DOI:
10.1039/C6RA01931E
(Paper)
RSC Adv., 2016,
6, 25689-25694
White-light emitting materials with tunable luminescence based on steady Eu(III) doping of Tb(III) metal–organic frameworks†
Received
22nd January 2016
, Accepted 25th February 2016
First published on 26th February 2016
Abstract
A sequence of isostructural lanthanide metal–organic frameworks, namely [Ln(Hbtca)(phen)] (Ln = Tb (1) and Eu (2), H4btca = biphenyl-2,3,3′,5′-tetracarboxylic acid and phen = 1,10-phenanthroline), has been successfully synthesized under a hydrothermal reaction. The single X-ray diffraction studies reveal that the structure of the Ln-MOFs is a double-paned 2D network. Thermogravimetric analysis has been used to characterise the Ln-MOFs, which display good thermal stability. By adjusting the ratio of Eu3+ to Tb3+, a series of co-doped lanthanide coordination polymers [EuxTb1−x(Hbtca) (phen)] (0 ≤ x ≤ 1) has been successfully acquired, with emission colors changes from an initial red, to yellow and lastly green. Excitingly, a white emission material has been successfully obtained with the ratio Eu0.0167Tb0.9833-MOF and the CIE coordinates (0.3199, 0.3381), these values are very close to the standardized values (0.3333, 0.3333).
Introduction
Recently, multicolor luminescent materials, especially those with white-light emission have gained considerable attention due to their wide applications in color-tunable phosphors,1 full-color displays,2 and multicolor-light emitting diodes (LEDs).3 In our daily life, solid state white-light emitting materials play an important role because such materials can adjust to specific environments and requirements, an ability that could result in tremendous benefits in lighting, automobiles, communication and medicine.4 In particular, lanthanide metal–organic frameworks (Ln-MOFs), as one kind of solid state white-light emitting material, offer numerous advantages such as photoluminescence efficiency, sharp and intense emission peaks and long luminescence lifetimes.5 Ln-MOFs can also emit in the primary color range (blue, green and red) that fully spans the entire visible spectrum,6 and thus can be used in white-light materials. Furthermore, considering that Ln-MOFs are usually isostructural and possess excellent spatial regularity, it is possible to precisely obtain multicolor and white emission materials via co-doping different lanthanide ions into the same framework along with varying the excitation wavelength.7 There are a great deal of approaches to obtain white-light emitting materials, such as monochromatic, dichromatic (blue and yellow),8 trichromatic (blue, green and red)7a,9 and tetrachromatic.10 Although so many approaches exist, it is still a challenge to target white-light emitting MOF materials because dichromatic and trichromatic materials should be compensated exactly.11 In this work, a series of isostructural Ln-MOFs [TbxEu1−x(Hbtca)(phen)] has been successfully synthesized by incorporating H4btca and phen ligands with LnCl3·6H2O (Ln = Eu, Tb) under hydrothermal reactions. The H4btca ligand was selected for more carboxylic acid sites and blue fluorescence emission. By adjusting the co-doping ratio of Eu3+ to Tb3+, a white-light emission material [Eu0.0167Tb0.9833(Hbtca)(phen)] has been successfully obtained with a ratio of 1
:
59. The white-light emitting MOF is sat at (0.3199, 0.3381) for the CIE coordinates upon excitation at 360 nm, these values are very close to the standardized values of (0.3333, 0.3333) according to the 1931 CIE coordinate diagram.12
Experimental
Materials and measurements
All the chemicals were used as commercially obtained without further purification. Elemental analyses of C, H and N were carried out on a Perkin-Elmer 2400 elemental analyzer. The FT-IR spectra using the KBr pellet method were measured using a Perkin-Elmer Spectrum Two FT-IR spectrometer (4000 to 400 cm−1). TGA data were recorded with a NETZSCH STA409pc under a heating rate of 10 °C min−1 from 20 to 1000 °C under nitrogen protection. X-ray diffraction (XRD) patterns were measured on an EMPYREAN PANALYTICAL apparatus and recorded on crushed single crystals over the range 5–80° using Cu-Kα radiation. Inductively coupled plasma spectroscopy (ICP) was performed on an Ultima 2 spectrometer. The fluorescence excitation and emission spectra were recorded at room temperature with an Edinburgh FLS920 spectrophotometer equipped with a 150 W xenon lamp as the excitation source. The luminescence decay curves were examined using an Edinburgh FLS920 spectrophotometer. The Commission International de I’Eclairage (CIE) color coordinates were calculated on the basis of the international CIE standards.13
X-ray crystallography
Crystallographic data were collected at a temperature of 293(2) K on an Agilent Xcalibur E X-ray single crystal diffractometer with graphite-monochromatic Cu-Kα radiation. The empirical absorption correction was carried out by a SADABS program. Using the SHELX-97 program, the structure of the Eu-MOF was solved by direct methods and refined anisotropically by a full-matrix least squares technique on the F2 values. All hydrogen atoms were added to appropriate positions in theory and refined isotropically using the riding model. The crystallographic data and refinement parameters for Eu-MOF are listed in Table S1.† Selected bond lengths and angles are listed in Table S2.†The CCDC reference number of Eu-MOF is 1441079.
