Editorial Perspectives: 2019 novel coronavirus (SARS-CoV-2): what is its fate in urban water cycle and how can the water research community respond?
Received
18th March 2020
, Accepted 18th March 2020
Background
The recent severe acute respiratory syndrome-coronavirus (SARS-CoV-2), which emerged in China in December 2019, is causing an outbreak of respiratory disease (named COVID-19 disease by the World Health Organization, WHO) and deaths in many parts of the world.1 This virus is a member of the Coronaviridae family, identified in the mid-1960s. Highly pathogenic strains of this family have emerged during the last two decades, including SARS-CoV-2, Middle East respiratory syndrome virus (MERS-CoV, 2012, Saudi Arabia), and severe acute respiratory syndrome virus (SARS-CoV, 2003, China). Coronaviruses, ranging from 60 to 220 nm in size, are enveloped single-stranded RNA viruses with crown-like spikes on their surfaces.2,3 The new SARS-CoV-2 virus is known to spread by person-to-person contact (through respiratory droplets over a short distance) or via fecal–oral routes.4 Because the new virus has already begun to spread worldwide, it is important for water engineers and professionals to understand the nature and fate of coronavirus and effective measures to protect public health.
Does coronavirus enter the urban water cycle?
To our water research community, one particularly important question is the fate of the SARS-CoV-2 virus in the urban water cycle and the risks to public health. Previous studies have shown that coronaviruses exist and can maintain their viability in sewage and hospital wastewater, originating from the fecal discharge of infected patients.5,6 Growing attention to this issue is also supported by studies highlighting the persistence of these viruses in aquatic environments and wastewater treatment plants.7,8 Therefore, the SARS-CoV-2 virus may already be present in wastewater, although its concentration and viability remain to be confirmed.4 On the other hand, how long this coronavirus can survive and remain infectious once discharged into wastewater is an open question. Studies carried out following the 2003 SARS epidemic have found traces of the virus in sewage, as coronaviruses can survive to a certain extent in the absence of adequate disinfection, multiplying the chance of contagion (Fig. 1).6,8–14
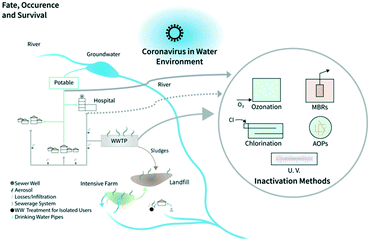 |
| Fig. 1 Coronavirus in water and sanitation systems: fate, occurrence, inactivation methods. | |
The survival of coronaviruses in water depends on a number of factors, including temperature (coronaviruses are very sensitive to temperature), light exposure (solar or UV inactivation), organic matter (viruses can adsorb onto particles of organic matter, affecting settling behavior or light shielding), and the presence of antagonist microorganisms (increasing the extent of inactivation). The continuous recurrence of cases connected with the most aggressive viruses, specifically SARS-CoV in China in 2003 with about 750 deaths, the “avian” H5N1 (highly pathogenic avian influenza) in southeast Asia in 2003, MERS-CoV in Saudi Arabia in 2012 with about 850 deaths, and the Ebola virus disease (EVD) in Guinea, Liberia and Sierra Leone in 2013, highlighted the need for greater information on potential transmission through environmental exposure routes, including water and wastewater exposure pathways.15 Therefore, we recommend a target monitoring program specifically for coronaviruses during water and wastewater treatment to further assess their fate in the urban water cycle. Proper surrogates for SARS-CoV2 may also need to be developed for a regular monitoring program.16
Is coronavirus infectious if present in water?
Existing scientific research on viruses in water and wastewater recycling processes has also produced substantial evidence of the routes of human infection by pathogens in water.12 Because coronaviruses can remain infectious for days in sewage and for longer periods in drinking water, water contaminated by coronaviruses is a potential vehicle for human exposure if aerosols are generated.5,8 For example, during the 2003 SARS outbreak, the aerosolization of water droplets containing coronaviruses from a leaking sewage pipe in a residential apartment resulted in a cluster of cases spread through a community in Hong Kong.5 In addition, coronavirus finds its way into drinking water distribution systems, especially where a lower concentration of residual disinfectant is detected in the system; its viral stability could be maintained by colonizing bacteria in biofilms in distribution systems and it could enter individual homes. There could be risks of aerosolization of coronavirus-containing droplets from shower heads when individuals take showers at home. This aerosol–human transmission pathway is believed to be important for human exposure to Legionella pneumophila from drinking water in residential homes.
