Research highlights: advances and challenges in developing mainstream anammox treatment
First published on 21st August 2015
Energy-neutral and energy-positive wastewater treatment processes are highly desirable for both environmental and economic reasons. The conventional nitrification–denitrification process for nitrogen removal is energy and chemical intensive, and anammox-based technologies have emerged as an attractive alternative. Anammox bacteria convert ammonia and nitrite directly to nitrogen gas. Compared to conventional nitrification–denitrification, partial nitritation followed by anammox halves the amount of ammonia oxidized to nitrite and bypasses oxidation of nitrite altogether, reducing aeration requirements. Additionally, anammox bacteria are autotrophic, decoupling nitrogen removal from the removal of biological oxygen demand (BOD). This eliminates the need for the addition of organic carbon or recycle streams and makes it suitable for pairing with anaerobic wastewater treatment for energy recovery, which removes BOD but not nutrients. Anammox-based technologies are already implemented in more than 100 full-scale plants worldwide,1 with a variety of reactor configurations including suspended biomass, granular biomass, and moving bed biofilm reactors (MBBR) that utilize biofilm carriers. At present, however, this is limited to side-stream processes in municipal wastewater treatment (15–25% of the total nitrogen load)2 and industrial wastewater treatment. The application of anammox bacteria for mainstream municipal wastewater treatment is still challenging due to a high BOD/N ratio in the wastewater, strict temperature requirements, the slow growth rates of anammox bacteria, and competition from heterotrophic denitrifiers and nitrite oxidizing bacteria (NOB).3,4 In this article, we highlight recent studies about monitoring and promoting the growth of anammox bacteria in mainstream wastewater treatment that can guide the future implementation of anammox-based technologies.
The growth of suspended anammox sludge in pretreated municipal wastewater
Municipal wastewater favors the heterotrophic denitrification process over the anammox process due to a high BOD/N ratio and the presence of potential anammox inhibitors in the wastewater.5,6 While a treatment step for BOD removal would presumably take place prior to anammox treatment, very little work has been done to establish whether anammox bacteria can thrive under these conditions. It was only recently shown that granular anammox sludge can grow when municipal wastewater is first treated with a high-rate aerobic system to remove BOD.7 However, the DEMON® process which utilizes suspended anammox sludge is currently the main technology for side-stream processes in municipal wastewater treatment,2 and may be a preferred alternative in mainstream treatment as well. Now, Laureni et al. are the first to demonstrate growth of suspended anammox sludge in municipal wastewater, also using a high-rate aerobic system as a pretreatment step.8
Two reactors were inoculated with suspended nitritation/anammox sludges from side-stream treatment of municipal wastewater and operated with micro-aeration in an attempt to achieve both partial nitritation and anammox treatment in the same reactor. The inocula had similar compositions but different morphologies, with Reactor 1 having large aggregates and Reactor 2 having smaller, denser aggregates, shown in Fig. 1. The large aggregates in Reactor 1 broke apart, lost rapid settling properties, and washed out, while the smaller aggregates in Reactor 2 maintained their structure and were retained. The wash out of ammonia oxidizing bacteria (AOB) precluded partial nitritation and disrupted anammox activity, but anammox activity was restored with a switch to synthetic wastewater and maintained when pretreated wastewater supplemented with nitrite was used as the feed. Low temperature had a large impact on anammox activity, but at even as low as 12.5 °C, activity was comparable to typical values for municipal wastewater treatment (46 mg N L−1 d−1). This study confirms the viability of anammox bacteria in pretreated municipal wastewater at a variety of temperatures and highlights the need to develop solutions for reactor startup and partial nitritation in mainstream treatment systems.
