DOI:
10.1039/C4RA13577F
(Paper)
RSC Adv., 2015,
5, 7720-7728
Deep eutectic solvent based on choline chloride and malonic acid as an efficient and reusable catalytic system for one-pot synthesis of functionalized pyrroles†
Received
1st November 2014
, Accepted 19th December 2014
First published on 22nd December 2014
Abstract
A deep eutectic solvent (DES) based on choline chloride and malonic acid was prepared easily with high purity at a low cost. It has been applied as a dual catalyst and reaction medium for the synthesis of functionalized pyrroles by a one-pot, four-component reaction of amines, aldehydes, 1,3-dicarbonyl compounds and nitromethane. This green solvent could be recycled and reused three times without loss of its efficiency.
Introduction
Developing new environmentally benign solvents is one of the key subjects in the chemistry industry. During the past years, ionic liquids (ILs) as a class of green solvents have been applied in many fields such as separation technology,1 biocatalysis2 and organic synthesis3 because of their distinctive properties such as low vapor pressure, non-volatility, good stability and recyclability. However, recent examples have also demonstrated the disadvantages of using ILs as solvents, such as high cost, difficult preparation and some toxic properties.4 Therefore, developing a simple synthetic and greener alternative solvent has very important practical significance. In recent years, low-cost eutectic mixtures including deep eutectic solvents (DESs) based on choline chloride (ChCl) first introduced by Abbott and co-workers,5 low-melting mixture (LMM) of sugar, urea and inorganic salts first described by König and co-workers,6 natural deep eutectic solvents (NADES) first described by Choi and co-workers,7 low-transition temperature mixtures (LTTMs) descried by Kroon and co-workers,8 and deep eutectic ionic liquids (DEILs) reported by Hillman's group9 with similar physical properties and phase behaviour to ILs, are gaining increasing attention in different fields of chemistry. Compared with ILs, the synthetic process of these eutectic mixtures is relatively simple, only requiring mixing of two or more compounds under heating until a homogenous liquid is formed without any purification and 100% atom untilzation rate.10 In addition, the synthetic materials of these eutectic mixtures are abundant, low-toxic, relatively cheap, and biodegradable, which makes them particularly desirable for large-scale applications successfully in industrial production.11 To date, they have been applied to many chemical processing such as extraction,12 polymerizations,13 biomass processing,14 materials synthesis15 and organic reactions.16
The pyrrole nucleus is one of the most relevant simple heterocycles found in a large number of natural products, agrochemicals, and pharmaceuticals.17 Many pyrrole-containing compounds possess potent biological activity.18,19 Furthermore, pyrrole derivatives are particularly important in materials science.20 Consequently, the preparation of pyrroles has received considerable attention and a number of synthetic routes have been reported, such as Hantzsch,21 Paal–Knorr,22 and Clauson-Kaas reactions.23 Multicomponent reactions (MCRs) have been extensively used in organic synthesis. Up to date, MCRs have emerged as vital tools for the preparation of substituted and functionalized pyrrole derivatives from simple materials.24 In 2010, Jana and co-workers reported one-pot, four-component coupling reactions of 1,3-dicarbonyl compounds, amines, aromatic aldehydes, and nitroalkanes for the synthesis of highly functionalized pyrroles catalyzed by FeCl3. However, this method suffers from the shortcoming of long reaction time, low yield of products and tedious work-up procedure.25 In the past several years, great efforts have been made in this area in order to develop a simple and efficient method to improve this conversion. Khan,26 Saeidian,27 Jeong,28 and Zhang29 have reported some metal-catalyzed methods for this conversion. Despite the advances achieved, all of these methods used transition metals, and these bring great difficulties form the separation and purification of the target products, especially in the synthesis of pharmaceutical molecules. To solve these problems, a series of metal-free catalyzed methods for this transformation have been reported, for example, the use of I2,30 Amberlyst-15,31 bromodimethylsulfonium bromide (BDMS),32 and ionic liquid.33 However, the major limitation of these methods lies in the variable amines that must be aliphatic amines or benzylamines. Montmorillonite clay,34 gluconic acid aqueous solution (GAAS)35 and 1-butyl-3-methylimidazolium hydrogen sulfate ([bmim]HSO4)36 have also been used for this four-component reaction. However, these methods required long reaction times. Thus, the development of a simple, more efficient method for the synthesis of pyrroles remains an attractive goal.
As a part of our ongoing research program aimed at developing multicomponent reactions37 and environmental benign synthetic methodologies,38 herein we wish to report a simple, practical and metal-free method for the construction of N-protected functionalized pyrroles by one-pot, four-component reaction of amines, aldehydes, 1,3-dicarbonyl compounds and nitromethane in choline chloride–malonic acid (Scheme 1).
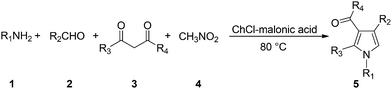 |
| Scheme 1 Synthesis of functionalized pyrroles using ChCl–malonic acid. | |
Results and discussion
The preparation of choline chloride–malonic acid based DES was performed by stirring ChCl (1 mol) and malonic acid (1 mol) at 80 °C until a homogenous liquid was formed. This method gave DES with 100% atom economy since it completely forms a eutectic mixture with no by-product formation. Thermal stability of ChCl–malonic acid was investigated by TG analysis in the N2 atmosphere, which showed that there were two steps of weight loss (Fig. 1). The weight loss at temperatures below 110 °C was due to the removal of water. The weight loss of the ChCl–malonic acid system was nearly 43.6% in the temperature range 110–160 °C, which was attributed to the complete decomposition of malonic acid. In addition, there is less weight loss when ChCl–malonic acid was heated for 5 h at100 °C, which indicates that this DES is stable under 100 °C. It was hence tempting and logical to study its potential as a green solvent in organic synthesis.
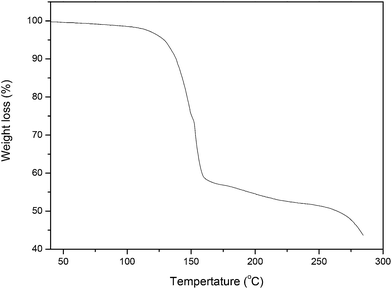 |
| Fig. 1 TG analysis of ChCl–malonic acid. | |
To optimize the reaction conditions, the model reaction of aniline, 4-chlorobenzaldehyde, acetylacetone and nitromethane was investigated and the results are recorded in Table 1. To initiate our study, the model reaction was performed in the absence of catalyst under solvent-free condition and only a trace amount of the desired product was detected after prolonged reaction time (Table 1, entry 1). The commercial available ionic liquids such as bmimBF4 and bmimPF6 were explored, no improvement was observed. Recently, Handy and Lavender reported that ChCl/urea DES could been used as effective solvent/catalyst for synthesis of pyrrole via the Paal–Knorr reaction,39 however, this four-component reaction could not proceed at all in this DES (entry 4). Furthermore, the use of some ChCl based on DESs such as ChCl + oxalic acid/tartaric acid/citric acid provided the desired product in very low yields. When the reaction was performed in ChCl
:
FeCl3 or ChCl
:
ZnBr2, the yields of product were significantly improved. This finding encouraged us to test other DESs. Previously, Abbott and co-workers reported that DESs can be formed between ChCl and dicarboxylic acids such as malonic or succinic acid, and their physical properties of these DESs such as viscosity, conductivity and surface tension were also investigated.5b Subsequently, the model reaction was undertaken in ChCl + itaconic acid/fumaric acid/malic acid/succinic acid/malonic acid. To our delight, ChCl–malonic acid was found to be a more efficient medium and provided desired product in remarkably high yield (88% yield, entry 14). The difference in the catalytic efficiency of these DESs may be due to their varying acidity. A lower reaction temperature of 60 °C decreased the yield to 52% (Table 1, entry 17), but a higher reaction temperature (90 °C) did not make a significant effect in the yield of product (Table 1, entry 18). The reaction in the presence of individual component such as ChCl and malonic acid instead of DES was also investigated. It was found that 12% yield of product was obtained when only malonic acid was used where no reaction was observed in the case of ChCl in the absence of malonic acid, which indicated that neither ChCl nor malonic acid promotes the reaction alone. The co-existence of ChCl and malonic acid has shown the synergetic effect on the reaction, which is perhaps the main reason for the high catalytic activity of the DES. This result is consistent with ChCl/oxalic acid system.16j Malonic acid only forms deep eutectic solvent with choline chloride, and it does not participate in the reaction. Finally, we examined the reaction with various amount of ChCl–malonic acid. The reaction proceeded smoothly to give the desired product 5m in 88% yield when 0.5 g and 1.0 g ChCl–malonic acid was used. A slightly lower yield was achieved using 1.5 g ChCl–malonic acid. Thus, the most suitable reaction conditions for the model reaction were established (Table 1, entry 14).
