DOI:
10.1039/D3QM01189E
(Research Article)
Mater. Chem. Front., 2024,
8, 1101-1111
Backbone-shape engineering of fused-ring electron-deficient molecular semiconductors for unipolar n-type organic transistors: synthesis, conformation changes, and structure–property correlations†
Received
9th November 2023
, Accepted 15th December 2023
First published on 15th December 2023
Abstract
Backbone-shape engineering is one important strategy towards high-mobility molecular semiconductors. However, it has been seldom employed in modifying fused-ring electron-deficient small molecules, which can provide useful molecular structure–property correlations for developing high-performance n-type organic semiconductors. In this work, we design and synthesize a series of n-type fused-ring small molecules based on ladder-type dithienothiophen-pyrrolobenzothiadiazole (TPBT) derivatives, namely BTPOCl-c, BTPOCl-s, and BTPOCl-w. Due to the different backbone symmetry and length of TPBT derivatives, the above molecules demonstrate three shapes, i.e. C-, S-, and W-backbone shape. Intriguingly, all molecules show a slightly helical conformation between the central cores and end-groups. However, the dihedral angles in the helical conformation are different, which gradually become smaller in the trend of BTPOCl-c < BTPOCl-s < BTPOCl-w. Therefore, changing the backbone shape from C to S and then to W is conducive to enhancing the backbone planarity. Consequently, BTPOCl-w-based unipolar n-type organic transistors display the highest electron mobility among the three molecules. The above result is reasonably explained by BTPOCl-w's deepest energy levels, highest crystallinity with the shortest π–π stacking distance, and larger grain size with fewer grain boundaries. We believe that backbone-shape engineering is a promising approach for developing advanced n-type molecular semiconductors.
1. Introduction
Because of their mechanical flexibility and solution processability, organic π-conjugated small molecules and polymers are potential semiconducting materials for light-weight, large-area, and flexible electronic devices.1–3 The past two decades have witnessed great progress in a variety of electronic devices ranging from organic light emitting diodes,4–6 organic photovoltaics (OPVs),7–10 and organic thin film transistors (OTFTs)11–17 to emerging application fields such as bioelectronics and stretchable electronics.18–20 One of the driving forces to the above remarkable progress is the development of novel high-performance π-conjugated molecular semiconductors.
Among the organic π-conjugated molecules, dye/pigment molecules, such as perylenediimide (PDI), naphthalenediimide (NDI), isoindigo (IID), and diketopyrrolopyrrole (DPP), have been widely used as acceptors to construct n-type molecular semiconductors.21–23 On the other hand, fused-ring electron-deficient small molecules are another important class of n-type molecular semiconductor. In 2016, Zhan and coworkers reported a planar fused-ring electron-deficient small molecule (ICC6IDT-IC) based on indacenodithiophene (IDT) for high-performance OPVs.24 In 2019, Zou and coworkers further substituted the central IDT donor unit with a dithienothiophen-pyrrolobenzothiadiazole (TPBT) electron-deficient unit.25 Although the photovoltaic properties of various TPBT-based small molecules are investigated thoroughly,26–28 they have been less explored in the field of OTFTs. For the rational design of fused-ring electron-deficient small molecules, molecular design strategies of central core modifications,29–31 substitution of electron-withdrawing end groups,32–34 and sidechain engineering35–38 are usually employed. Among these molecular design strategies, molecular backbone-shape engineering has been seldom used. Only a handful of p-type fused-ring molecular semiconductors have been reported, in which the molecular shape has a significant influence on the electronic structures and charge transport properties.39,40 For example, Takimiya et al reported several naphthodithiophene (NDT)-based molecular semiconductors.39 While the linear-shaped molecule NDT1 shows a higher hole mobility of 1.5 cm2 V−1 s−1, its angular-shaped counterpart NDT3 demonstrates a lower mobility of 0.3 cm2 V−1 s−1 (Fig. 1 top). In another instance, Michinobu et al. reported two carbazoledioxazine derivatives with angular and linear shapes. They also found that the linear-shaped small molecule Lin-CZ shows higher mobility than that of the angular-shaped one due to Lin-CZ's denser molecular packing motif (Fig. 1 top).40 Based on the above results, we became interested in modifying TPBT-based fused-ring electron-deficient small molecules by using backbone-shape engineering and further investigate its effect on molecular conformation and charge transport properties.
 |
| Fig. 1 Chemical structures: (top) p-type molecular semiconductors with different backbone shapes; (bottom) fused-ring electron-deficient small molecules with different backbone shapes in this study. | |
Herein, three TPBT-based fused-ring electron-deficient small molecules, namely BTPOCl-c, BTPOCl-s, and BTPOCl-w are designed and synthesized (Fig. 1 bottom). BTPOCl-c shows a C shape, which is also regarded as a banana shape. BTPOCl-s demonstrates an S-shape with one end-group spreading up and the other one down (similar to angular shape). BTPOCl-w exhibits a W-shape with both end-groups spreading up. All three molecules demonstrate a slightly helical conformation between the central ladder-type cores and two end-groups, which is revealed by computational density functional theory (DFT) calculations. However, the dihedral angles in the helical conformation gradually become smaller in the trend of BTPOCl-c < BTPOCl-s < BTPOCl-w, indicating that a higher planarity of the backbone conformation is achieved. This also offers the opportunity to tailor the molecular organizations and electron transport properties in OTFTs. BTPOCl-w shows the highest electron mobility among the three molecules, which is reasonably explained by the deepest LUMO energy levels and highest crystallinity with the shortest π–π stacking distance demonstrated by two dimensional grazing incidence wide-angle X-ray scattering (2D-GIWAXS) patterns and atomic force microscopy (AFM) images. These results indicated that the W-shaped derivative, BTPOCl-w, is a promising n-type molecular semiconductor for further advancing OTFT technology.
