43, 4220–4220 CrossRef CAS.
- R. N. Mead, J. B. Morgan, G. B. Avery Jr, R. J. Kieber, A. M. Kirk, S. A. Skrabal and J. D. Willey, Mar. Chem., 2009, 116, 13–17 CrossRef CAS PubMed.
- M. Scheurer, H.-J. Brauch and F. Lange, Anal. Bioanal. Chem., 2009, 394, 1585–1594 CrossRef CAS PubMed.
- M. Scheurer, F. R. Storck, H. J. Brauch and F. T. Lange, Water Res., 2010, 44, 3573–3584 CrossRef CAS PubMed.
- F. T. Lange, M. Scheurer and H.-J. Brauch, Anal. Bioanal. Chem., 2012, 403, 2503–2518 CrossRef CAS PubMed.
- M. Scheurer, M. Godejohann, A. Wick, O. Happel, T. A. Ternes, H.-J. Brauch, W. K. L. Ruck and F. T. Lange, Environ. Sci. Pollut. Res., 2012, 19, 1107–1118 CrossRef CAS PubMed.
- J. L. Gerlock, W. Tang, M. A. Dearth and T. J. Korniski, Polym. Degrad. Stab., 1995, 48, 121–130 CrossRef CAS.
- M. A. Dearth, T. J. Korniski and J. L. Gerlock, Polym. Degrad. Stab., 1995, 48, 111–120 CrossRef CAS.
- M. Scheurer, B. Schmutz, O. Happel, H. J. Brauch, R. Wülser and F. R. Storck, Sci. Total Environ., 2014, 481, 425–432 CrossRef CAS PubMed.
- M. M. Huber, T. A. Ternes and U. Von Gunten, Environ. Sci. Technol., 2004, 38, 5177–5186 CrossRef CAS.
- T. Haddad, E. Baginska and K. Kümmerer, Water Res., 2015, 72, 75–126, DOI:10.1016/j.watres.2014.12.042.
- S. D. Richardson and T. A. Ternes, Anal. Chem., 2014, 86, 2813–2848 CrossRef CAS PubMed.
- T. Kosjek, S. Perko, D. Žigon and E. Heath, J. Chromatogr., A, 2013, 1290, 62–72 CrossRef CAS PubMed.
-
Australian Guidelines for Water Recycling: Managing Health and Environmental Risks. Augmentation of Drinking Water Supplies, Environment Protection and Heritage Council, National Health and Medical Research Council, Natural Resource Management Ministerial Council, Canberra, 2008 Search PubMed.
-
Australian Drinking Water Guidelines 6, National Health and Medical Research Council, Natural Resource Management Ministerial Council, Canberra, 2011, https://www.clearwater.asn.au/user-data/resource-files/Aust_drinking_water_guidelines.pdf Search PubMed.
-
Memorandum of Understanding between the Department of Health and Water Corporation for Recycled Water, Water Corporation of Western Australia, Government of Western Australia Department of Health, Perth, Western Australia, 2010 Search PubMed.
-
Martindale, The Complete Drug Reference, ed. S. C. Sweetman, BPharm, FRPharmS, 36th edn, Pharmaceutical Press, London, UK, 2009 Search PubMed.
-
Conclusion regarding the peer review of the pesticide risk assessment of the active substance prosulfocarb, European Food Safety Authority, Parma, Italy, 2007 Search PubMed.
- F. D. L. Leusch, S. J. Khan, S. Laingam, E. Prochazka, S. Froscio, T. Trinh, H. F. Chapman and A. Humpage, Water Res., 2014, 49, 300–315 CrossRef CAS PubMed.
- B. I. Escher, M. Allinson, R. Altenburger, P. A. Bain, P. Balaguer, W. Busch, J. Crago, N. D. Denslow, E. Dopp, K. Hilscherova, A. R. Humpage, A. Kumar, M. Grimaldi, B. S. Jayasinghe, B. Jarosova, A. Jia, S. Makarov, K. A. Maruya, A. Medvedev, A. C. Mehinto, J. E. Mendez, A. Poulsen, E. Prochazka, J. Richard, A. Schifferli, D. Schlenk, S. Scholz, F. Shiraishi, S. Snyder, G. Su, J. Y. M. Tang, B. V. D. Burg, S. C. V. D. Linden, I. Werner, S. D. Westerheide, C. K. C. Wong, M. Yang, B. H. Y. Yeung, X. Zhang and F. D. L. Leusch, Environ. Sci. Technol., 2014, 48, 1940–1956 CrossRef CAS PubMed.
- M. Macova, S. Toze, L. Hodgers, J. F. Mueller, M. Bartkow and B. I. Escher, Water Res., 2011, 45, 4238–4247 CAS.
-
J. E. Drewes, J. D. C. Hemming, J. J. Schauer and W. C. Sonzogni, Removal of Endocrine Disrupting Compounds in Water Reclamation Processes, Water Environment Research Fundation, 2006 Search PubMed.
Footnote |
† Electronic supplementary information (ESI) available. See DOI: 10.1039/c4ew00104d |
|
This journal is © The Royal Society of Chemistry 2015 |
Click here to see how this site uses Cookies. View our privacy policy here.