Table 1 Reaction of 4-chlorobenzaldehyde, aniline, acetylacetone and nitromethane in various DESsa
To demonstrate the industrial applicability of this protocol, the model reaction was carried out on a large scale (50 mmol). The reaction was completed in 1 h and afforded the corresponding product in 89% yield (Table 1, entry 21).
With these findings in hand, we examined this method to a wider range of amines, aldehydes, and 1,3-dicarbonyl compounds and the results was depicted in Table 2. Firstly, diverse amines were investigated. The results demonstrated that a wide range of anilines, regardless of electron-donating or electron-withdrawing groups on the benzene ring, could undergo the reaction smoothly to afford the expected products in moderate to good yields. Anilines bearing a weakly electron-withdrawing were a little bit less efficient in the reaction. A decrease in reactivity was observed when ortho-substitution substrate was used (Table 2, entry 5), thus revealing a strong steric effect. Noticeably, the notoriously difficult 4-nitroaniline was also successfully converted into the corresponding product 5i (Table 2, entry 9). It is worth mentioning that the reaction time was shortened obviously compared with previous reported method.35 In addition, this reaction was amenable to polycyclic aromatic substrate such as naphthylamine, and the corresponding product 5g was obtained in high yield (Table 2, entry 10). Amine containing heteroaromatic group such as furan-2-ylmethanamine was also subjected to the standard reaction conditions, very valuable product 5k was obtained in good yield (Table 2, entry 11). Moreover, prop-2-en-1-amine could be employed as a facile substrate and provided the corresponding products in good yields (Table 2, entries 18 and 19). Also, it was found that different kinds of aliphatic amines reacted successfully to afford the desired products under the same reaction conditions in apparently shorter reaction time (Table 2, entries 12 and 13).
Table 2 Synthesis of multisubstituted pyrroles 5
Entry |
Aldehyde |
Amine |
R3 |
R4 |
Product |
Time/h |
Yielda/% |
Ref. |
Isolated yield. |
1 |
PhCHO |
PhNH2 |
Me |
Me |
5a |
1.0 |
90 |
25 |
2 |
PhCHO |
4-CH3C6H4NH2 |
Me |
Me |
5b |
1.0 |
90 |
35 |
3 |
PhCHO |
4-C(CH3)3C6H4NH2 |
Me |
Me |
5c |
1.0 |
91 |
35 |
4 |
PhCHO |
4-FC6H4NH2 |
Me |
Me |
5d |
1.0 |
87 |
34 |
5 |
PhCHO |
2-ClC6H4NH2 |
Me |
Me |
5e |
1.8 |
56 |
|
6 |
PhCHO |
3-ClC6H4NH2 |
Me |
Me |
5f |
1.2 |
80 |
24b |
7 |
PhCHO |
4-ClC6H4NH2 |
Me |
Me |
5g |
1.0 |
89 |
26 |
8 |
PhCHO |
4-BrC6H4NH2 |
Me |
Me |
5h |
1.0 |
86 |
35 |
9 |
PhCHO |
4-NO2C6H4NH2 |
Me |
Me |
5i |
1.5 |
52 |
35 |
10 |
PhCHO |
 |
Me |
Me |
5j |
1.5 |
60 |
24b |
11 |
PhCHO |
 |
Me |
Me |
5k |
0.5 |
80 |
|
12 |
PhCHO |
PhCH2NH2 |
Me |
Me |
5l |
0.5 |
82 |
24b |
13 |
PhCHO |
 |
Me |
Me |
5m |
0.5 |
85 |
35 |
14 |
4-CH3C6H4CHO |
PhNH2 |
Me |
Me |
5n |
1.0 |
90 |
35 |
15 |
4-ClC6H4CHO |
PhNH2 |
Me |
Me |
5o |
1.0 |
88 |
35 |
16 |
4-NO2C6H4CHO |
PhNH2 |
Me |
Me |
5p |
1.5 |
78 |
35 |
17 |
4-CF3C6H4CHO |
PhNH2 |
Me |
Me |
5q |
1.5 |
75 |
35 |
18 |
 |
H2C CHCH2NH2 |
Me |
Me |
5r |
1.5 |
70 |
|
19 |
 |
H2C CHCH2NH2 |
Me |
Me |
5s |
1.5 |
72 |
|
20 |
 |
PhNH2 |
Me |
Me |
5t |
1.5 |
81 |
29 |
21 |
PhCHO |
PhNH2 |
Me |
OMe |
5u |
1.5 |
80 |
35 |
22 |
PhCHO |
PhNH2 |
Me |
OEt |
5v |
1.5 |
85 |
35 |
23 |
PhCHO |
PhNH2 |
Me |
OCH2CH2OMe |
5w |
1.5 |
80 |
35 |
24 |
PhCHO |
PhNH2 |
Me |
OCH2CH CH2 |
5x |
1.5 |
76 |
35 |
25 |
PhCHO |
PhNH2 |
Me |
OC(CH3)3 |
5y |
2.0 |
83 |
|
26 |
PhCHO |
PhNH2 |
Me |
OCH2CH(CH3)2 |
5z |
2.0 |
80 |
|
27 |
PhCHO |
PhNH2 |
Et |
OMe |
5aa |
2.0 |
78 |
29 |
Next, the substrate scope of aldehydes with a variety of substituents was also investigated in this 4CRs. The aromatic ring of benzaldehyde bearing electron-withdrawing or electron-donating groups afforded the desired products in high yields, showing no significant influence on the reaction time and the yield of the products. Notably, heteroaromatic aldehydes such as 2-furaldehyde and 2-thiophenealdehyde were tolerated and furnished the corresponding products 5r and 5s in excellent yields. 1-Naphthaldehyde also afforded the product 5t in 80% yield. Unfortunately, alkyl aldehyde such as cyclohexanecarboxaldehyde or benzylic aldehyde provided inseparable complex mixtures under similar reaction conditions.