2 Results and discussion
2.1 Synthesis and characterization
The synthetic routes to the three different shaped fused-ring small molecules are shown in Scheme 1. The starting compounds 1, 4, and 7 underwent the double intramolecular Cadogan reductive cyclization in the presence of triphenylphosphine in o-dichlorobenzene at a high temperature of 180 °C, followed by the addition of 1-bromo-2-butyloctane under alkaline conditions with potassium carbonate and potassium iodide in DMF at 80 °C, affording the ladder-type fused-ring compounds 2, 5, and 8. By using the Vilsmeier–Haack reaction, the subsequent dialdehyde compounds 3, 6, and 9 can be obtained as orange solids. Finally, the three different shaped fused-ring small molecules BTPOCl-w, BTPOCl-s, and BTPOCl-c were prepared through a Knoevenagel condensation of the compounds 3, 6, and 9, respectively, with 2-(5,6-dichloro-3-oxo-2,3-dihydro-1H-inden-1-ylidene)malononitrile (IC-2Cl) in a high yield of ∼70%. The detailed procedures are summarized in the Experimental section and ESI,† with nuclear magnetic resonance (NMR) spectra and high-resolution mass spectra (MALDI-TOF). All three molecules exhibit good solubility in chloroform, dichloromethane and chlorobenzene, indicating great potential for solution-processable organic electronic devices.41 The thermogravimetry analysis (TGA) shown in Fig. S1 (ESI†) proves the good thermal stability of these electron-deficient small molecules. This is because their thermal decomposition temperatures (Td of 5% weight loss) are all beyond 230 °C, which meets the subsequent OTFT fabrication by using thermal annealing treatment.42
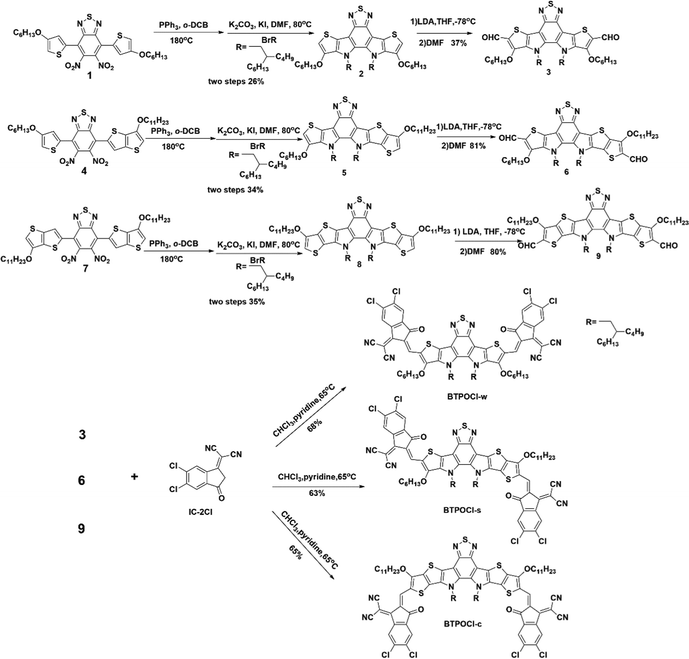 |
| Scheme 1 Synthetic routes to the three different shaped fused-ring small molecules. | |
2.2. Optical and electrochemical properties
The effect of backbone shape is observable in the UV-vis-NIR absorption profiles of the three small molecules. Fig. 2a and b show the UV-vis-NIR absorption spectra of the three different shaped fused-ring small molecules in dilute chloroform solution and thin films with detailed optical data summarized in Table 1. In chloroform solution, all three compounds exhibit similar vibronic shoulders with strong absorption from 600–800 nm. In detail, the maximum absorption peak is slightly red-shifted from 697 nm for BTPOCl-w to 708 nm for BTPOCl-s while the molecule BTPOCl-c shows an even more red-shifted maximum absorption peak at 714 nm. In the thin-film state, the same trend of gradual bathochromic shift is also observed. Interestingly, compared to their absorption in dilute solution, BTPOCl-w demonstrates an obviously enhanced vibration shoulder peak (0–1) compared to those of BTPOCl-c and BTPOCl-s, indicating much enhanced intermolecular π–π interactions in the solid state.43 The above results imply that backbone shape can alter the absorption profile, which can effectively reflect the different molecular packing behavior of the three molecules. Next, electrochemical cyclic voltammetry (CV) was utilized to estimate the energy levels of these molecules in thin films. A platinum disk working electrode, a platinum wire counter electrode, and an Ag/AgCl reference electrode were employed. The three molecule-based films were drop-cast from chloroform solutions on a Pt working electrode (2 mm in diameter). The 0.1 M tetra-n-butylammonium hexafluorophosphate (nBu4NPF6) in acetonitrile was used as the supporting electrolyte solution and thoroughly purged with argon before all CV measurements. The corresponding cyclic voltammograms are plotted in Fig. 2c. The estimated highest occupied molecular orbital (HOMO)/lowest unoccupied molecular orbital (LUMO) levels are listed in Table 1 and displayed in Fig. 2d. Compared to the HOMO/LUMO energy levels of the C-shaped molecule BTPOCl-c (−5.71 eV/−3.69 eV), changing the backbone shape from C to S leads to the downshift of the HOMO and LUMO levels (e.g.BTPOCl-s, EHOMO = −5.77 eV/ELUMO = −3.76 eV). Moreover, this trend is strengthened by altering the backbone shape from S to W. Thus, BTPOCl-w shows the deepest EHOMO of −5.85 eV/ELUMO of −3.82 eV among the three molecules. This result is probably due to the stronger electron-withdrawing ability of the W-shaped molecule than those of C- and S-shaped molecules. The Eeleg values of BTPOCl-c, BTPOCl-s, and BTPOCl-w were determined to be 2.02 eV, 1.99 eV, and 2.03 eV, respectively. This trend is consistent with the UV-vis-NIR results (Table 1). Overall, it implies that controlling the shape of small molecule-based organic semiconductors could facilely tune the absorption and energy levels, which might provide an efficient way to optimize the optoelectronic properties of molecular semiconductors. In this study, the W-shaped small molecule BTPOCl-w shows the deepest LUMO level, which is beneficial for efficient electron carrier transport.