Synthesis of lanthanide compounds 1–6
[Tb(Hbtca)(phen)] (1). H4btca (0.0330 g, 0.1 mmol), TbCl3·6H2O (0.0747 g, 0.2 mmol) and phen (0.0198 g, 0.1 mmol) were added to 8 ml of deionized water. A small amount of nitric acid (40 μl HNO3, 6 M) was used to control the pH of the reaction system, then the above mixture was placed in a sealed 23 ml Teflon-lined stainless vessel, which was heated at 160 °C for 72 h under autogenous pressure and was cooled down to room temperature at 5 °C h−1. Yellow block crystals were obtained. Yield: 0.010 g (7.59%). Anal. calcd for C56H30Tb2N4O16 (%): C, 50.47; H, 2.27; N, 4.20. Found: C, 50.45; H, 2.26; N, 4.20. IR (KBr pellet, cm−1): 1737.12(s), 1690.59(s), 1619.76(vs), 1580.08(vs), 1519.42(s), 1454.71(s), 1426.26(vs), 1390.23(vs), 1104.27(s), 943.90(m), 848.84(s), 836.02(s), 765.76(s), 729.01(s), 707.34(s), 685.40(s), 654.81(m), 540.21(m) and 454.52(m).
[Eu(Hbtca)(phen)] (2). Compound 2 was synthesized under a similar method as described for 1, except that the corresponding EuCl3·6H2O (0.0733 g, 0.2 mmol) reactant was used instead of TbCl3·6H2O. Yield: 0.011 g (8.35%). Anal. calcd for C56H30Eu2N4O16 (%): C, 51.00; H, 2.29; N, 4.25. Found: C, 51.04; H, 2.30; N, 4.27. IR (KBr pellet, cm−1): 1736.92(s), 1690.31(s), 1619.21(vs), 1580.33(vs), 1518.63(s), 1454.08(s), 1426.53(vs), 1388.92(vs), 1103.83(s), 941.88(m), 848.90(s), 835.16(s), 765.24(s), 728.80(s), 706.21(s), 685.23(s), 660.00(m), 539.49(m) and 453.65(m).The mixed-lanthanide compounds 3–6 [EuxTb1−x(Hbtca)(phen)] were synthesized using the same procedure as 1 except using mixtures of EuCl3·6H2O and TbCl3·6H2O with different proportions. The molar ratio of the Ln3+ ions in 3–6 match well with that of the starting materials, as further confirmed by the inductively coupled plasma spectroscopy (ICP) data, which are listed in Table S3.†
[Eu0.0167Tb0.9833(Hbtca)(phen)] (3). The corresponding ratio of Eu3+ and Tb3+ ions was 1
:
59 (EuCl3·6H2O: 0.0012 g, TbCl3·6H2O: 0.0735 g). Yield: 0.012 g (9.10%). Anal. calcd for C56H30(Eu0.0167Tb0.9833)2N4O16 (%): C, 50.48; H, 2.27; N, 4.20. Found: C, 50.50; H, 2.27; N, 4.20. IR (KBr pellet, cm−1): 1736.78(s), 1691.43(s), 1619.23(vs), 1610.75(s), 1577.17(vs), 1519.12(s), 1454.23(s), 1426.10(vs), 1386.85(vs), 1103.98(s), 942.46(m), 848.64(s), 836.24(s), 765.20(s), 728.63(s), 706.74(s), 685.24(s), 654.26(m), 539.19(m) and 453.72(m).