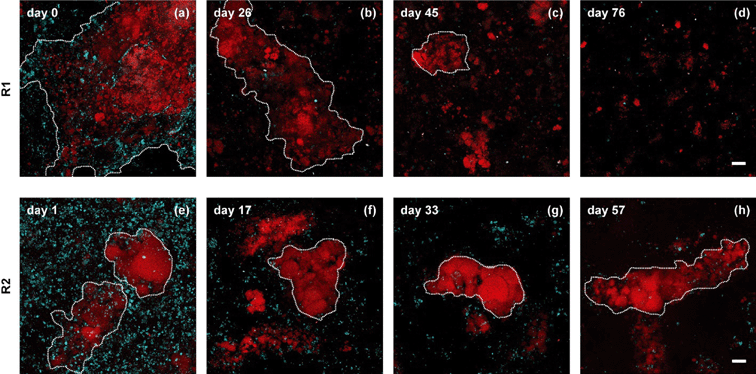 |
| Fig. 1 Fluorescence in situ hybridization – confocal laser scanning microscopy images illustrate changes in AOB and anammox bacteria during the startup periods for Reactor 1 (a–d) and Reactor 2 (e–h). Anammox populations are displayed with red color allocation and AOB in cyan. The scale bar is 20 μm. Reprinted with permission from M. Laureni, D. G. Weissbrodt, I. Szivák, O. Robin, J. L. Nielsen and E. Morgenroth, A. Joss, Water Res., 2015, 80, 325–336. Copyright 2015 Elsevier. | |
Effects of reactor configuration on partial nitritation/anammox at low temperatures
Currently, side-stream applications of anammox are operated above 30 °C, compared to the 10–20 °C temperature range for mainstream municipal wastewater treatment in moderate climates. It is known that anammox activity is greatly reduced at lower temperatures, though it can be maintained at practical levels as described above.8 Temperature effects are not isolated to anammox, however, as it can also affect AOB responsible for partial nitritation and NOB that produce nitrate. Nitrate is particularly undesirable as it cannot be removed in these systems. These interactions could impact anammox activity for mainstream municipal wastewater treatment, and the results of enriched anammox reactors would not be comparable. Gilbert et al. studied the effects of reactor configuration on combined partial nitritation/anammox, simulating temperature gradients from 20 °C to 10 °C observed in municipal wastewater treatment plants in moderate climates.2 Semi-batch reactors (SBRs) with suspended or granulated sludge, and MBBRs with 2 mm or 10 mm carriers were tested (Fig. 2).
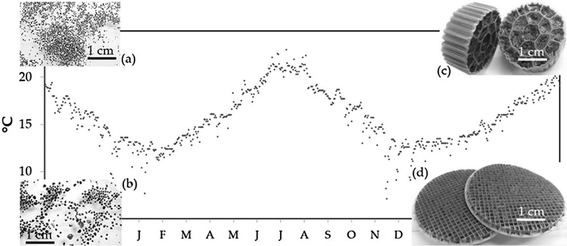 |
| Fig. 2 Temperature profile of water at a municipal wastewater treatment plant, and anammox bacteria in (a) suspended sludge, (b) granulated sludge, (c) 2 mm carriers, and (d) 10 mm carriers. Reprinted with permission from E. M. Gilbert, S. Agrawal, T. Schwartz, H. Horn, and S. Lackner, Water Res., 2015, 81, 92–100. Copyright 2015 Elsevier. | |
Nitrite accumulation occurred in the suspended sludge beginning at 16 °C and all other reactors beginning at 13 °C, indicating reduced anammox activity. Reactor configuration determined the thickness of the biofilms, with suspended anammox sludge having the thinnest biofilms, and the 10 mm carriers having the thickest. Thicker biofilms minimized residual nitrite (nitrite formed from partial nitritation of ammonium, but not converted to nitrogen gas by anammox) in the effluent at low temperatures. Consequently, as temperatures were reduced to 10 °C, accumulation of residual nitrite was 90% for the suspended sludge, 50% for the granulated sludge and the 2 mm carrier, and less than 5% for the 10 mm carrier after acclimation. This demonstrates the critical role of biofilm in maintaining anammox activity at low temperatures. Nitrate production was present from the beginning and accounted for 25–30% of converted ammonium at 20 °C. In the SBRs, nitrate production declined with temperature and was negligible below 13 °C. Conversely, nitrate production was maintained or increased in the MBBRs before dropping below 10% of converted ammonium below 10 °C. Analysis of the bacteria via qPCR showed that AOB fractions were constant while NOB fractions increased with decreasing temperature. Anammox fractions declined with temperature in both SBRs but were stable in the MBBRs. The study demonstrates the importance of biofilm thickness for resilient anammox activity at low temperatures, and only the MBBR with the 10 mm carrier shows the thickest biofilms of 10 mm and generated acceptable effluent at 10 °C.