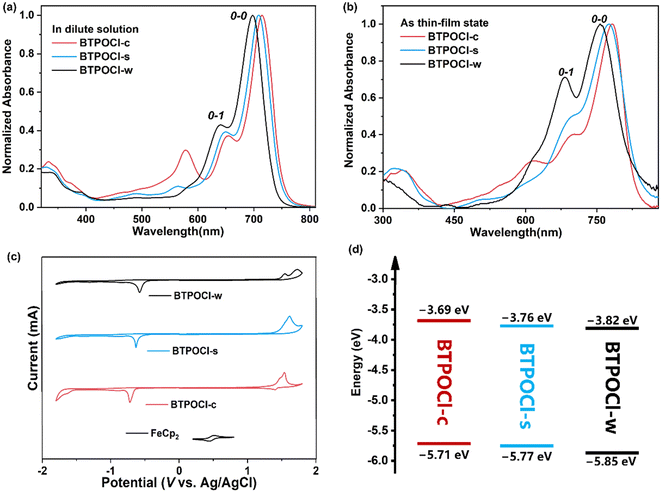 |
| Fig. 2 (a) and (b) Normalized absorption spectra of BTPOCl-c, BTPOCl-s, and BTPOCl-w (a) in dilute chloroform solution and (b) as thin films. (c) The cyclic voltammograms plots of BTPOCl-c, BTPOCl-s, and BTPOCl-w. For calibration, the redox potential of ferrocene (FeCp2) was measured under the same conditions. (d) The diagram of the HOMO/LUMO energy levels of BTPOCl-c, BTPOCl-s, and BTPOCl-w. | |
Table 1 Summary of the optical and electronic properties
Compounds |
λ
sol max
(nm) |
λ
film max
(nm) |
λ
onset
(nm) |
E
optg d (eV) |
HOMOe (eV) |
LUMOe (eV) |
E
eleg f (eV) |
In chloroform solution.
In neat films.
Obtained from the onset wavelength of the absorption of films.
Estimated with the equation: Eoptg = 1240/λonset.
Measured by the cyclic voltammetry (CV) method.
Obtained with the equation: Eeleg = ELUMO − EHOMO.
|
BTPOCl-c
|
714 |
781 |
828 |
1.50 |
−5.71 |
−3.69 |
2.02 |
BTPOCl-s
|
708 |
775 |
831 |
1.49 |
−5.77 |
−3.76 |
1.99 |
BTPOCl-w
|
697 |
756 |
820 |
1.51 |
−5.85 |
−3.82 |
2.03 |
2.3 Conformation control via backbone-shape engineering
To figure out the backbone-shape effects on the molecular conformation of these molecules, density-functional theory (DFT) calculation was conducted at the B3LYP/6-31G(d) level. Methyl groups were reasonably used for N-substituents and alkyl side groups to shorten the calculation time without scarifying the accuracy.44Fig. 3a–c summarizes the top view and side view of the optimized molecular conformations of the three molecules. The torsion angles between the central ladder-type cores and the end-groups are calculated to be 1.9° and 1.5° for BTPOCl-c, 2.1° and 1.5° for BTPOCl-c, and 1.1° and 1.9° for BTPOCl-c. Thus, it reveals that all three molecules have a relative planar conformation because of the S⋯O and H⋯O intramolecular noncovalent interactions.45–47 Interestingly, all three molecules demonstrate a slightly helical conformation between the central ladder-type cores and two IC-2Cl end-groups (see the side view in Fig. 3a–c). However, the dihedral angles in the helical conformation are different, which are calculated to be 9.2° for BTPOCl-c, 5.5° for BTPOCl-s, and 2.3° for BTPOCl-w. This suggests that altering the backbone shape from C to S and then to W is conducive to enhancing planarity.48 As illustrated in Fig. 3d–f, the calculated HOMO/LUMO values are gradually downshifted with altering the backbone shape from C to S and then to W, which is consistent with the CV results. While the HOMO orbitals of all three molecules are mainly located on the ladder-type central cores, the distribution pattern of the LUMO orbitals is different for the three molecules. For the C-shaped BTPOCl-c, it mainly localizes on the ladder-type central core. However, BTPOCl-s shows relatively more distribution at one of the end groups (IC-2Cl). Excitingly, BTPOCl-w's LUMO orbitals widely delocalize on the whole backbone, indicating the most effective LUMO orbital overlapping during the solid state packing. Thus, high charge carrier mobility can be expected for BTPOCl-w.49–51 Furthermore, the electrostatic potential (ESP) distributions of the three small molecules were computed with the corresponding images shown in Fig. 3g–i. The positive ESP values distribute on the most conjugated surfaces of the three molecules (green to blue colored regions), indicating their electron-deficient characteristics. Changing the backbone shape from C to S and then to W, the positive areas (especially the blue colored regions) of ESP distribution are gradually increased, implying that the electron-withdrawing properties of the three molecules are gradually strengthened. In addition, the ESP distributions of BTPOCl-s present an asymmetric ESP distribution owing to its asymmetric chemical structure, which might be beneficial to enhance intermolecular electrostatic interactions.52 Overall, the backbone shape has a large influence on the molecular conformation and corresponding electronic structures and energy levels. Among the three molecules, BTPOCl-w demonstrates the most electron-deficient properties with a very planar backbone conformation, which can facilitate the electron transport in the OTFT devices.53
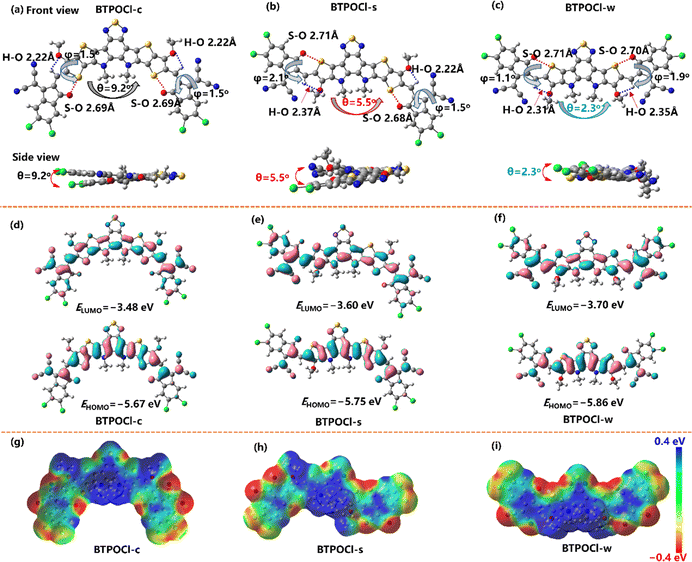 |
| Fig. 3 (a)–(c) DFT optimized geometry of the three small molecules BTPOCl-c (a), BTPOCl-s (b), and BTPOCl-w (c). (d)–(f) The calculated HOMO/LUMO orbitals and energy levels of the three small molecules BTPOCl-c (d), BTPOCl-s (e), and BTPOCl-w (f). (g)–(i) The electrostatic potential (ESP) distributions of the three small molecules BTPOCl-c (g), BTPOCl-s (h), and BTPOCl-w (i). | |
2.4 Unipolar n-type thin-film transistors