[Eu0.025Tb0.975(Hbtca)(phen)] (4). The corresponding ratio of Eu3+ and Tb3+ ions was 1
:
39 (EuCl3·6H2O: 0.0018 g, TbCl3·6H2O: 0.0728 g). Yield: 0.011 g (8.35%). Anal. calcd for C56H30(Eu0.025Tb0.975)2N4O16 (%): C, 50.48; H, 2.27; N, 4.21. Found: C, 50.54; H, 2.28; N, 4.23. IR (KBr pellet, cm−1): 1736.20(s), 1691.81(s), 1619.17(vs), 1609.88(s), 1576.62(vs), 1519.37(s), 1453.95(s), 1426.19(vs), 1387.23(vs), 1103.96(s), 942.19(m), 848.63(s), 836.22(s), 765.20(s), 728.67(s), 706.74(s), 685.19(s), 654.26(m), 540.44(m) and 453.51(m).
[Eu0.0333Tb0.9667(Hbtca)(phen)] (5). The corresponding ratio of Eu3+ and Tb3+ ions was 1
:
29 (EuCl3·6H2O: 0.0024 g, TbCl3·6H2O: 0.0722 g). Yield: 0.010 g (7.59%). Anal. calcd for C56H30(Eu0.0333Tb0.9667)2N4O16 (%): C, 50.48; H, 2.27; N, 4.21. Found: C, 50.62; H, 2.29; N, 4.24. IR (KBr pellet, cm−1): 1736.41(s), 1691.96(s), 1618.94(vs), 1610.01(s), 1576.48(vs), 1519.52(s), 1453.81(s), 1426.22(vs), 1387.60(vs), 1104.13(s), 942.40(m), 848.75(s), 837.19(s), 765.21(s), 728.86(s), 706.86(s), 685.25(s), 654.78(m), 540.74(m) and 453.59(m).
[Eu0.05Tb0.95(Hbtca)(phen)] (6). The corresponding ratio of Eu3+ and Tb3+ ions was 1
:
19 (EuCl3·6H2O: 0.0037 g, TbCl3·6H2O: 0.0709 g). Yield: 0.010 g (7.59%). Anal. calcd for C56H30(Eu0.05Tb0.95)2N4O16 (%): C, 50.50; H, 2.27; N, 4.21. Found: C, 50.76; H, 2.30; N, 4.26. IR (KBr pellet, cm−1): 1736.30(s), 1691.89(s), 1619.15(vs), 1610.11(s), 1572.00(vs), 1519.56(s), 1453.81(s), 1426.31(vs), 1388.31(vs), 1104.20(s), 942.30(m), 848.66(s), 836.72(s), 765.22(s), 728.94(s), 706.84(s), 685.27(s), 654.78(m), 540.20(m) and 453.66(m).
Results and discussion
The powder XRD patterns obtained at room temperature are shown in Fig. 1. The peak positions of compound 1 are in agreement with compound 2, this confirms that compounds 1 and 2 are isomorphic. Subsequently, a series of co-doped lanthanide coordination polymers [EuxTb1−x(Hbtca)(phen)] (0 ≤ x ≤ 1) has been designed and successfully acquired by adjusting the feed ratio of Eu3+ to Tb3+. The powder X-ray diffraction analysis confirms that compounds 1–6 are isomorphic (shown in Fig. 1).