How can coronavirus occurrence in wastewater be minimized?
The disinfection steps in both drinking water plants and wastewater treatment plants (WWTPs) and the associated regulatory requirements have been developed to inactivate and kill off a broad spectrum of pathogens. The recent coronavirus outbreak highlights the importance of disinfection to protect public health. For example, based on coronavirus disinfection data from healthcare settings, the U.S. Occupational Safety and Health Administration (OSHA) released its new wastewater worker guidance in February 2020, stating that current disinfection technologies employed in WWTPs, such as oxidation with hypochlorous acid (also known as free chlorine) or peracetic acid, and inactivation by ultraviolet irradiation, are expected to be effective in protecting wastewater workers, as well as the public, from coronavirus.17
Regarding the choices of disinfectant for coronavirus inactivation in water, the use of chlorine still represents the best economic solution.18 However, chlorine reacts with ammonia present in wastewater to form combined chlorine (chloramine), which behaves differently to free chlorine during disinfection. Thus, it is important to understand the chlorine/chloramine speciation and breakpoints specific to the wastewater chemistry for each facility. Furthermore, it is necessary to establish quantitative disinfection kinetics, for example, log inactivation vs. CT values of coronaviruses for traditional disinfectants, including chlorine, chloramine and ozone, and emerging disinfectants, including peracetic acid and hydrogen peroxide. The role of the optimal dose of residual disinfectant is also crucial for drinking water systems.
In WWTPs, membrane bioreactors (MBRs) can also play an important role.19,20 Viruses are generally concentrated in suspended solids that are efficiently removed by filtration mechanisms. In MBRs the retention of suspended solids in the bioreactor, combined with the presence of antagonist microorganisms and adverse chemical–physical conditions, leads to the efficient inactivation of enveloped viruses, such as coronaviruses.19,20 Peracetic acid has been found to have some efficacy against some non-enveloped viruses (e.g. norovirus) that are known to be more resistant than enveloped viruses.13,21 Additional research could provide reassurance of the effectiveness of disinfection processes, specifically against coronaviruses and at lower doses and contact times. Additional studies may also be warranted for disinfectants such as peracetic acid and combined chlorine (chloramine), where there is a lack of coronavirus-specific data or the evidence suggests higher bacterial susceptibility to disinfection compared to viruses.
What are the future research needs?
One research need is to develop new or upgrade existing water and wastewater treatment infrastructure for hot-spots that possibly receive coronavirus from sources including hospitals, community clinics and nursing homes. A fit-for-purpose and decentralized virus inactivation treatment for wastewater discharged from these places will reduce the environmental loading of viruses and secondary transmission. Additional portable disinfection devices for drinking water in individual households will also help minimize waterborne viral infection. For example, light emitting diode (LED)-based UV point-of-use systems may be possible for decentralized disinfection. Furthermore, in places where urine separation infrastructure has been implemented, additional disinfection treatment of urine waste is needed in combination with resource recovery.
Another important research need is a better understanding of the efficacy of emerging disinfection technologies for coronavirus inactivation, especially treatment steps that are integrated into potable water reuse, including UV-based advanced oxidation processes (UV/AOPs) and ozone/biologically activated carbon (O3/BAC). For UV/AOP systems, photo-oxidants including hydrogen peroxide, chlorine, chloramine, persulfate and peracetic acid warrant additional research.22 In addition, regulatory guidelines for virus removal in potable reuse systems need additional review for possible more stringent requirements in the event of a coronavirus outbreak. For example, the state of California currently requires a 12-log removal of viruses during the entire indirect potable treatment train. Additional log-removal credit may be needed to protect public water systems in light of a virus outbreak. Preferential requirements for different viruses may also be needed.