Encouraged by the good results with acetylacetone, we further expand the reaction scope. A variety of 1,3-dicarbonyl compounds such as methyl acetoacetate, ethyl acetoacetate, 2-methoxyethyl acetoacetate, allyl acetoacetate, tert-butyl 3-oxobutanoate, isobutyl 3-oxobutanoate and methyl 3-oxopentanoate were applied to this 4CRs, the desired products were isolated in high yields (Table 2, entries 21–27).
The structures of the products were determined by their FTIR, 1H NMR, 13C NMR spectra and elemental analysis. Furthermore, the structure of compound 5c was unambiguously confirmed by single crystal X-ray analysis (Fig. 2).
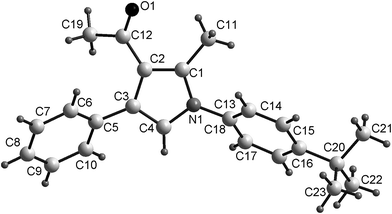 |
| Fig. 2 Single-crystal X-ray structural of compound 5c.† | |
Based on the literatures,34 the product formation for this four-component reaction can be mechanistically rationalized via a domino reaction pathway (Scheme 2). We propose that choline chloride–malonic acid DES catalyzes the reaction via hydrogen-bonding activation of carbonyl group of acetylacetone and increases its electrophilicity, thereby facilitating the attack of aniline to generate the enaminone A. Similarly, it also forms hydrogen bond with the oxygen of carbonyl group of 4-chlorobenzaldehyde to facilitate the Knoevenagel condensation with nitromethane to produce intermediate B. The presence of intermolecular hydrogen bonding plays an important role and provides additional attractive forces between molecules. The DES also might assist in improving the reactivity of enaminone A, which participates in the Michael addition with B to give intermediate C. This can be verified by the fact that intermediate A and B could be isolated in the present reaction process. In addition, product 5o can be obtained by two-component reaction of A and B in this DES. Subsequently, an intramolecular cyclization of intermediate C involving the attack by the imine nitrogen to the nearby –C
N(O)OH moiety affords intermediate D. Finally the intermediate D undergoes aromatization by the elimination of nitroxyl (HNO) and water molecule to lead the final product 5o.
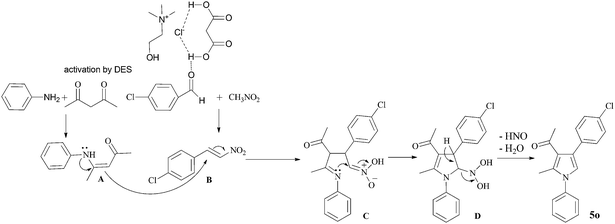 |
| Scheme 2 Plausible mechanism for the synthesis of pyrrole 5o. | |
Deep eutectic solvents as a novel reaction media have been used to the development of a green protocol for the simple and efficient preparation of various heterocyclic compounds owing to its atom economy and ease of recovery and reuse. Thus, the reusability of the DESs was investigated by using aniline, 4-chlorobenzaldehyde, acetylacetone and nitromethane as starting materials under optimized conditions (Table 3). Upon completion of the reaction, water was added to the reaction mixture. The DES was dissolved in water and the crude product was obtained by extraction with EtOAc. The DES was recovered by evaporating water at 80 °C under vacuum and recycled for the next batch. The result of the reusability of the DES was illustrated in Table 3. The DES can be recycled for up to five times without significant loss in yield, which shows that this DES possessed excellent activity and reusability.
Table 3 Recycling of the solvent
Entry |
Reaction runs |
Yielda (%) |
Isolated yield. |
1 |
1st |
88 |
2 |
2nd |
88 |
3 |
3rd |
87 |
4 |
4th |
85 |
5 |
5th |
84 |
Finally, in order to show the merit of the present method, we have compared our result with the most recently reported catalytic systems in the literatures. As can be seen in Table 4, it is clear that the present protocol is superior in terms of reaction time and product yield.
Table 4 Comparison of our result with previously reported systems
Entry |
Catalyst |
Reaction conditions |
Product |
Time |
Yield (%) |
1 |
FeCl3 (10 mol%) |
Reflux |
5a |
14 h |
54 (ref. 25) |
2 |
NiCl2·6H2O (10 mol%) |
Reflux |
5a |
10 h |
52 (ref. 26) |
3 |
Silica supported tungsticacid (10 mol%) |
Reflux |
5y |
4 h |
79 (ref. 28) |
4 |
Magnetic nanoparticle CoFe2O4 supported Mo (1.0 mol%) |
90 °C |
5a |
4 h |
90 (ref. 29) |
5 |
I2 (10 mol%) |
90 °C |
5l |
6 h |
85 (ref. 30) |
6 |
Amberlyst-15 (10 wt%) |
Ultrasound |
5u |
4 h |
72 (ref. 31) |
7 |
BDMS (10 mol%) |
Room temperature |
5u |
9 h |
70 (ref. 32) |
8 |
Montmorillonite clay K-10 (10 mol%) |
60 °C |
5a |
6 h |
70 (ref. 34) |
9 |
GAAS (5 ml) |
100 °C |
5a |
7 h |
87 (ref. 35) |
10 |
[bmim]HSO4 (20 mol%) |
90 °C |
5a |
3 h |
90 (ref. 36) |
11 |
ChCl–malonic acid (0.1g) |
80 °C |
5a |
1 h |
90 (this work) |
Conclusions
In conclusion, we have developed a simple, green, and highly efficient procedure for synthesis of diversely substituted pyrroles by one-pot, four-component coupling reaction of amines, aldehydes, 1,3-dicarbonyl compounds and nitromethane. The reaction proceeded in deep eutectic solvent and provided the corresponding products in good to excellent yields. The good catalytic potency and excellent recyclability of choline chloride–malonic acid make this protocol more useful for preparation of multisubstituted pyrroles in large scale over reported methodologies.
Experimental section
General information
All solvents and chemicals were obtained commercially and were used without further purification. Melting points were measured on an X-4 digital melting point apparatus and were corrected with benzoic acid. IR spectra were obtained as KBr pellets or as liquid films on KBr pellets with a Bruker-TENSOR 27 spectrometer. 1H NMR (500 MHz) and 13C NMR (125 MHz) spectra were recorded on Bruker DRX-500 spectrometer using CDCl3 as the solvent and TMS as an internal standard. Elemental analyses were determined on a Vario EL III CHNOS elemental analyzer.
Preparation of deep eutectic solvents
A mixture of choline chloride and the second component was heated until a clear liquid appeared, then allowed to cool at room temperature and used without further purification.
General procedure for synthesis of functionalized pyrroles 5
A mixture of aldehyde (1 mmol), amine (1 mmol), 1,3-dicarbonyl compound (1 mmol), CH3NO2 (3 mmol) in ChCl–malonic acid (0.5 g) was stirred at 80 °C (monitored by TLC). Upon completion of the reaction, the reaction mixture was cooled to room temperature and water (5 mL) was added. The ChCl–malonic acid was dissolved in water and the products were extracted with EtOAc (3 × 5 mL). Pure products were obtained by evaporation of the solvent, followed by recrystallization from ethanol or by column chromatography on silica gel using ethyl acetate/hexane as the eluent.
1-(2-Methyl-1,4-diphenyl-1H-pyrrol-3-yl)ethanone (5a). White solid, mp 106–107 °C; 1H NMR (CDCl3, 500 MHz) δ 2.08 (s, 3H), 2.41 (s, 3H), 6.68 (s, 1H), 7.31–7.35 (m, 3H), 7.38–7.39 (m, 4H), 7.43 (t, J = 7.5 Hz, 1H), 7.49–7.51 (m, 2H) ppm; 13C NMR (CDCl3, 125 MHz) δ 12.9, 31.2, 120.6, 122.6, 126.3, 126.3, 126.9, 128.1, 128.3, 129.4, 129.4, 135.3, 136.0, 138.8, 197.7 ppm.