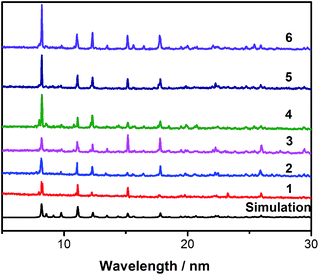 |
| Fig. 1 The simulated pattern (bottom, black) and experimental powder XRD patterns for compounds 1–6. | |
The IR spectra of 1–6 are similar and they have almost the same positions for the absorption peaks (Fig. S3†). The TGA analyses of 1, 2 and the doped compound 3 were carried out, as shown in Fig. 2. The curves are similar, due to the absence of solvent water molecules in the structure, and the TGA curves display a one-step weight loss with a stable plateau until about 500 °C (Fig. 2). This thermally stable temperature is very high, and surpasses most of the reported values for MOFs.14
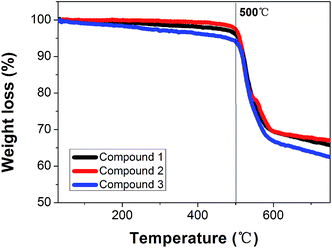 |
| Fig. 2 The TGA curves for compounds 1–3. | |
Structure description
The X-ray diffraction analysis reveals that all the compounds are isomorphic, herein, compound 2 is selected as a representative to describe. The single-crystal X-ray diffraction analysis reveals that compound 2 crystallizes in the triclinic system and space group P
. The asymmetry unit contains two crystallographically independent Eu3+ ion centers, two anion Hbtca3− ligands and two phen ligands (Fig. 3). Both the Eu1 and Eu2 centers are nine-coordinated by seven carboxylate-oxygen atoms from five Hbtca3− ligands and two nitrogen atoms from a chelating phen ligand, and display a distorted tricapped trigonal prism geometry configuration. Two adjacent crystallographically equivalent Eu(III) atoms are connected by two bidentate bridging ligands and two tridentate chelating carboxylate groups from four independent Hbtca3− ligands to generate a binuclear unit Eu2(OCO)6, the distance between the two equivalent Eu3+ centers is 3.87(11) Å.
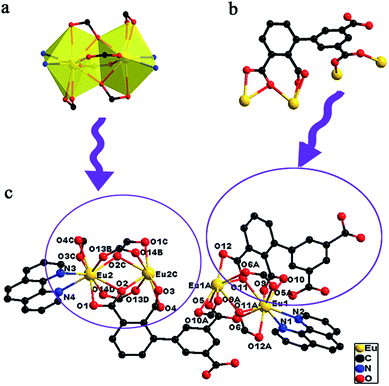 |
| Fig. 3 (a) View of the dimeric unit coordination environment of the Eu3+ ions in 2. (b) The coordination modes of the Hbtca3− ligands. (c) The coordination environment of the central metal atoms in 2. Symmetry codes for the atoms generated: A: −x, −y + 2, −z + 2; B: x, y − 1, z; C: −x, −y + 1, −z + 1; D: −x, −y + 2, −z + 1 (hydrogen atoms are omitted for clarity). | |
Four binuclear units are connected with each other through Hbtca3− ligands forming a closed loop, and two phen groups are loaded in the middle of the loop face-to-face, the centroid-to-centroid distance between the central arene rings of the phen groups is 3.5 Å, which is within the scope of distances typically considered for parallel displaced π–π stacking (3.3–3.8 Å)15 (Fig. 4b). The closed loops are further alternately connected through bridging Hbtca3− ligands to fabricate an interesting double-paned extended 2D network structure (Fig. 4). Along the crystallographic b axis, the framework also contains helical tubes, that are constructed from Eu2(OCO)6 dimeric units bridged by Hbtca3− ligands which serve as the helical tubular walls and the size of the channel is approximately 5.5 × 5.5 Å (Fig. 4d). Viewed from the b axis, the helical tube structure is shown as a bead-like one-dimensional chain containing cylinder-shaped open pores, which are represented as gold cylinders. The bond lengths of Eu–O and Eu–N are in the range of 2.368(7)–2.654 (9) Å and 2.537(8)–2.603(11) Å, respectively. The bond angles of O′–Eu–O and O–Eu–N are in the range of 50.7(3)–157.0(3)° and 69.8(3)–144.6(3)°, respectively. The N′–Eu–N bond angle is 64.0(3)° and these values are all comparable to the values reported in another paper.16 The coordination modes of the Hbtca3− ligands are described in Fig. 3c.