Drinking water distribution systems are another area with research needs. Main distribution system service line pipes and premise plumbing in residential buildings are known to have bacterial colonies and biofilm growth. Whether these biologically active systems also host viruses and affect their viral stability is an open question. Better characterization and monitoring of viral pathogen diversity including the emerging coronavirus in aging drinking water distribution systems will protect the public from possible waterborne infection.
As a preventative measure against the coronavirus outbreak, the public is significantly increasing the use of bactericides, virucides and disinfectants to prevent a possible infection and is limiting travel and activities. This behavior affects our lifestyle and the economy, but from an environmental point of view it will increase the environmental presence of antibiotic-resistant bacteria. This will have indirect impacts on ecosystems and human health, but at the same time reduces global emissions in the atmosphere. Is this sustainable? In the future, we will certainly need to support research and innovation projects that establish integrated and cross-sector approaches for risk-management, combining the research areas of contaminants of emerging concern (CEC), pathogens and antimicrobial resistance genes. The whole water cycle, from the sources of river basins, estuaries and oceans, to wastewater discharge and water reuse, needs to be considered. To address these challenges in a comprehensive way and to develop multidisciplinary and practical solutions for safe drinking water and healthy aquatic environments, research communities of chemists, environmental engineers, microbiologists and public health specialists should work together and create synergistic joint approaches.
It is now clear to all that globalisation also introduces new health risks. When developed countries want to do business internationally, including in developing countries where water and sanitation systems are often insufficient or ineffective, they must take into account the possibility of introducing viruses and epidemics in their cities. Where water and sanitation systems are not adequate, the risk of finding novel viruses is very high. Since it probably is not right to restrict business and people's freedom, do developed countries have a shared responsibility to diffuse an epidemic? In a responsible and ideal scenario, the governments of developed countries must support and finance water and sanitation systems in developing countries, in order to also protect the citizens of their own countries.
Acknowledgements
The authors are grateful for financial support from: i) Sanitary Environmental Engineering Division (SEED) at University of Salerno; ii) Dr. Antonio Buonerba at Inter-University Consortium of Relevant Hazardous (Consorzio inter-Universitario per la previsione e la prevenzione dei Grandi Rischi, C.U.G.RI.); iii) U.S. National Science Foundation Career Program (CBET-1653931).
Notes and references
-
WHO, Coronavirus disease (COVID-2019) situation reports.
-
C. Robinson, M. J. Loeffelholz and B. A. Pinsky, in Clinical Virology Manual, 2016, ch. 19, Respiratory Viruses, pp. 255–276, DOI:10.1128/9781555819156.ch19.
- G. La Rosa, M. Fratini, S. della Libera, M. Iaconelli and M. Muscillo, Emerging and potentially emerging viruses in water environments, Ann. Ist. Super. Sanita, 2012, 48, 397–406 CAS.
-
H. Zhang, Z. Kang, H. Gong, D. Xu, J. Wang, Z. Li, X. Cui, J. Xiao, T. Meng, W. Zhou, J. Liu and H. Xu, The digestive system is a potential route of 2019-nCov infection: a bioinformatics analysis based on single-cell transcriptomes, bioRxiv, 2020, DOI:10.1101/2020.01.30.927806, 2020.2001.2030.927806.
- L. S. Hung, The SARS epidemic in Hong Kong: what lessons have we learned?, J. R. Soc. Med., 2003, 96, 374–378 CrossRef.
- W. K. Leung, K.-f. To, P. K. S. Chan, H. L. Y. Chan, A. K. L. Wu, N. Lee, K. Y. Yuen and J. J. Y. Sung, Enteric involvement of severe acute respiratory syndrome-associated coronavirus infection, Gastroenterology, 2003, 125, 1011–1017 CrossRef.
- T.-T. Fong and E. K. Lipp, Enteric Viruses of Humans and Animals in Aquatic Environments: Health Risks, Detection, and Potential Water Quality Assessment Tools, Microbiol. Mol. Biol. Rev., 2005, 69, 357–371 CrossRef CAS PubMed.
- L. Casanova, W. A. Rutala, D. J. Weber and M. D. Sobsey, Survival of surrogate coronaviruses in water, Water Res., 2009, 43, 1893–1898 CrossRef CAS PubMed.