1-(2-Methyl-4-phenyl-1-(p-tolyl)-1H-pyrrol-3-yl)ethanone (5b). White solid, mp 109–111 °C; 1H NMR (CDCl3, 500 MHz) δ 2.07 (s, 3H), 2.40 (s, 3H), 2.42 (s, 3H), 6.64 (s, 1H), 7.21 (d, J = 8.5 Hz, 2H), 7.26–7.32 (m, 3H), 7.37–7.38 (m, 4H) ppm; 13C NMR (CDCl3, 125 MHz) δ 12.9, 21.1, 31.1, 120.7, 122.4, 126.1, 126.2, 126.8, 128.3, 129.4, 129.9, 135.4, 136.1, 136.2, 138.1, 197.6 ppm.
1-(1-(4-(tert-Butyl)phenyl)-2-methyl-4-phenyl-1H-pyrrol-3-yl)ethanone (5c). White solid, mp 148–149 °C; 1H NMR (CDCl3, 500 MHz) δ 1.37 (s, 9H), 2.08 (s, 3H), 2.41 (s, 3H), 6.66 (s, 1H), 7.25 (d, J = 8.5 Hz, 2H), 7.29–7.34 (m, 1H), 7.38–7.39 (m, 4H), 7.49 (d, J = 8.5 Hz, 2H) ppm; 13C NMR (CDCl3, 125 MHz) δ 13.0, 31.1, 31.4, 34.8, 120.7, 122.4, 125.8, 126.2, 126.3, 126.8, 128.3, 129.4, 135.5, 136.1, 136.2, 151.3, 197.7 ppm.
1-(1-(4-Fluorophenyl)-2-methyl-4-phenyl-1H-pyrrol-3-yl)ethanone (5d). White solid, mp 126–127 °C; 1H NMR (CDCl3, 500 MHz) δ 2.07 (s, 3H), 2.38 (s, 3H), 6.63 (s, 1H), 7.16 (t, J = 8.0 Hz, 2H), 7.29–7.31 (m, 3H), 7.36–7.37 (m, 4H) ppm; 13C NMR (CDCl3, 125 MHz) δ 12.8, 31.1, 116.4 (d, 2JCF = 22.8 Hz), 120.7, 122.6, 126.4, 126.9, 128.1 (d, 3JCF = 8.4 Hz), 128.3, 129.3, 134.8 (d, 4JCF = 2.9 Hz), 135.4, 135.9, 162.1 (d, 1JCF = 247.1 Hz), 197.5 ppm.
1-(1-(2-Chlorophenyl)-2-methyl-4-phenyl-1H-pyrrol-3-yl)ethanone (5e). Pale yellow solid, mp 98–99 °C; 1H NMR (CDCl3, 500 MHz) δ 2.09 (s, 3H), 2.28 (s, 3H), 6.56 (s, 1H), 7.34–7.29 (m, 1H), 7.44–7.35 (m, 7H), 7.57–7.55 (m, 1H) ppm; 13C NMR (CDCl3, 125 MHz) δ 12.3, 31.1, 76.8, 77.0, 77.3, 120.3, 121.9, 126.3, 126.8, 127.6, 128.2, 129.5, 129.6, 130.2, 130.5, 132.6136.0.136.4136.5197.5 ppm; anal. calcd for C19H16ClNO: C, 73.66; H, 5.21; N, 4.52; found: C, 73.42; H, 5.01; N, 4.70%; ESI-MS: m/z = 310 (M + 1)+.
1-(1-(3-Chlorophenyl)-2-methyl-4-phenyl-1H-pyrrol-3-yl)ethanone (5f). Yellow sticky liquid; 1H NMR (CDCl3, 500 MHz) δ 2.07 (s, 3H), 2.41 (s, 3H), 6.65 (s, 1H), 7.23–7.27 (m, 1H), 7.30–7.44 (m, 8H) ppm; 13C NMR (CDCl3, 125 MHz) δ 12.9, 31.1, 98.5, 120.4, 122.6, 123.0, 124.5, 125.4, 126.5, 126.7, 127.0, 128.4, 129.3, 130.4, 135.0, 135.1, 135.7, 139.9, 197.6 ppm.
1-(1-(4-Chlorophenyl)-2-methyl-4-phenyl-1H-pyrrol-3-yl)ethanone (5g). Yellow solid, mp 127–128 °C; 1H NMR (CDCl3, 500 MHz) δ 2.07 (s, 3H), 2.40 (s, 3H), 6.64 (s, 1H), 7.28 (d, J = 8.5 Hz, 2H), 7.31–7.40 (m, 5H), 7.47 (d, J = 8.5 Hz, 2H) ppm; 13C NMR (CDCl3, 125 MHz) δ 12.8, 31.1, 120.4, 122.9, 126.6, 126.9, 127.5, 128.3, 129.3, 129.6, 134.1, 135.1, 135.8, 137.3, 197.6 ppm.
1-(1-(4-Bromophenyl)-2-methyl-4-phenyl-1H-pyrrol-3-yl)ethanone (5h). White solid, mp 141–143 °C; 1H NMR (CDCl3, 500 MHz) δ 2.06 (s, 3H), 2.40 (s, 3H), 6.63 (s, 1H), 7.21 (d, J = 8.0 Hz, 2H), 7.31–7.39 (m, 5H), 7.61 (d, J = 8.0 Hz, 2H) ppm; 13C NMR (CDCl3, 125 MHz) δ 12.9, 31.1, 120.4, 122.0, 122.9, 126.7, 127.0, 127.8, 128.4, 129.3, 132.6, 135.1, 135.8, 137.8, 197.6 ppm.
1-(2-Methyl-1-(4-nitrophenyl)-4-phenyl-1H-pyrrol-3-yl)ethanone (5i). Yellow solid, mp 171–172 °C; 1H NMR (CDCl3, 500 MHz) δ 2.07 (s, 3H), 2.46 (s, 3H), 6.72 (s, 1H), 7.33–7.42 (m, 5H), 7.54 (d, J = 8.0 Hz, 2H), 8.39 (d, J = 8.0 Hz, 2H) ppm; 13C NMR (CDCl3, 125 MHz) δ 13.1, 31.2, 119.9, 124.1, 125.0, 126.5, 127.3, 127.5, 128.5, 129.2, 134.7, 135.3, 144.1, 146.8, 197.7 ppm.
1-(2-Methyl-1-(naphthalen-1-yl)-4-phenyl-1H-pyrrol-3-yl)ethanone (5j). White solid, mp 143–144 °C; 1H NMR (CDCl3, 500 MHz) δ 2.15 (s, 3H), 2.22 (s, 3H), 6.71 (s, 1H), 7.32 (t, J = 7.5 Hz, 1H), 7.38–7.49 (m, 6H), 7.51–7.59 (m, 3H), 7.97 (t, J = 9.0 Hz, 2H) ppm; 13C NMR (CDCl3, 125 MHz) δ 12.4, 31.2, 121.8, 121.9, 122.9, 125.3, 125.4, 126.1, 126.8, 126.9, 127.6, 128.3, 128.3, 129.4, 129.5, 130.6, 134.1, 135.3, 136.1, 137.1, 197.8 ppm.