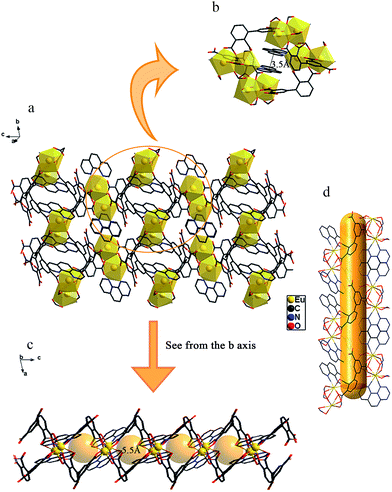 |
| Fig. 4 (a) The polyhedral view of the 2D framework of 2. (b) The closed loop constructed by binuclear units and Hbtca3− ligands and the π–π conjugate coordination of the phen ligands. (c) The bead-like one-dimensional chain viewed from the b axis and (d) the helical tubes of 2 which contain cylinder-shaped open pores. The open pores are represented as gold cylinders. | |
Photoluminescence properties
The solid state fluorescence spectra of compounds 1 and 2, the H4btca ligand and the phen ligand were measured at room temperature and are shown in Fig. 5 and S1.† Upon excitation at 277 nm, the emission of the free H4btca ligands exhibits a broad peak and with a maximum intensity at 352 nm presumably due to the π → π* transitions of the ligand.7b The phen ligand exhibits a fluorescence peak at 439 nm when excited at 370 nm, which belongs to the blue light emission range (400 to 490 nm).17 The characteristic narrow peaks of Tb3+ at 492, 547, 587 and 622 nm are attributed to 5D4 → 7DJ (J = 6–3) transitions, of which the 5D4 → 7D5 transition centred at 547 nm has the maximum intensity, hence showing green luminescence (510 to 570 nm).18 In addition, compound 2 exhibits five major peaks at 582 nm, 595 nm, 618 nm, 655 nm and 699 nm, corresponding to the 5D0 → 7FJ (J = 0–4) transitions of the Eu3+ ions. The highest peak at 618 nm is due to the transition 5D0 → 7F2, hence showing red luminescence (600 to 720 nm).19 It is worth noting that the ligand-based emission completely disappears in the luminescence spectra of compounds 1 and 2, revealing that the ligand serves as an excellent chromophore for sensitizing the lanthanide centers and that the energy transfer from the ligands to the Ln3+ ions is efficient.9 The lifetime τ of compounds 1 and 2 are 0.20 ms and 1.14 ms, respectively (Fig. S6†). The phenomenon found, that the lifetime of Eu3+ is much longer than Tb3+, is due to the sensitization ability of the ligand to Eu3+ which is stronger than Tb3+. The tendency is further confirmed by the excited state lifetimes in the doped-MOFs, for Tb (0.14 ms) to Eu (1.3 ms) (Fig. S6†). Furthermore, it is worth mentioning that the lifetime of the Eu3+ ion is longer compared with those reported previously.20
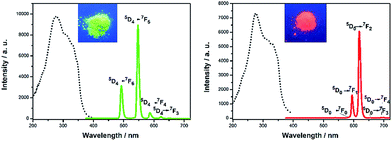 |
| Fig. 5 The excitation and emission spectroscopy data for compounds 1 (a) and 2 (b). | |
The solid state fluorescence emission spectra of the ligands, and compounds 1 and 2 exhibit blue, green and red light emissions, respectively. Because a white-light emission material can be acquired by tuning the ratio of the three primary colors (blue, green and red), a series of different mixed ratio complexes [EuxTb1−x(Hbtca)(phen)] has been synthesized through doping Eu(III) ions and Tb(III) ions into the same framework in an attempt to obtain the white-emission material. The contents of Eu3+ and Tb3+ were matched well with the initial feed ratio and further determined by inductively coupled plasma spectroscopy (ICP), which is listed in Table S3.† As expected, the white-light emission was successfully obtained by adjusting the ion Eu3+/Tb3+ proportion to 0.0167/0.9833 (compound 3) with excitation at 360 nm. The CIE coordinates of 3 are (0.3199, 0.3381) (Fig. 6), these values are very close to the standardized coordinates for pure white light (0.3333, 0.3333). Upon excitation at 360 nm, the emission colors of the other doped-MOFs are close to white. The CIE chromaticity diagram and emission spectra are illustrated in Fig. 7. The CIE coordinates of the co-doped compounds are summarized in Table 1.