- K. H. Chan, L. L. L. M. Poon, V. C. C. Cheng, Y. Guan, I. F. N. Hung, J. Kong, L. Y. C. Yam, W. H. Seto, K. Y. Yuen and J. S. M. Peiris, Detection of SARS coronavirus in patients with suspected SARS, Emerging Infect. Dis., 2004, 10, 294–299 CrossRef PubMed.
- M. Jevšnik, A. Steyer, T. Zrim, M. Pokorn, T. Mrvič, Š. Grosek, F. Strle, L. Lusa and M. Petrovec, Detection of human coronaviruses in simultaneously collected stool samples and nasopharyngeal swabs from hospitalized children with acute gastroenteritis, Virol. J., 2013, 10, 46 CrossRef PubMed.
- L. L. M. Poon, K. H. Chan, O. K. Wong, T. K. W. Cheung, I. Ng, B. Zheng, W. H. Seto, K. Y. Yuen, Y. Guan and J. S. M. Peiris, Detection of SARS Coronavirus in Patients with Severe Acute Respiratory Syndrome by Conventional and Real-Time Quantitative Reverse Transcription-PCR Assays, Clin. Chem., 2004, 50, 67–72 CrossRef CAS PubMed.
- B. S. Choudri and Y. Charabi, Health effects associated with wastewater treatment, reuse, and disposal, Water Environ. Res., 2019, 91, 976–983 CrossRef CAS PubMed.
- Y. Ye, R. M. Ellenberg, K. E. Graham and K. R. Wigginton, Survivability, Partitioning, and Recovery of Enveloped Viruses in Untreated Municipal Wastewater, Environ. Sci. Technol., 2016, 50, 5077–5085 CrossRef CAS PubMed.
- K. R. Wigginton, Y. Ye and R. M. Ellenberg, Emerging investigators series: the source and fate of pandemic viruses in the urban water cycle, Environ. Sci.: Water Res. Technol., 2015, 1, 735–746 RSC.
-
S. Chattopadhyay and S. Taft, Exposure Pathways to High-Consequence Pathogens in the Wastewater Collection and Treatment Systems, U.S. Environmental Protection Agency, Cincinnati, OH, 2018 Search PubMed.
-
I. Xagoraraki and E. O'Brien, in Women in Water Quality: Investigations by Prominent Female Engineers, ed. D. J. O'Bannon, Springer International Publishing, Cham, 2020, pp. 75–97, DOI:10.1007/978-3-030-17819-2_5.
-
OSHA, Standards and directives for COVID-19, United States of America Occupational Safety and Health Administration, https://www.osha.gov/SLTC/covid-19/standards.html, 2020 Search PubMed.
- X.-W. Wang, J.-S. Li, M. Jin, B. Zhen, Q.-X. Kong, N. Song, W.-J. Xiao, J. Yin, W. Wei, G.-J. Wang, B.-y. Si, B.-Z. Guo, C. Liu, G.-R. Ou, M.-N. Wang, T.-Y. Fang, F.-H. Chao and J.-W. Li, Study on the resistance of severe acute respiratory syndrome-associated coronavirus, J. Virol. Methods, 2005, 126, 171–177 CrossRef CAS PubMed.
- M. Bodzek, K. Konieczny and M. Ra, Membranes in water and wastewater disinfection : review, Arch. Environ. Prot., 2019, 45, 3–18 CAS.
- R. M. Chaudhry, K. L. Nelson and J. E. Drewes, Mechanisms of Pathogenic Virus Removal in a Full-Scale Membrane Bioreactor, Environ. Sci. Technol., 2015, 49, 2815–2822 CrossRef CAS PubMed.
- P. M. Gundy, C. P. Gerba and I. L. Pepper, Survival of Coronaviruses in Water and Wastewater, Food Environ. Virol., 2009, 1, 10 CrossRef.
- W. Li, T. Jain, K. Ishida and H. Liu, A mechanistic understanding of the degradation of trace organic contaminants by UV/hydrogen peroxide, UV/persulfate and UV/free chlorine for water reuse, Environ. Sci.: Water Res. Technol., 2017, 3, 128–138 RSC.
|
This journal is © The Royal Society of Chemistry 2020 |
Click here to see how this site uses Cookies. View our privacy policy here.