1-(1-(Furan-2-ylmethyl)-2-methyl-4-phenyl-1H-pyrrol-3-yl)ethanone (5k). White solid, mp 77–78 °C; IR (KBr): 2926, 1637, 1604, 1500, 1168, 943 cm−1; 1H NMR (CDCl3, 500 MHz) δ 2.00 (s, 3H), 2.54 (s, 3H), 4.98 (s, 2H), 6.25 (d, J = 8.0 Hz, 1H), 6.34 (dd, J = 2.5, 2.0 Hz, 1H), 6.53 (s, 1H), 7.28–7.30 (m, 3H), 7.34 (t, J = 7.5 Hz, 2H), 7.38 (s, 1H) ppm; 13C NMR (CDCl3, 125 MHz) δ 11.2, 30.9, 43.1, 108.4, 110.4, 119.3, 121.8, 125.7, 126.5, 128.0, 129.1, 134.6, 136.0, 142.8, 149.2, 197.4 ppm; anal. calcd for C18H17NO2
:
C, 77.40; H, 6.13; N, 5.01; found: C, 77.32; H, 6.02; N, 4.98%; ESI-MS: m/z = 280 (M + 1)+.
1-(1-Benzyl-2-methyl-4-phenyl-1H-pyrrol-3-yl)ethanone (5l). White solid, mp 87–88 °C; 1H NMR (CDCl3, 500 MHz) δ 2.04 (s, 3H), 2.44 (s, 3H), 5.06 (s, 2H), 6.54 (s, 1H), 7.09 (d, J = 7.5 Hz, 2H), 7.26–7.37 (m, 8H) ppm; 13C NMR (CDCl3, 125 MHz) δ 11.6, 31.1, 50.3, 120.2, 122.1, 125.9, 126.7, 127.9, 128.3, 129.0, 129.4, 135.2, 136.4, 136.7, 197.5 ppm.
1-(1-Cyclopropyl-2-methyl-4-phenyl-1H-pyrrol-3-yl)ethanone (5m). White solid, mp 80–81 °C; 1H NMR (CDCl3, 500 MHz) δ 0.94–0.97 (m, 2H), 1.00–1.06 (m, 2H), 2.00 (s, 3H), 2.58 (s, 3H), 3.13–3.17 (m, 1H), 6.50 (s, 1H), 7.26–7.36 (m, 5H) ppm; 13C NMR (CDCl3, 125 MHz) δ 6.9, 12.2, 28.2, 31.1, 119.6, 122.0, 125.1, 126.7, 128.3, 129.4, 136.5, 137.4, 197.5 ppm.
1-(2-Methyl-1-phenyl-4-(p-tolyl)-1H-pyrrol-3-yl)ethanone (5n). White solid, mp 92–93 °C; 1H NMR (CDCl3, 500 MHz) δ 2.09 (s, 3H), 2.38 (s, 3H), 2.41 (s, 3H), 6.65 (s, 1H), 7.19 (d, J = 8.0 Hz, 2H), 7.26 (t, J = 7.5 Hz, 2H), 7.32 (d, 2H), 7.41 (t, J = 7.5 Hz, 1H), 7.48 (t, J = 7.5 Hz, 2H) ppm; 13C NMR (CDCl3, 125 MHz) δ 13.0, 21.2, 31.2, 120.5, 122.6, 126.2, 126.3, 128.1, 129.0, 129.2, 129.4, 133.0, 135.2, 136.5, 138.8, 197.8 ppm.
1-(4-(4-Chlorophenyl)-2-methyl-1-phenyl-1H-pyrrol-3-yl)ethanone (5o). White solid, mp 105–106 °C; 1H NMR (CDCl3, 500 MHz) δ 2.10 (s, 3H), 2.40 (s, 3H), 6.66 (s, 1H), 7.30–7.37 (m, 6H), 7.43 (t, J = 7.5 Hz, 1H), 7.50 (t, J = 7.5 Hz, 2H) ppm; 13C NMR (CDCl3, 125 MHz) δ 13.0, 31.2, 120.8, 122.5, 125.0, 126.3, 128.3, 128.5, 129.4, 130.5, 132.8, 134.5, 135.5, 138.6, 197.2 ppm.
1-(2-Methyl-4-(4-nitrophenyl)-1-phenyl-1H-pyrrol-3-yl)ethanone (5p). Brown sticky liquid; 1H NMR (CDCl3, 500 MHz) δ 2.18 (s, 3H), 2.40 (s, 3H), 6.78 (s, 1H), 7.34 (d, J = 7.0 Hz, 2H), 7.48 (d, J = 7.5 Hz, 1H), 7.51–7.54 (m, 4H), 8.25 (d, J = 8.5 Hz, 2H) ppm; 13C NMR (CDCl3, 125 MHz) δ 12.9, 31.3, 121.7, 122.5, 123.7, 124.1, 126.3, 128.6, 129.5, 129.6, 136.1, 138.3, 143.0, 146.5, 196.7 ppm.
1-(2-Methyl-1-phenyl-4-(4-(trifluoromethyl)phenyl)-1H-pyrrol-3-yl)ethanone (5q). Yellow sticky liquid; 1H NMR (CDCl3, 500 MHz) δ 2.12 (s, 3H), 2.41 (s, 3H), 6.72 (s, 1H), 7.33 (d, J = 7.5 Hz, 2H), 7.45 (t, J = 7.0 Hz, 1H), 7.51 (m, 4H), 7.64 (d, J = 8.5 Hz, 2H) ppm; 13C NMR (CDCl3, 125 MHz) δ 12.9, 31.2, 121.2, 122.5, 124.4 (q, 1JFC = 270.5 Hz), 125.2 (q, 3JFC = 3.6 Hz), 126.3, 128.4, 129.0, 129.4 (q, 2JFC = 12.5 Hz), 135.7, 138.5, 139.8, 197.0 ppm.
1-(1-Allyl-4-(furan-2-yl)-2-methyl-1H-pyrrol-3-yl)ethanone (5r). Yellow sticky liquid; IR (KBr): 2924, 1654, 1560, 1419, 1190, 1010, 937 cm−1; 1H NMR (CDCl3, 500 MHz) δ 2.15 (s, 3H), 2.46 (s, 3H), 4.45 (dt, J = 5.0, 1.5 Hz, 2H), 5.02 (d, J = 17.0 Hz, 1H), 5.23 (d, J = 10.0 Hz, 1H), 5.87–5.94 (m, 1H), 6.35 (d, J = 3.0 Hz, 1H), 6.46 (dd, J = 3.0, 2.0 Hz, 1H), 6.67 (s, 1H), 7.44 (s, 1H) ppm; 13C NMR (CDCl3, 125 MHz) δ 11.4, 29.8, 41.0, 107.7, 111.0, 114.6, 117.7, 120.9, 132.5, 135.5, 141.6, 149.1, 196.5 ppm; anal. calcd for C14H15NO2: C, 73.34; H, 6.59; N, 6.11; found: C, 73.30; H, 6.51; N, 5.99%; ESI-MS: m/z = 230 (M + 1)+.