Since the three molecules have different shapes and conformations, they should have an influence on the charge-carrier transporting properties (Fig. 4a). Thus, top-gate bottom-contact (TG/BC) OTFTs were fabricated to investigate this effect. The devices were fabricated by spin-coating the small-molecule solutions (5 g L−1 in CHCl3) onto a gold patterned glass substrate to form the organic semiconducting layer. After thermal annealing at 90 °C for 30 min, the dielectric layer was fabricated with a spin-coating process of a 1500 rpm 60 μL polymethyl methacrylate (PMMA) butylacetate solution and annealed at 90 °C for 30 minutes. Finally, a 100 nm Ag layer was deposited on the dielectric layer with a thermal evaporation process as the gate electrode by using a shadow mask. The detailed fabrication procedure is described in the ESI.† As shown in Fig. 4b and Table 2, one could find that the charge-transporting performance is dramatically improved upon altering the backbone shape from C to S and then to W. The C-shape molecule BTPOCl-c exhibits a unipolar electron mobility (μe) of 0.01 cm2 V−1 s−1 and on–off ratio (Ion/Ioff) of ∼103 (Fig. 4c). Changing the C-shaped banana conformation to the asymmetric S conformation leads to a twice growth of μe up to 0.02 cm2 V−1 s−1 (Fig. 4e and Table 2). This enhancement partially resulted from the asymmetric ESP distribution owing to its asymmetric chemical structure, which can strengthen the intermolecular electrostatic interactions. Interestingly, in the case of BTPOCl-w in which the conformation is further changed to W shape, a five-fold increase in the electron charge transport is observed. Thus, BTPOCl-w displays μe of 0.05 cm2 V−1 s−1 with improved Ion/Ioff of ∼104 (Fig. 4g and Table 2). The significantly enhanced μe is due to BTPOCl-w's low-lying LUMO level and very planar backbone conformation, which can facilitate the electron transport in the OTFT devices. Overall, it is remarkable that improving the OTFT device performance can be achieved through small molecules’ backbone-shape engineering, which is correlated to the changes of their HOMO/LUMO energy levels and solid state packing behaviors.
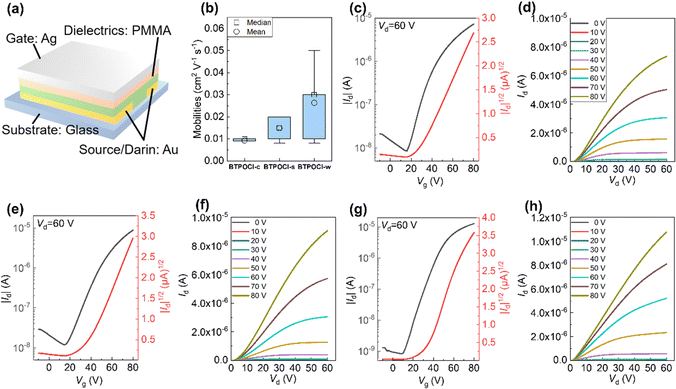 |
| Fig. 4 (a) Top-gate/bottom-contact device configuration used in this study. (b) The electron-mobility comparison profile of the three molecules. (c), (e) and (g) Typical n-type transfer characteristics of the transistors for (c) BTPOCl-c; (e) BTPOCl-s; (g) BTPOCl-w. (d), (f), and (h) Typical n-type output characteristics of the transistors for (d) BTPOCl-c; (f) BTPOCl-s; (h) BTPOCl-w. | |
Table 2 Summary of small-molecule-based OTFT performance
Compounds |
μ
e,max (cm2 V−1 s−1)a |
μ
e,avg (cm2 V−1 s−1)b |
V
th (V) |
I
on/Ioff |
The maximum values of the electron mobilities.
The average values calculated from at least 10 devices.
|
BTPOCl-c
|
0.01 |
0.009 |
24.78 |
103 |
BTPOCl-s
|
0.02 |
0.01 |
29.17 |
104 |
BTPOCl-w
|
0.05 |
0.03 |
30.74 |
104 |
2.5 Characterization of molecular organization and film morphologies
To further investigate the influence of the different molecular conformations on the solid-state packing organization and correlate it with the different OTFT performances, the two-dimensional (2D) grazing incidence wide-angle X-ray scattering (2D-GIWAXS) patterns of the studied three molecules were measured.54 As shown in Fig. 5a–j, all three molecule-based thin films demonstrate multiple diffraction rings, indicating isotropic packing features. However, the out-of-plane (OOP) and in-plane (IP) π–π stacking (010) diffraction peaks of BTPOCl-c are not obvious, suggesting its very weak crystallinity (Fig. 5b and c). Changing the C-shaped banana conformation to the asymmetric S conformation leads to higher intensity and shorter distance of the π–π stacking (3.47 vs. 3.55 Å), indicating that BTPOCl-s has higher crystallinity than that of BTPOCl-c (Fig. 5j and k), which is due to the strengthened intermolecular electrostatic interactions. Intriguingly, the 2D patterns of BTPOCl-w exhibit much stronger diffraction peaks in both the OOP and IP directions, indicating that BTPOCl-w owns the best crystallinity (Fig. 5g, h, and i). Meanwhile, the OOP diffraction peak (010) of BTPOCl-w shows the smallest FWHM (0.145 Å−1) and highest crystalline coherence length (Lc) of 43.3 Å among the three molecules, further indicating its higher thin-film crystallinity (Table 3). Combined with the shortest π–π stacking distance of 3.41 Å, BTPOCl-w demonstrates the largest number of π–π stacking layers of 23.5 according to Scherrer analysis (Table 3). Therefore, it reveals that BTPOCl-w has an excellent long-range order. As shown in the proposed molecular packing models (Fig. 5j–l), changing the backbone conformation from C to S and then to W, the molecules tend to pack more densely and compactly with increased crystallinity and shorter π–π stacking distance, which results from the more planar backbone and increased intermolecular interactions. In addition, atomic force microscopy (AFM) images of BTPOCl-c and BTPOCl-s display smaller grain size, while BTPOCl-w shows larger grain size and fewer grain boundaries (Fig. 6). Overall, with the combination of the highest crystallinity with the shortest π–π stacking distance and larger grain size with fewer grain boundaries, BTPOCl-w demonstrates the highest electron mobility among the three small molecules.