Increasing anammox growth rates
Even with high levels of nitrogen and elevated temperatures, anammox in municipal side-stream processes requires doubling times of 15–30 d.9 This growth rate is slow compared to other chemolithotrophs, and presents an obstacle for mainstream municipal treatment due to the demands of reactor volume and biomass retention, as well as long periods required for start-up and for recovery from process disruptions. This problem further exacerbates challenges associated with operation at low temperatures in moderate climates. The study in which Lotti et al. were able to increase the growth rate of a previously stable anammox community by reducing solids retention time (SRT) suggests that this slow growth rate is not an inherent limitation.9
A reactor highly enriched with anammox (97%) was operated at an SRT of 12 d from day 0 to day 68, and at a reduced SRT of 4.1 d from day 69 to day 187 (Fig. 3). Following an adaptation period, the biomass specific maximum nitrite removal rate of the reactor increased while doubling time decreased. After day 187, the SRT was decreased stepwise until nitrite accumulation occurred, at an SRT of 3 d. The observed growth rate, 0.334 d−1, is the highest in the reported literature and four times faster than any previous direct measurements. Analysis of nitrite concentrations suggests that the increase in growth rate is best explained by the increase in electron transfer capacity and the biomass specific substrate uptake rate. However the mechanism of the increase is not clear. The adaptation period required prior to the improved kinetics was over 40 generations, much longer than would be expected for metabolic up-regulation. Phylogenetic analysis showed no change in the dominant anammox strain, while analysis of the internal transcribed spacer region suggests that no major mutations occurred. Regardless of the reason for the change, the electron transfer rates observed in this study were comparable to that of other chemolithotrophs, indicating that the view of anammox as an intrinsically slow growing organism is incorrect. If these growth rates can be achieved in treatment systems, it could substantially change our understanding of the capabilities and limitations of these systems.
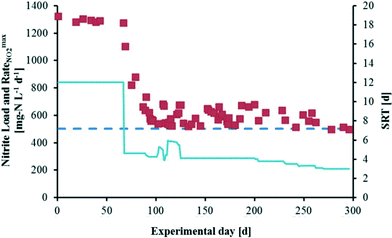 |
| Fig. 3 Reactor operation. The dashed line represents the volumetric nitrite loading rate, the red squares represent maximum volumetric nitrite removal rate measured in ex situ batch experiments, and the solid line represents SRT. Adapted with permission from T. Lotti, R. Kleerebezem, J. M. Abelleira-Pereira, B. Abbas and M. C. M. van Loodsdrecht, Water Res., 2015, 81, 261–268. Copyright 2015 Elsevier. | |
Can we measure anammox activity in partial nitritation/anammox reactors with mass balances?
The ability to monitor anammox activity in mainstream treatment is not a trivial problem. In a single-stage partial nitritation/anammox reactor with high ratios of BOD to nitrogen, it is possible for heterotrophic bacteria to mask the decrease of anammox activity by taking over a substantial portion of nitrogen removal.10 While a variety of methods exist for determining anammox concentration and activity,11 it is desirable to utilize parameters that are already routinely monitored (i.e. BOD, dissolved oxygen (DO), ammonium, nitrite, etc.) instead of acquiring new instrumentation for this specific purpose. Schielke-Jenni et al. evaluated whether it was theoretically and practically possible to distinguish between anammox and heterotrophic nitrogen removal by using these parameters and the activated sludge model (Fig. 4).12
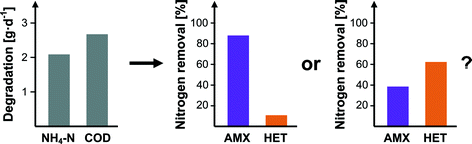 |
| Fig. 4 Can we detect shifts in nitrogen removal from anammox activity to heterotrophic denitrification? Reproduced from ref. 12. | |
In order to develop a system of solvable equations, it is necessary to assume that stoichiometric and kinetic parameters are known for all reactions, and that the process is at steady state. If so, the measurement of flow rates, BOD, nitrite, nitrate, ammonium, alkalinity, total inorganic carbon, pH, and oxygen is sufficient to solve the system of equations and obtain concentrations of AOB, NOB, anammox, and oxygen-, nitrite-, and nitrate-respirating heterotrophic bacteria. However, propagation of error is problematic, as a 5% standard deviation in conversion rate measurements (an optimistic estimate) results in a 1210% standard deviation for anammox concentrations. This can be overcome in part with high-rate automated sensors, with 600 measurements necessary to achieve a 50% standard deviation for anammox concentrations. Development of reliable nitrite sensors would enable this method as a viable option.