1-(1-Allyl-2-methyl-4-(thiophen-2-yl)-1H-pyrrol-3-yl)ethanone (5s). Yellow sticky liquid; IR (KBr): 2920, 1654, 1560, 1541, 1411, 1265, 991 cm−1; 1H NMR (CDCl3, 500 MHz) δ 2.12 (s, 3H), 2.45 (s, 3H), 4.44 (dt, J = 5.5, 1.5 Hz, 2H), 5.03 (dd, J = 17.0, 1.0 Hz, 1H), 5.23 (dd, J = 10.5, 1.0 Hz, 1H), 5.87–5.95 (m, 1H), 6.58 (s, 1H), 6.93 (dd, J = 3.5, 1.0 Hz, 1H), 7.03 (dd, J = 5.0, 3.5 Hz, 1H), 7.25 (dd, J = 5.0, 1.0 Hz, 1H) ppm; 13C NMR (CDCl3, 125 MHz) δ 11.4, 30.5, 49.0, 117.0, 117.8, 121.0, 122.3, 124.8, 126.9, 127.1, 132.6, 135.3, 137.2, 196.9 ppm; anal. calcd for C14H15NOS: C, 68.54; H, 6.16; N, 5.71; found: C, 68.44; H, 6.01; N, 5.68%; ESI-MS: m/z = 246 (M + 1)+.
1-(2-Methyl-4-(naphthalen-1-yl)-1-phenyl-1H-pyrrol-3-yl)ethanone (5t). White solid, mp 114–115 °C; 1H NMR (CDCl3, 500 MHz) δ 1.70 (s, 3H), 2.53 (s, 3H), 6.73 (s, 1H), 7.38–7.52 (m, 9H), 7.84–7.89 (m, 2H), 7.95 (d, J = 8.5 Hz, 1H) ppm; 13C NMR (CDCl3, 125 MHz) δ 12.9, 31.1, 118.8, 119.6, 120.8, 122.5, 124.2, 126.3, 126.7, 127.7, 128.4, 129.4, 130.1, 133.6, 135.6, 136.1, 156.3, 157.5, 197.6 ppm.
Methyl 2-methyl-1,4-diphenyl-1H-pyrrole-3-carboxylate (5u). Yellow sticky liquid; 1H NMR (CDCl3, 500 MHz) δ 2.45 (s, 3H), 3.70 (s, 3H), 6.71 (s, 1H), 7.26–7.28 (m, 1H), 7.32–7.36 (m, 4H), 7.41–7.44 (m, 3H), 7.47–7.50 (m, 2H) ppm; 13C NMR (CDCl3, 125 MHz) δ 12.8, 50.7, 111.5, 121.0, 126.4, 126.7, 127.8, 128.2, 129.2, 129.4, 135.6, 136.8, 139.0, 166.3 ppm.
Ethyl 2-methyl-1,4-diphenyl-1H-pyrrole-3-carboxylate (5v). Yellow sticky liquid; 1H NMR (CDCl3, 500 MHz) δ 1.14 (t, J = 7.0 Hz, 3H), 2.46 (s, 3H), 4.18 (q, J = 7.0 Hz, 2H), 6.71 (s, 1H), 7.25–7.28 (m, 1H), 7.32–7.35 (m, 4H), 7.42–7.43 (m, 3H), 7.47–7.50 (m, 2H) ppm; 13C NMR (CDCl3, 125 MHz) δ 12.8, 14.2, 59.5, 111.8, 120.9, 126.4, 126.7, 127.6, 128.1, 129.4, 129.4, 135.7, 136.6, 139.0, 165.9 ppm.
2-Methoxyethyl 2-methyl-1,4-diphenyl-1H-pyrrole-3-carboxylate (5w). Yellow sticky liquid; 1H NMR (CDCl3, 500 MHz) δ 2.46 (s, 3H), 3.25 (s, 3H), 3.47 (t, J = 5.0 Hz, 2H), 4.28 (t, J = 5.0 Hz, 2H), 6.71 (s, 1H), 7.26–7.28 (m, 1H), 7.32–7.35 (m, 4H), 7.41–7.44 (m, 3H), 7.47–7.50 (m, 2H) ppm; 13C NMR (CDCl3, 125 MHz) δ 12.8, 58.7, 62.6, 70.4, 111.5, 121.0, 126.4, 126.9, 127.7, 128.2, 129.4, 135.7, 137.0, 139.0, 165.7 ppm.
Allyl 2-methyl-1,4-diphenyl-1H-pyrrole-3-carboxylate (5x). Yellow sticky liquid; 1H NMR (CDCl3, 500 MHz) δ 2.46 (s, 3H), 4.64 (d, J = 4.5 Hz, 2H), 5.09 (m, 2H), 5.79–5.86 (m, 1H), 6.71 (s, 1H), 7.26–7.28 (m, 1H), 7.32–7.35 (m, 4H), 7.42–7.43 (m, 3H), 7.48–7.51 (m, 2H) ppm; 13C NMR (CDCl3, 125 MHz) δ 12.9, 64.4, 111.5, 117.4, 121.1, 126.4, 126.4, 126.9, 127.8, 128.2, 129.4, 129.4, 132.6, 135.6, 136.9, 139.0, 165.5 ppm.
tert-Butyl 2-methyl-1,4-diphenyl-1H-pyrrole-3-carboxylate (5y). Yellow sticky liquid; IR (KBr): 2976, 1691, 1502, 1290, 1138, 1030, 962 cm−1; 1H NMR (CDCl3, 500 MHz) δ 1.35 (s, 9H), 2.44 (s, 3H), 6.69 (s, 1H), 7.26 (t, J = 7.5 Hz, 1H), 7.31–7.35 (m, 4H), 7.39–7.43 (m, 3H), 7.48 (t, J = 8.0 Hz, 2H) ppm; 13C NMR (CDCl3, 125 MHz) δ 12.5, 28.1, 79.7, 120.5, 126.2, 126.3, 126.5, 126.7, 127.6, 128.0, 129.0, 129.3, 136.0, 136.0, 165.5 ppm; anal. calcd for C22H23NO2: C, 79.25; H, 6.95; N, 4.20; found: C, 79.23; H, 6.88; N, 4.18%; ESI-MS: m/z = 334 (M + 1)+.
iso-Butyl 2-methyl-1,4-diphenyl-1H-pyrrole-3-carboxylate (5z). Yellow sticky liquid; IR (KBr): 2958, 1697, 1523, 1408, 1224, 1138, 985 cm−1; 1H NMR (CDCl3, 500 MHz) δ 0.78 (d, J = 7.0 Hz, 6H), 1.76–1.84 (m, 1H), 2.50 (s, 3H), 3.95 (d, J = 6.5 Hz, 2H), 6.72 (s, 1H), 7.28 (t, J = 7.5 Hz, 1H), 7.34–7.37 (m, 4H), 7.42–7.46 (m, 3H), 7.50 (t, J = 7.0 Hz, 2H) ppm; 13C NMR (CDCl3, 125 MHz) δ 12.8, 19.2, 27.7, 70.0, 111.8, 120.9, 126.3, 126.8, 127.8, 128.1, 129.3, 129.4, 135.8, 136.6, 139.0, 166.0 ppm; anal. calcd for C22H23NO2: C, 79.25; H, 6.95; N, 4.20; found: C, 79.23; H, 6.86; N, 4.08%; ESI-MS: m/z = 335 (M + 1)+.