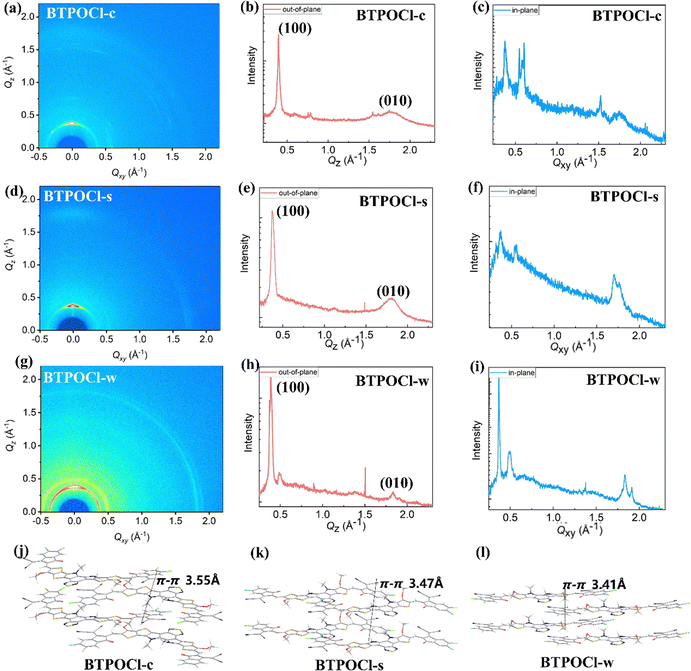 |
| Fig. 5 (a)–(c) 2D GIWAXS patterns and the related 1D profiles in the out-of-plane and in-plane direction based on BTPOCl-c. (d)–(f) 2D GIWAXS patterns and the related 1D profiles in the out-of-plane and in-plane direction based on BTPOCl-s. (g)–(i) 2D GIWAXS patterns and the related 1D profiles in the out-of-plane and in-plane direction based on BTPOCl-w. (j)–(l) Proposed molecular packing models of (j) BTPOCl-c, (k) BTPOCl-s, and (l) BTPOCl-w. | |
Table 3 Crystallographic parameters of small molecules in the out-of-plane direction
Compounds |
q
z [Å−1] |
d
L–L [Å] |
q
xy
[Å−1] |
d
π–π [Å] |
FWHM [Å−1] |
L
c [Å] |
L
c/d |
BTPOCl-c
|
0.396 |
15.9 |
1.771 |
3.55 |
0.461 |
13.6 |
3.8 |
BTPOCl-s
|
0.376 |
16.7 |
1.809 |
3.47 |
0.341 |
18.4 |
5.3 |
BTPOCl-w
|
0.391 |
16.1 |
1.839 |
3.41 |
0.145 |
43.3 |
23.5 |
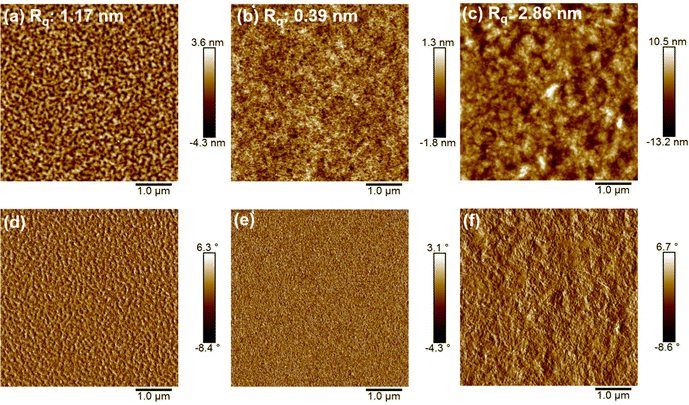 |
| Fig. 6 Tapping-mode atomic force microscope (AFM) topography (a)–(c) and the corresponding phase (d)–(f) images of (a) and (d) BTPOCl-c, (b) and (e) BTPOCl-s, and (c) and (f) BTPOCl-w. | |
3. Conclusions
In summary, backbone-shape engineering is employed to tune the molecular conformation and electron transport properties of the fused-ring electron-deficient small molecules. Thus, three TPBT-based fused-ring electron-deficient small molecules with C-, S-, and W-shaped backbones, namely BTPOCl-c, BTPOCl-s, and BTPOCl-w are designed and synthesized. All three molecules demonstrate a slightly helical conformation between the central ladder-type cores and two IC-2Cl end-groups. However, the dihedral angles in the helical conformation vary significantly, whose value gradually becomes smaller in the trend of BTPOCl-c < BTPOCl-s < BTPOCl-w. It thus suggests that altering the backbone shape from C to S and then to W is conducive to enhancing the planarity of the backbone conformation. This also offers the opportunity to tailor the UV-vis-NIR absorption profiles, HOMO/LUMO energy levels, molecular packing organizations, and charge carrier transporting properties in OTFTs. As a result, BTPOCl-w shows the highest electron mobility among the three molecules, which is reasonably explained by the deepest HOMO/LUMO energy levels, highest crystallinity with shortest π–π stacking distance, and larger grain size with fewer grain boundaries. We believe that backbone-shape engineering will guide the way to developing more promising fused-ring electron-deficient small molecules for high performance OTFTs and OPVs.
4. Experimental details
4.1 Chemical synthesis and characterization
All commercially available solvents, reagents, and chemicals were purchased from Aldrich, TCI and several other reagent companies and used as received without further purification unless otherwise specified. All reactions and manipulations were carried out with the use of a standard inert atmosphere and Schlenk techniques. 1H NMR (400 MHz) and 13C NMR (100 MHz) spectra were measured on a Varian Mercury Plus-400 spectrometer. The following abbreviations were used to explain the multiplicities: s = singlet, d = doublet, t = triplet, and m = multiplet. Deuterated chloroform was used as the solvent. The MALDI-TOF mass spectra were measured by a Bruker autoflex maX MALDI-TOF mass spectrometer. Synthesis of BTPOCl-c was adapted from the published literature.55 The synthetic procedures of the intermediates are described in the ESI.† Synthesis of BTPOCl-w and BTPOCl-s is described as follows.
Synthesis of BTPOCl-w.