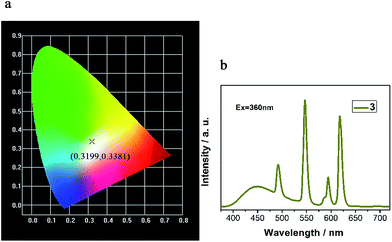 |
| Fig. 6 (a) The CIE chromaticity diagram showing the location of 3 upon excitation at 360 nm. (b) Emission spectrum (λex = 360 nm) of 3. | |
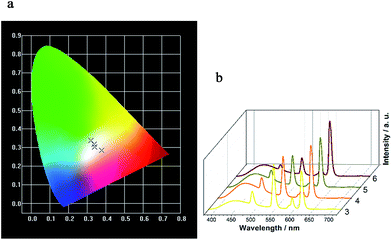 |
| Fig. 7 (a) The CIE chromaticity diagram showing the locations of compounds 3–6 excited at 360 nm. (b) The emission spectra of compounds 3–6 upon excitation at 360 nm. | |
Table 1 The CIE coordinates for compounds 3–6 upon 360 nm excitation
Compound |
CIE coordinates (λex = 360 nm) |
3 |
(0.3779, 0.2858) |
4 |
(0.3409, 0.3024) |
5 |
(0.3377, 0.3164) |
6 |
(0.3199, 0.3381) |
When controlling the molar ratio of Eu3+/Tb3+ and changing the excitation wavelength gradually, the doped compounds 3–6 emit various colors including red, orange, white, yellowish, yellow, kelly green and green, as illustrated in CIE chromaticity diagram (Fig. S5†), tunable full-color can be easily realized.
In the emission of compound 3, the fluorescence peak of free H4btca ligands at 352 nm has disappeared due to the effective energy transfer from the ligands to the Ln3+ ions, while compound 3 exhibits a broad emission centered at 450 nm presumably assigned to the phen ligands which has a red-shift of nearly 10 nm. The slight red-shift may be attributed to the coordination of the ligands to the Ln3+ ions which increases the rigidity of the ligands and thus reduces the loss of energy by radiationless decay of the intraligand emission excited state.21 Furthermore, it is speculated that the chelating ligand phen facilitates the intramolecular energy transfer from the ligands to the lanthanide ions, leading to the enhanced “antenna effect”.14b,22
Conclusions
In summary, we have successfully synthesized isostructural lanthanide metal–organic frameworks by incorporating LnCl3·6H2O (Ln = Eu, Tb) with H4btca as a good chromophore and phen as a chelating ligand. Based on the characteristic emissions of Tb3+ (green) and Eu3+ (red) ions, associated with the blue light emission of the ligands, a white emission material [Eu0.0167Tb0.9833(Hbtca)(phen)] has been successfully obtained. Significantly, the TGA curves prove that the white emission material has a very high thermal stability. The present work provides a promising bi-component approach to the design of tunable color and solid state white-light emission MOF materials, which will probably develop their applications in color displays, labeling and imaging in the future.
Acknowledgements
We are grateful for the financial support provided by the NSFC of China (21361016) and the Inner Mongolia Autonomous Region Fund for Natural Science (2013ZD09).