Methyl 2-ethyl-1,4-diphenyl-1H-pyrrole-3-carboxylate (5aa). White solid, mp 100–101 °C; 1H NMR (CDCl3, 500 MHz) δ 1.10 (t, J = 7.5 Hz, 3H), 2.86 (q, J = 7.5 Hz, 2H), 3.69 (s, 3H), 6.65 (s, 1H), 7.22–7.26 (m, 1H), 7.32–7.35 (m, 4H), 7.41–7.48 (m, 5H) ppm; 13C NMR (CDCl3, 125 MHz) δ 14.6, 19.2, 50.5, 110.6, 121.3, 126.3, 126.6, 126.8, 127.6, 128.4, 129.1, 129.3, 135.7, 139.2, 143.0, 166.0 ppm.
Acknowledgements
We are grateful for the financial support for this research from the National Natural Science Foundation of China (no. 21272053) and the Nature Science Foundation of Hebei Province (no. B2015205182).
References
-
(a) S. K. Tang, G. A. Baker and H. Zhao, Chem. Soc. Rev., 2012, 41, 4030–4066 RSC;
(b) A. C. Barsanti, C. Chiappe, T. Ghilardi and C. S. Pomelli, RSC Adv., 2014, 4, 38848–38854 RSC;
(c) M. Atanassova, V. Kurteva, L. Lubenov and I. Billard, RSC Adv., 2014, 4, 38820–38829 RSC;
(d) A. Rout, E. R. Souza and K. Binnemans, RSC Adv., 2014, 4, 11899–11906 RSC.
-
(a) P. Pollet, E. A. Davey, E. E. Urena-Benavides, C. A. Eckert and C. L. Liotta, Green Chem., 2014, 16, 1034–1055 RSC;
(b) D. F. Izquierdo, J. M. Bernal, M. I. Burguete, E. Garcia-Verdugo, P. Lozano and S. V. Luis, RSC Adv., 2013, 3, 13123–13126 RSC.
-
(a) A. K. Bagdi and A. Hajra, RSC Adv., 2014, 4, 23287–23291 RSC;
(b) S. Majumdar, J. De, A. Chakraborty and D. K. Maiti, RSC Adv., 2014, 4, 24544–24550 RSC;
(c) J. D. Patil and D. M. Pore, RSC Adv., 2014, 4, 14314–14319 RSC;
(d) H. Veisi, A. A. Manesh, N. Khankhani and R. Ghorbani-Vaghei, RSC Adv., 2014, 4, 25057–25062 RSC.
-
(a) S. Steudte, S. Bemowsky, M. Mahrova, U. Bottin-Weber, E. Tojo-Suarez, P. Stepnowski and S. Stolte, RSC Adv., 2014, 4, 5198–5205 RSC;
(b) G. Cevasco and C. Chiappe, Green Chem., 2014, 16, 2375–2385 RSC.
-
(a) A. P. Abbott, G. Capper, D. L. Davies, R. K. Rasheed and V. Tambyrajah, Chem. Commun., 2003, 70–71 RSC;
(b) A. P. Abbott, D. Boothby, G. Capper, D. L. Davies and R. K. Rasheed, J. Am. Chem. Soc., 2004, 126, 9142–9147 CrossRef CAS PubMed.
-
(a) G. Imperato, E. Eibler, J. Niedermaier and B. König, Chem. Commun., 2005, 1170–1172 RSC;
(b) G. Imperato, S. Hoger, D. Lenoir and B. König, Green Chem., 2006, 8, 1051–1055 RSC.
- Y. T. Dai, J. van Spronsen, G. J. Witkamp, R. Verpoorte and Y. H. Choi, Anal. Chim. Acta, 2013, 766, 61–68 CrossRef CAS PubMed.
- M. Francisco, A. van den Bruinhorst and M. C. Kroon, Angew. Chem., Int. Ed., 2013, 52, 3074–3085 CrossRef CAS PubMed.
- M. A. Skopek, M. A. Mohamoud, K. S. Ryder and A. R. Hillman, Chem. Commun., 2009, 935–937 RSC.
-
(a) Q. H. Zhang, K. De Oliveira Vigier, S. Royer and F. Jérôme, Chem. Soc. Rev., 2012, 41, 7108–7146 RSC;
(b) B. K. Tang and K. H. Row, Monatsh. Chem., 2013, 144, 1427–1454 CrossRef CAS PubMed.
-
(a) A. Paiva, R. Craveiro, I. Aroso, M. Martins, R. L. Reis and A. R. C. Duarte, ACS Sustainable Chem. Eng., 2014, 2, 1063–1071 CrossRef CAS;
(b) C. Russ and B. König, Green Chem., 2012, 14, 2969–2982 RSC;
(c) E. L. Smith, A. P. Abbott and K. S. Ryder, Chem. Rev., 2014, 114, 11060–11082 CrossRef CAS PubMed.
-
(a) F. Pena-Pereira and J. Namiesnik, ChemSusChem, 2014, 7, 1784–1800 CrossRef CAS PubMed;
(b) F. S. Oliveira, A. B. Pereiro, L. P. N. Rebelo and I. M. Marrucho, Green Chem., 2013, 15, 1326–1330 RSC.
- F. del Monte, D. Carriazo, M. C. Serrano, M. C. Gutierrez and M. L. Ferrer, ChemSusChem, 2014, 7, 999–1009 CrossRef CAS PubMed.
- M. Francisco, A. van den Bruinhorst and M. C. Kroon, Green Chem., 2012, 14, 2153–2157 RSC.
-
(a) D. V. Wagle, H. Zhao and G. A. Baker, Acc. Chem. Res., 2014, 47, 2299–2308 CrossRef CAS PubMed;
(b) D. Carriazo, M. C. Serrano, M. C. Gutierrez, M. L. Ferrer and F. del Monte, Chem. Soc. Rev., 2012, 41, 4996–5014 RSC.
-
(a) Z. H. Zhang, X. N. Zhang, L. P. Mo, Y. X. Li and F. P. Ma, Green Chem., 2012, 14, 1502–1506 RSC;
(b) F. P. Ma, G. T. Cheng, Z. G. He and Z. H. Zhang, Aust. J. Chem., 2012, 65, 409–416 CAS;
(c) N. Azizi, E. Batebi, S. Bagherpour and H. Ghafuri, RSC Adv., 2012, 2, 2289–2293 RSC;
(d) N. Azizi and E. Gholibeglo, RSC Adv., 2012, 2, 7413–7416 RSC;
(e) P. H. Li, F. P. Ma, P. Wang and Z. H. Zhang, Chin. J. Chem., 2013, 31, 757–763 CrossRef CAS;
(f) U. B. Patil, A. S. Singh and J. M. Nagarkar, RSC Adv., 2014, 4, 1102–1106 RSC;
(g) A. Rajawat, S. Khandelwal and M. Kumar, RSC Adv., 2014, 4, 5105–5112 RSC;
(h) P. Wang, F. P. Ma and Z. H. Zhang, J. Mol. Liq., 2014, 198, 259–262 CrossRef CAS PubMed;
(i) Y. C. Zhang, F. L. Lu, X. H. Cao and J. Q. Zhao, Rsc Adv., 2014, 4, 40161–40169 RSC;
(j) A. K. Sanap and G. S. Shankarling, Rsc Adv., 2014, 4, 34938–34943 RSC;
(k) J. Lu, X. T. Li, E. Q. Ma, L. P. Mo and Z. H. Zhang, ChemCatChem, 2014, 6, 2854–2859 CrossRef CAS;
(l) A. A. Assanosi, M. M. Farah, J. Wood and B. Al-Duri, RSC Adv., 2014, 4, 39359–39364 RSC;
(m) A. Chaskar, Lett. Org. Chem., 2014, 11, 480–486 CrossRef CAS;
(n) N. Azizi, S. Dezfooli and M. M. Hashemi, C. R. Chim., 2013, 16, 1098–1102 CrossRef CAS PubMed;
(o) N. Azizi, M. Mariami and M. Edrisi, Dyes Pigm., 2014, 100, 215–221 CrossRef CAS PubMed;
(p) N. Azizi, S. Dezfooli and M. M. Hashemi, J. Mol. Liq., 2014, 194, 62–67 CrossRef CAS PubMed;
(q) N. Azizi, Z. Yadollahy and A. Rahimzadeh-Oskooee, Tetrahedron Lett., 2014, 55, 1722–1725 CrossRef CAS PubMed.