Outlook
These papers describe some of the recent progress made in and challenges that remain for mainstream removal of nitrogen via anammox. Anammox bacteria are viable and active in municipal wastewater treated via high-rate aerobic treatment, even at low temperatures. However, achieving partial nitritation together with anammox at low temperatures remains a challenge, for which attached growth strategies with thick biofilms may be a solution. The potential for faster growth of anammox bacteria may open up new possibilities for the design of anammox treatment, and it also indicates there are still many unknowns about these organisms to be explored. It is also theoretically possible to differentiate anammox from heterotrophic activity and evaluate anammox activity based on routine wastewater treatment parameters, but requires the development and implementation of highly sensitive sensors to reduce uncertainty. In addition to the challenges discussed in the article, many others need to be tackled. For example, the combination of anammox treatment for anaerobically treated municipal wastewater needs to be assessed. If successful, it will further improve energy efficiency and recovery. Evaluation of the performance of anammox and conventional nitrification should not just focus on nitrogen removal and energy savings. It is also necessary to investigate micropollutant removal in the anammox treatment because it is largely unknown, while conventional nitrification degrades many micropollutants effectively. Though challenges remain, anammox treatment offers an exciting possibility for mainstream municipal wastewater treatment for desired nitrogen removal and significant energy savings.
References
- S. Lackner, E. M. Gilbert, S. E. Vlaeminck, A. Joss, H. Horn and M. C. M. van Loosdrecht, Water Res., 2014, 55, 292–303 CrossRef CAS PubMed.
- E. M. Gilbert, S. Agrawal, T. Schwartz, H. Horn and S. Lackner, Water Res., 2015, 81, 92–100 CrossRef CAS PubMed.
- G. Xu, Y. Zhou, Q. Yang, Z. M. Lee, J. Gu, W. Lay, Y. Cao and Y. Liu, Appl. Microbiol. Biotechnol., 2015, 99, 2485–2490 CrossRef CAS PubMed.
- H. Gao, Y. D. Scherson and G. F. Wells, Environ. Sci.: Processes Impacts, 2014, 16, 1223–1246 CAS.
- M. Kumar and J. G. Lim, J. Hazard. Mater., 2010, 178, 1–9 CrossRef CAS PubMed.
- D. Güven, A. Dapena, B. Kartal, M. C. Schmid, B. Mass, K. T. Pas-Schoonen, S. Sozen, R. Mendez, H. J. M. Camp, M. S. M. Jetten, M. Strous and I. Schmidt, Appl. Environ. Microbiol., 2005, 71, 1066–1071 CrossRef PubMed.
- T. Lotti, R. Kleerebezem, C. V. T. Kip, T. L. G. Hendrickx, J. Kruit, M. Hoekstra and M. C. M. van Loosdrecht, Environ. Sci. Technol., 2014, 48, 7874–7880 CrossRef CAS PubMed.
- M. Laureni, D. G. Weissbrodt, I. Szivák, O. Robin, J. L. Nielsen, E. Morgenroth and A. Joss, Water Res., 2015, 80, 325–336 CrossRef CAS PubMed.
- T. Lotti, R. Kleerebezem, J. M. Abelleira-Pereira, B. Abbas and M. C. M. van Loodsdrecht, Water Res., 2015, 81, 261–268 CrossRef CAS PubMed.
- S. Jenni, S. E. Vlaeminck, E. Morgenroth and K. M. Udert, Water Res., 2014, 49, 316–326 CrossRef CAS PubMed.
- S. M. Podmirseg, T. Pümpel, R. Markt, S. Murthy, C. Bott and B. Wett, Water Res., 2015, 68, 194–205 CrossRef PubMed.
- S. Schielke-Jenni, K. Villez, E. Morgenroth and K. M. Udert, Environ. Sci.: Water Res. Technol., 2015, 1, 523–534 CAS.
|
This journal is © The Royal Society of Chemistry 2015 |
Click here to see how this site uses Cookies. View our privacy policy here.