To a solution of compound 5 (22.0 mg, 0.0239 mmol) and 2-(5,6-dichloro-3-oxo-2,3-dihydro-1H-inden-1-ylidene)malononitrile (IC-2Cl) (37.8 mg, 0.144 mmol) in dry CHCl3 (10 mL) was added pyridine (1 mL) under N2. The mixture was refluxed for 16 hours and then allowed to cool to room temperature, and then the mixture was poured into CH3OH (100 mL) and filtered, and the residue left in the filter paper was dissolved by CHCl3. After removing the solvent, the residue was purified using column chromatography on silica gel (eluent: n-hexane: CH2Cl2 = 1
:
1, v/v), yielding a dark purple solid (23.0 mg, 68%).1H NMR (400 MHz, CDCl3, ppm): δ = 9.24 (s, 2H), 8.75 (s, 2H), 7.94 (s, 2H), 4.59–4.58 (m, 4H), 4.43(t, 4H, J = 6.0 Hz), 2.12–2.00 (m, 6H), 1.60–1.41 (m, 14H), 1.00–0.66 (m, 48H); 13C NMR (100 MHz, CDCl3, ppm): δ = 185.8, 158.7, 153.2, 147.6, 139.6, 139.4, 139.0, 137.5, 137.4, 136.2, 135.9, 134.8, 128.9, 127.0, 125.2, 120.5, 115.0, 114.9, 114.8, 79.5, 69.4, 54.3, 39.3, 32.0, 31.8, 30.4, 30.1, 29.6, 25.9, 23.0, 22.9, 22.7, 14.3, 14.2, 14.1; MALDI-TOF MS: calcd for C76H82Cl4N8O4S3, 1408.4379; found, 1409.0007.
Synthesis of BTPOCl-s.
To a solution of compound 6 (56.0 mg, 0.0576 mmol) and 2-(5,6-dichloro-3-oxo-2,3-dihydro-1H-inden-1-ylidene)malononitrile (IC-2Cl) (84.6 mg, 0.321 mmol) in dry CHCl3 (10 mL) was added pyridine (1 mL) under N2. The mixture was refluxed for 16 hours and then allowed to cool to room temperature, and then the mixture was poured into CH3OH (100 mL) and filtered, and the residue left on the filter paper was dissolved by CHCl3. After removing the solvent, the residue was purified using column chromatography on silica gel (eluent: n-hexane: CH2Cl2 = 1
:
1, v/v), yielding a dark purple solid (52.0 mg, 63%).1H NMR (400 MHz, CDCl3, ppm): δ = 9.38 (s, 1H), 9.29 (s, 1H), 8.81 (s, 1H), 8.76 (s, 1H), 7.99 (s, 1H), 7.92 (s, 1H), 4.81(t, 2H, J = 6.0 Hz), 4.76–4.75 (m, 2H), 4.59–4.58 (m, 2H), 4.47 (t, 2H, J = 8.0 Hz), 2.15–2.02 (m, 6H), 1.63–1.30 (m, 26H), 1.06–0.67 (m, 46H); 13C NMR (100 MHz, CDCl3, ppm): δ = 186.7, 186.1, 162.6, 158.9, 158.3, 153.7, 147.6, 147.6, 139.5, 139.2, 139.1, 139.0, 138.7, 138.6, 138.4, 137.4, 137.2, 137.1, 136.2, 136.1, 135.1, 133.9, 131.9, 128.8, 128.2, 127.0, 126.8, 125.2, 120.6, 120.2, 118.0, 114.8, 114.6, 113.7, 79.5, 74.5, 69.0, 68.1, 55.6, 55.2, 39.7, 39.2, 32.1, 31.9, 31.8, 31.7, 30.8, 30.4, 30.1, 29.9, 29.8, 29.7, 29.7, 29.6, 29.6, 29.5, 26.0, 25.8, 23.1, 23.1, 23.0, 22.9, 22.9, 22.7, 14.3, 14.3, 14.3, 14.2, 14.0, 14.0; MALDI-TOF MS: calcd for C83H92Cl4N8O4S4, 1534.4849; found, 1535.0177.
Conflicts of interest
There are no conflicts to declare.
Acknowledgements
We are grateful for financial support from the National Natural Science Foundation of China (Grant No. 52203216 and 22375051), and the Natural Science Foundation of Shanghai (21ZR1406900). Y. C. acknowledges the financial support by the Postdoctoral Research Foundation of China (2022M720756 and YJ20210249). The authors thank beamline 14B1 of Shanghai Synchrotron Radiation Facility for providing the beam time and assistance during experiments.
Notes and references
- K. Liu, T. T. Larsen-Olsen, Y. Lin, M. Beliatis, E. Bundgaard, M. Jørgensen, F. C. Krebs and X. Zhan, Roll-coating fabrication of flexible organic solar cells: comparison of fullerene and fullerene-free systems, J. Mater. Chem. A, 2016, 4, 1044–1051 RSC.
- Y. Wang and Y. Liu, Insight into conjugated polymers for organic electrochemical transistors, Trends Chem., 2023, 5, 279–294 CrossRef CAS.
- Y. Bian, Y. Liu and Y. Guo, Intrinsically stretchable organic optoelectronic devices and arrays: progress and perspective, Sci. Bull., 2023, 68, 975–980 CrossRef CAS PubMed.
- G. M. Farinola and R. Ragni, Electroluminescent materials for white organic light emitting diodes, Chem. Soc. Rev., 2011, 40, 3467–3482 RSC.
- J. Song, H. Lee, E. G. Jeong, K. C. Choi and S. Yoo, Organic Light-Emitting Diodes: Pushing Toward the Limits and Beyond, Adv. Mater., 2020, 32, 1907539 CrossRef CAS PubMed.
- M. C. Gather, A. Köhnen and K. Meerholz, White Organic Light-Emitting Diodes, Adv. Mater., 2011, 23, 233–248 CrossRef CAS PubMed.
- T. Kim, J. Choi, H. J. Kim, W. Lee and B. J. Kim, Comparative Study of Thermal Stability, Morphology, and Performance of All-Polymer, Fullerene–Polymer, and Ternary Blend Solar Cells Based on the Same Polymer Donor, Macromolecules, 2017, 50, 6861–6871 CrossRef CAS.
- Y. Wang, Y. Liu, S. Chen, R. Peng and Z. Ge, Significant Enhancement of Polymer Solar Cell Performance via Side-Chain Engineering and Simple Solvent Treatment, Chem. Mater., 2013, 25, 3196–3204 CrossRef CAS.
- H. Yao and J. Hou, Recent Advances in Single-Junction Organic Solar Cells, Angew. Chem., Int. Ed., 2022, 61, e202209021 CrossRef CAS.