- T. S. A. Heugebaert, B. I. Roman and C. V. Stevens, Chem. Soc. Rev., 2012, 41, 5626–5640 RSC.
- A. Al-Mourabit, M. A. Zancanella, S. Tilvi and D. Romo, Nat. Prod. Rep., 2011, 28, 1229–1260 RSC.
- S. Durgamma, A. Muralikrishna, V. Padmavathi and A. Padmaja, Med. Chem. Res., 2014, 23, 2916–2929 CrossRef CAS.
- M. Takase, N. Yoshida, T. Narita, T. Fujio, T. Nishinaga and M. Iyoda, RSC Adv., 2012, 2, 3221–3224 RSC.
- V. Estevez, M. Villacampa and J. C. Menendez, Chem. Commun., 2013, 49, 591–593 RSC.
- Z. H. Zhang, J. J. Li and T. S. Li, Ultrason. Sonochem., 2008, 15, 673–676 CrossRef CAS PubMed.
- F. P. Ma, P. H. Li, B. L. Li, L. P. Mo, N. Liu, H. J. Kang, Y. N. Liu and Z. H. Zhang, Appl. Catal., A, 2013, 457, 34–41 CrossRef CAS PubMed.
-
(a) V. Estévez, M. Villacampa and J. C. Menéndez, Chem. Soc. Rev., 2014, 43, 4633–4657 RSC;
(b) G. R. Reddy, T. R. Reddy, S. C. Joseph, K. S. Reddy, C. L. T. Meda, A. Kandale, D. Rambabu, G. R. Krishna, C. M. Reddy, K. V. L. Parsa, K. S. Kumar and M. Pal, RSC Adv., 2012, 2, 9142–9150 RSC.
- S. Maiti, S. Biswas and U. Jana, J. Org. Chem., 2010, 75, 1674–1683 CrossRef CAS PubMed.
- A. T. Khan, M. Lal, P. R. Bagdi, R. S. Basha, P. Saravanan and S. Patra, Tetrahedron Lett., 2012, 53, 4145–4150 CrossRef CAS PubMed.
- H. Saeidian, M. Abdoli and R. Salimi, C. R. Chim., 2013, 16, 1063–1070 CrossRef CAS PubMed.
- A. B. Atar and Y. T. Jeong, Tetrahedron Lett., 2013, 54, 5624–5628 CrossRef CAS PubMed.
- B. L. Li, M. Zhang, H. C. Hu, X. Du and Z. H. Zhang, New J. Chem., 2014, 38, 2435–2442 RSC.
- G. R. Reddy, T. R. Reddy, S. C. Joseph, K. S. Reddy and M. Pal, RSC Adv., 2012, 2, 3387–3395 RSC.
- P. R. K. Murthi, D. Rambabu, M. V. B. Rao and M. Pal, Tetrahedron Lett., 2014, 55, 507–509 CrossRef PubMed.
- P. R. Bagdi, R. S. Basha, M. Lal and A. T. Khan, Chem. Lett., 2013, 42, 939–941 CrossRef CAS.
- H. M. Meshram, B. M. Babu, G. S. Kumar, P. B. Thakur and V. M. Bangade, Tetrahedron Lett., 2013, 54, 2296–2302 CrossRef CAS PubMed.
- J. B. Bharate, R. Sharma, S. Aravinda, V. K. Gupta, B. Singh, S. B. Bharate and R. A. Vishwakarma, RSC Adv., 2013, 3, 21736–21742 RSC.
- B. L. Li, P. H. Li, X. N. Fang, C. X. Li, J. L. Sun, L. P. Mo and Z. H. Zhang, Tetrahedron, 2013, 69, 7011–7018 CrossRef CAS PubMed.
- N. Gupta, K. N. Singh and J. Singh, J. Mol. Liq., 2014, 199, 470–473 CrossRef CAS PubMed.
-
(a) J. T. Hou, J. W. Gao and Z. H. Zhang, Appl. Organomet. Chem., 2011, 25, 47–53 CrossRef CAS;
(b) J. Deng, L. P. Mo, F. Y. Zhao, Z. H. Zhang and S. X. Liu, ACS Comb. Sci., 2012, 14, 335–341 CrossRef CAS PubMed;
(c) R. Y. Guo, P. Wang, G. D. Wang, L. P. Mo and Z. H. Zhang, Tetrahedron, 2013, 69, 2056–2061 CrossRef CAS PubMed;
(d) R. Y. Guo, Z. M. An, L. P. Mo, R. Z. Wang, H. X. Liu, S. X. Wang and Z. H. Zhang, ACS Comb. Sci., 2013, 15, 557–563 CrossRef CAS PubMed;
(e) R. Y. Guo, Z. M. An, L. P. Mo, S. T. Yang, H. X. Liu, S. X. Wang and Z. H. Zhang, Tetrahedron, 2013, 69, 9931–9938 CrossRef CAS PubMed;
(f) B. L. Li, H. C. Hu, L. P. Mo and Z. H. Zhang, RSC Adv., 2014, 4, 12929–12943 RSC;
(g) X. T. Li, A. D. Zhao, L. P. Mo and Z. H. Zhang, RSC Adv., 2014, 4, 51580–51588 RSC;
(h) X. N. Zhao, G. F. Hu, M. Tang, T. T. Shi, X. L. Guo, T. T. Li and Z. H. Zhang, RSC Adv., 2014, 4, 51089–51097 RSC.
-
(a) J. Deng, L. P. Mo, F. Y. Zhao, L. L. Hou, L. Yang and Z. H. Zhang, Green Chem., 2011, 13, 2576–2584 RSC;
(b) Y. H. Liu, J. Deng, J. W. Gao and Z. H. Zhang, Adv. Synth. Catal., 2012, 354, 441–447 CrossRef CAS;
(c) P. H. Li, B. L. Li, Z. M. An, L. P. Mo, Z. S. Cui and Z. H. Zhang, Adv. Synth. Catal., 2013, 355, 2952–2959 CrossRef CAS;
(d) X. N. Zhao, H. C. Hu, F. J. Zhang and Z. H. Zhang, Appl. Catal., A, 2014, 482, 258–265 CrossRef CAS PubMed;
(e) P. H. Li, B. L. Li, H. C. Hu, X. N. Zhao and Z. H. Zhang, Catal. Commun., 2014, 46, 118–122 CrossRef CAS PubMed.
- S. Handy and K. Lavender, Tetrahedron Lett., 2013, 54, 4377–4379 CrossRef CAS PubMed.
Footnote |
† Electronic supplementary information (ESI) available. CCDC 1008451. For ESI and crystallographic data in CIF or other electronic format see DOI: 10.1039/c4ra13577f |
|
This journal is © The Royal Society of Chemistry 2015 |
Click here to see how this site uses Cookies. View our privacy policy here.