Notes and references
-
(a) Y. Wang, H. Li, Y. Feng, H. Zhang, G. Calzaferri and T. Ren, Angew. Chem., 2010, 49, 1434–1438 CrossRef CAS PubMed;
(b) C. M. Granadeiro, R. A. S. Ferreira, P. C. R. Soares-Santos, L. D. Carlos, T. Trindade and H. I. S. Nogueira, J. Mater. Chem., 2010, 20, 3313 RSC;
(c) F. Wang, Y. Han, C. S. Lim, Y. Lu, J. Wang, J. Xu, H. Chen, C. Zhang, M. Hong and X. Liu, Nature, 2010, 463, 1061–1065 CrossRef CAS PubMed.
-
(a) Y. H. Niu, M. S. Liu, J. W. Ka, J. Bardeker, M. T. Zin, R. Schofield, Y. Chi and A. K. Y. Jen, Adv. Mater., 2007, 19, 300–304 CrossRef CAS;
(b) H.-C. Su, H.-F. Chen, F.-C. Fang, C.-C. Liu, C.-C. Wu, K.-T. Wong, Y.-H. Liu and S.-M. Peng, J. Am. Chem. Soc., 2008, 130, 3413–3419 CrossRef CAS PubMed.
-
(a) N. Guo, Y. Huang, H. You, M. Yang, Y. Song, K. Liu and Y. Zheng, Inorg. Chem., 2010, 49, 10907–10913 CrossRef CAS PubMed;
(b) W. Ki and J. Li, J. Am. Chem. Soc., 2008, 130, 8114–8115 CrossRef CAS PubMed.
- E. F. Schubert and J. K. Kim, Science, 2005, 308, 1274–1278 CrossRef CAS PubMed.
-
(a) S. V. Eliseeva and J. C. Bunzli, Chem. Soc. Rev., 2010, 39, 189–227 RSC;
(b) M. D. Allendorf, C. A. Bauer, R. K. Bhakta and R. J. Houk, Chem. Soc. Rev., 2009, 38, 1330–1352 RSC;
(c) L. Armelao, S. Quici, F. Barigelletti, G. Accorsi, G. Bottaro, M. Cavazzini and E. Tondello, Coord. Chem. Rev., 2010, 254, 487–505 CrossRef CAS.
-
(a) J. Rocha, L. D. Carlos, F. A. Paz and D. Ananias, Chem. Soc. Rev., 2011, 40, 926–940 RSC;
(b) K. A. White, D. A. Chengelis, K. A. Gogick, J. Stehman, N. L. Rosi and S. Petoud, J. Am. Chem. Soc., 2009, 131, 18069–18071 CrossRef CAS PubMed;
(c) W. J. Rieter, K. M. L. Taylor, H. An, W. Lin and W. Lin, J. Am. Chem. Soc., 2006, 128, 9024–9025 CrossRef CAS PubMed.
-
(a) H. Zhang, X. Shan, Z. Ma, L. Zhou, M. Zhang, P. Lin, S. Hu, E. Ma, R. Li and S. Du, J. Mater. Chem. C, 2014, 2, 1367 RSC;
(b) F. Zhang, P. Yan, H. Li, X. Zou, G. Hou and G. Li, Dalton Trans., 2014, 43, 12574–12581 RSC.
- S. Sapra, S. Mayilo, T. A. Klar, A. L. Rogach and J. Feldmann, Adv. Mater., 2007, 19, 569–572 CrossRef CAS.
- S. Dang, J.-H. Zhang and Z.-M. Sun, J. Mater. Chem., 2012, 22, 8868 RSC.
-
(a) Z. Y. Mao and D. J. Wang, Inorg. Chem., 2010, 49, 4922–4927 CrossRef CAS PubMed;
(b) C. Giansante, G. Raffy, C. Schäfer, H. Rahma, M.-T. Kao, A. G. L. Olive and A. Del Guerzo, J. Am. Chem. Soc., 2011, 133, 316–325 CrossRef CAS PubMed;
(c) Y. Yang, M. Lowry, C. M. Schowalter, S. O. Fakayode, J. O. Escobedo, X. Xu, H. Zhang, T. J. Jensen, F. R. Fronczek, I. M. Warner and R. M. Strongin, J. Am. Chem. Soc., 2006, 128, 14081–14092 CrossRef CAS PubMed;
(d) Y. Liu, M. Nishiura, Y. Wang and Z. Hou, J. Am. Chem. Soc., 2006, 128, 5592–5593 CrossRef CAS PubMed;
(e) M. J. Bowers Ii, J. R. McBride, M. D. Garrett, J. A. Sammons, A. D. Dukes Iii, M. A. Schreuder, T. L. Watt, A. R. Lupini, S. J. Pennycook and S. J. Rosenthal, J. Am. Chem. Soc., 2009, 131, 5730–5731 CrossRef PubMed;
(f) S.-L. Zhong, R. Xu, L.-F. Zhang, W.-G. Qu, G.-Q. Gao, X.-L. Wu and A.-W. Xu, J. Mater. Chem., 2011, 21, 16574 RSC.