- Y. Liang, D. Zhang, Z. Wu, T. Jia, L. Lüer, H. Tang, L. Hong, J. Zhang, K. Zhang, C. J. Brabec, N. Li and F. Huang, Organic solar cells using oligomer acceptors for improved stability and efficiency, Nat. Energy, 2022, 7, 1180–1190 CrossRef CAS.
- H. H. Choi, J. Y. Baek, E. Song, B. Kang, K. Cho, S. Kwon and Y. Kim, A Pseudo-Regular Alternating Conjugated Copolymer Using an Asymmetric Monomer: A High-Mobility Organic Transistor in Nonchlorinated Solvents, Adv. Mater., 2015, 27, 3626–3631 CrossRef CAS PubMed.
- K. Yang, Z. Chen, Y. Wang and X. Guo, Alkoxy-Functionalized Bithiophene/thiazoles: Versatile Building Blocks for High-Performance Organic and Polymeric Semiconductors, Acc. Mater. Res., 2023, 4, 237–250 CrossRef CAS.
- Z. Yi, S. Wang and Y. Liu, Design of High-Mobility Diketopyrrolopyrrole-Based π-Conjugated Copolymers for Organic Thin-Film Transistors, Adv. Mater., 2015, 27, 3589–3606 CrossRef CAS PubMed.
- Y. Wang, R. Hosokawa, T. Mori and T. Michinobu, Polarity Engineering of Benzobisthiadiazole-Based Polymer Thin Film Transistors by Variation of Electron Affinity of the Comonomers, Bull. Chem. Soc. Jpn., 2017, 90, 1041–1049 CrossRef CAS.
- T. Shen, W. Li, Y. Zhao, Y. Wang and Y. Liu, A Hybrid Acceptor-Modulation Strategy: Fluorinated Triple-Acceptor Architecture for Significant Enhancement of Electron Transport in High-Performance Unipolar n-Type Organic Transistors, Adv. Mater., 2023, 35, 2210093 CrossRef CAS PubMed.
- M.-H. Lee, J. Kim, M. Kang, J. Kim, B. Kang, H. Hwang, K. Cho and D.-Y. Kim, Precise Side-Chain Engineering of Thienylenevinylene-Benzotriazole-Based Conjugated Polymers with Coplanar Backbone for Organic Field Effect Transistors and CMOS-like Inverters, ACS Appl. Mater. Interfaces, 2017, 9, 2758–2766 CrossRef CAS PubMed.
- X. Guo, A. Facchetti and T. J. Marks, Imide- and amide-functionalized polymer semiconductors, Chem. Rev., 2014, 114, 8943–9021 CrossRef CAS.
- H. Piwonski, W. Li, Y. Wang, T. Michinobu and S. Habuchi, Improved Fluorescence and Brightness of Near-Infrared and Shortwave Infrared Emitting Polymer Dots for Bioimaging Applications, ACS App. Polym. Mater., 2019, 2, 569–577 CrossRef.
- H. Piwonski, Y. Wang, W. Li, T. Michinobu and S. Habuchi, Millimeter-Deep Detection of Single Shortwave-Infrared-Emitting Polymer Dots through Turbid Media, Nano Lett., 2020, 20, 8803–8810 CrossRef CAS PubMed.
- J. Chen, W. Huang, D. Zheng, Z. Xie, X. Zhuang, D. Zhao, Y. Chen, N. Su, H. Chen, R. M. Pankow, Z. Gao, J. Yu, X. Guo, Y. Cheng, J. Strzalka, X. Yu, T. J. Marks and A. Facchetti, Highly stretchable organic electrochemical transistors with strain-resistant performance, Nat. Mater., 2022, 21, 564–571 CrossRef CAS PubMed.
- Y. H. Zhao, W. Li, T. Shen, Y. Zhao, Y. Liu and Y. Wang, The marriage of dual-acceptor strategy and C-H activation polymerization: naphthalene diimide-based n-type polymers with adjustable molar mass and decent performance, Sci. China: Chem., 2023, 66, 548–561 CrossRef CAS.
- B. Li, X. Zou, M. Xiong, Q. Li, X. Kang, Y. Mu, J. Wang, J. Pei, C. Yang, Z. Lan and X. Wan, Thiazoloisoindigo-based ambipolar polymers for excellent balanced hole and electron mobility, Mater. Chem. Front., 2022, 6, 3369–3381 RSC.
- S. Kang, M. Han, Y. Cho, J. Hong, S. Heo, S. Jeong, Y. Noh and C. Yang, Understanding of copolymers containing pyridine and selenophene simultaneously and their polarity conversion in transistors, Mater. Chem. Front., 2020, 4, 3567–3577 RSC.
- Y. Lin, Q. He, F. Zhao, L. Huo, J. Mai, X. Lu, C.-J. Su, T. Li, J. Wang, J. Zhu, Y. Sun, C. Wang and X. Zhan, A Facile Planar Fused-Ring Electron Acceptor for As-Cast Polymer Solar Cells with 8.71% Efficiency, J. Am. Chem. Soc., 2016, 138, 2973–2976 CrossRef CAS PubMed.
- J. Yuan, Y. Zhang, L. Zhou, G. Zhang, H.-L. Yip, T.-K. Lau, X. Lu, C. Zhu, H. Peng, P. A. Johnson, M. Leclerc, Y. Cao, J. Ulanski, Y. Li and Y. Zou, Single-Junction Organic Solar Cell with over 15% Efficiency Using Fused-Ring Acceptor with Electron-Deficient Core, Joule, 2023, 3, 1140–1151 CrossRef.
- M. Kataria, H. D. Chau, N. Y. Kwon, S. H. Park, M. J. Cho and D. H. Choi, Y-Series-Based Polymer Acceptors for High-Performance All-Polymer Solar Cells in Binary and Non-binary Systems, ACS Energy Lett., 2022, 7, 3835–3854 CrossRef CAS.
- Y. Cui, P. Zhu, X. Shi, X. Liao and Y. Chen, Theoretical Study of Excited State Charge Transfer Characteristics based on A-D-A and A-DA’D-A Type Nonfullerene Acceptors, J. Phys. Chem. C, 2021, 125, 10250–10259 CrossRef CAS.
- F. Feng, P. Wang, Y. Li and X. Bao, Versatile π-bridges in nonfullerene electron acceptors of organic solar cells, Mater. Chem. Front., 2023, 7, 3855–3878 RSC.
- J. Zhao, C. Yao, M. U. Ali, J. Miao and H. Meng, Recent advances in high-performance organic solar cells enabled by acceptor-donor-acceptor-donor-acceptor (A–DA′D–A) type acceptors, Mater. Chem. Front., 2020, 4, 3487–3504 RSC.