-
(a) S. Huh, S. Jung, Y. Kim, S.-J. Kim and S. Park, Dalton Trans., 2010, 39, 1261–1265 RSC;
(b) K. Liu, H. You, Y. Zheng, G. Jia, Y. Huang, M. Yang, Y. Song, L. Zhang and H. Zhang, Cryst. Growth Des., 2010, 10, 16–19 CrossRef CAS.
- L. I. Braddock, T. J. Podlas and N. Marec, Microchem. J., 1967, 12, 55–77 CrossRef CAS.
- T. Smith and J. Guild, Trans. Opt. Soc., 1931, 33, 73–134 CrossRef.
-
(a) J. Song, Z. Luo, D. K. Britt, H. Furukawa, O. M. Yaghi, K. I. Hardcastle and C. L. Hill, J. Am. Chem. Soc., 2011, 133, 16839–16846 CrossRef CAS PubMed;
(b) S. Zou, Q. Li and S. Du, RSC Adv., 2015, 5, 34936–34941 RSC.
- C. Janiak, J. Chem. Soc., Dalton Trans., 2000, 21, 3885–3896 RSC.
-
(a) W. Xu, Y. Zhou, D. Huang, W. Xiong, M. Su, K. Wang, S. Han and M. Hong, Cryst. Growth Des., 2013, 13, 5420–5432 CrossRef CAS;
(b) J. Ye, J. Zhang, G. Ning, G. Tian, Y. Chen and Y. Wang, Cryst. Growth Des., 2008, 8, 3098–3106 CrossRef CAS.
- X. Wang, Q.-G. Zhai, S.-N. Li, Y.-C. Jiang and M.-C. Hu, Cryst. Growth Des., 2014, 14, 177–188 CAS.
- X. Rao, Q. Huang, X. Yang, Y. Cui, Y. Yang, C. Wu, B. Chen and G. Qian, J. Mater. Chem., 2012, 22, 3210 RSC.
- O. L. Malta, M. A. C. dos Santos, L. C. Thompson and N. K. Ito, J. Lumin., 1996, 69, 77–84 CrossRef CAS.
-
(a) Y.-Q. Sun, J. Zhang and G.-Y. Yang, Chem. Commun., 2006, 4700–4702 RSC;
(b) W.-G. Lu, D.-C. Zhong, L. Jiang and T.-B. Lu, Cryst. Growth Des., 2012, 12, 3675–3683 CrossRef CAS;
(c) A. Ablet, S.-M. Li, W. Cao, X.-J. Zheng, W.-T. Wong and L.-P. Jin, Chem.–Asian J., 2013, 8, 95–100 CrossRef CAS PubMed.
- Y. Cui, Y. Yue, G. Qian and B. Chen, Chem. Rev., 2012, 112, 1126–1162 CrossRef CAS PubMed.
- H.-B. Zhang, Y. Peng, X.-C. Shan, C.-B. Tian, L. Ping and S.-W. Du, Inorg. Chem. Commun., 2011, 14, 1165–1169 CrossRef CAS.
Footnote |
† Electronic supplementary information (ESI) available: Experimental details and additional data. CCDC 1441079. For ESI and crystallographic data in CIF or other electronic format see DOI: 10.1039/c6ra01931e |
|
This journal is © The Royal Society of Chemistry 2016 |
Click here to see how this site uses Cookies. View our privacy policy here.