- B. Zhang, J. Li, A. Tang, Y. Geng, Q. Guo and E. Zhou, Utilizing Benzotriazole-Fused DAD-Type Heptacyclic Ring to Construct n-Type Polymer for All-Polymer Solar Cell Application, ACS Appl. Energy Mater., 2021, 4, 4217–4223 CrossRef CAS.
- T. Duan, W. Feng, Y. Li, Z. Li, Z. Zhang, H. Liang, H. Chen, C. Zhong, S. Jeong, C. Yang, S. Chen, S. Lu, O. A. Rakitin, C. Li, X. Wan, B. Kan and Y. Chen, Electronic Configuration Tuning of Centrally Extended Non-Fullerene Acceptors Enabling Organic Solar Cells with Efficiency Approaching 19%, Angew. Chem., Int. Ed., 2023, 62, e202308832 CrossRef CAS PubMed.
- G. Li, L. Feng, S. Mukherjee, L. O. Jones, R. M. Jacobberger, W. Huang, R. M. Young, R. M. Pankow, W. Zhu, N. Lu, K. L. Kohlstedt, V. K. Sangwan, M. R. Wasielewski, M. C. Hersam, G. C. Schatz, D. M. DeLongchamp, A. Facchetti and T. J. Marks, Non-fullerene acceptors with direct and indirect hexa-fluorination afford >17% efficiency in polymer solar cells, Energy Environ. Sci., 2022, 15, 645–659 RSC.
- S. Shi, S. Zhang, Z. Xue, X. Yao, G. Zhang, J. Gao, Y. Li, X. Tu, S. Zhang, C. Zhang, Z. Liu, Z. Tang, H. Zhong, W. Li and Z. Fei, Near-Infrared Acceptors with Imide-Containing End Groups for Organic Solar Cells, ACS Appl. Mater. Interfaces, 2023, 15, 12119–12126 CrossRef CAS PubMed.
- M. Hao, T. Liu, Y. Xiao, L.-K. Ma, G. Zhang, C. Zhong, Z. Chen, Z. Luo, X. Lu, H. Yan, L. Wang and C. Yang, Achieving Balanced Charge Transport and Favorable Blend Morphology in Non-Fullerene Solar Cells via Acceptor End Group Modification, Chem. Mater., 2019, 31, 1752–1760 CrossRef CAS.
- H. Feng, X. Song, M. Zhang, J. Yu, Z. Zhang, R. Geng, L. Yang, F. Liu, D. Baran and W. Tang, Side chain engineering on dithieno[3,2-b:2,3-d]pyrrol fused electron acceptors for efficient organic solar cells, Mater. Chem. Front., 2019, 3, 702–708 RSC.
- P. Mondelli, G. Boschetto, P. N. Horton, P. Tiwana, C. Skylaris, S. J. Coles, M. Krompiec and G. Morse, Meta-analysis: the molecular organization of non-fullerene acceptors, Mater. Horiz., 2020, 7, 1062–1072 RSC.
- Y. Chen, F. Bai, Z. Peng, L. Zhu, J. Zhang, X. Zou, Y. Qin, H. K. Kim, J. Yuan, L.-K. Ma, J. Zhang, H. Yu, P. C. Y. Chow, F. Huang, Y. Zou, H. Ade, F. Liu and H. Yan, Asymmetric Alkoxy and Alkyl Substitution on Nonfullerene Acceptors Enabling High-Performance Organic Solar Cells, Adv. Energy Mater., 2021, 11, 2003141 CrossRef CAS.
- Y. Chen, Z. Wu, L. Ding, S. Zhang, Z. Chen, W. Li, Y. Zhao, Y. Wang and Y. Liu, Manipulating Crystal Stacking by Sidechain Engineering for High-Performance N-Type Organic Semiconductors, Adv. Funct. Mater., 2023, 33, 2304316 CrossRef CAS.
- S. Shinamura, I. Osaka, E. Miyazaki, A. Nakao, M. Yamagishi, J. Takeya and K. Takimiya, Linear- and Angular-Shaped Naphthodithiophenes: Selective Synthesis, Properties, and Application to Organic Field-Effect Transistors, J.
Am. Chem. Soc., 2011, 133, 5024–5035 CrossRef CAS PubMed.
- Y. Wang, H. Tatsumi, R. Otsuka, T. Mori and T. Michinobu, Highly-stable, green-solvent-processable organic thin-film transistors: angular-vs. linear-shaped carbazoledioxazine derivatives, J. Mater. Chem. C, 2018, 6, 5865–5876 RSC.
- T. Shen, W. Li, Y. Zhao, Y. Liu and Y. Wang, An all-C–H-activation strategy to rapidly synthesize high-mobility well-balanced ambipolar semiconducting polymers, Matter, 2022, 5, 1953–1968 CrossRef CAS.
- Q. Liu, Y. Wang, Y. Ren, A. Kohara, H. Matsumoto, Y. Chen, S. Manzhos, K. Feron, S. E. Bottle, J. Bell, Y. Zhou, T. Michinobu and P. Sonar, Diketopyrrolopyrrole-Based Dual-Acceptor Copolymers to Realize Tunable Charge Carrier Polarity of Organic Field-Effect Transistors and High-Performance Nonvolatile Ambipolar Flash Memories, ACS Appl. Electron. Mater., 2020, 2, 1609–1618 CrossRef CAS.
- Y. Wang, T. Hasegawa, H. Matsumoto and T. Michinobu, Significant Improvement of Unipolar n-Type Transistor Performances by Manipulating the Coplanar Backbone Conformation of Electron-Deficient Polymers via Hydrogen Bonding, J. Am. Chem. Soc., 2019, 141, 3566–3575 CrossRef CAS PubMed.
- S. Zhang, W. Li, Y. Chen, Z. Wu, Z. Chen, Y. Zhao, Y. Wang and Y. Liu, Perylenediimide regioisomers with tunable physicochemical and charge-transport properties, Chem. Commun., 2023, 59, 9876–9879 RSC.
- F. Lin, K. Jiang, W. Kaminsky, Z. Zhu and A. K. Y. Jen, A Non-fullerene Acceptor with Enhanced Intermolecular π-Core Interaction for High-Performance Organic Solar Cells, J. Am. Chem. Soc., 2020, 142, 15246–15251 CrossRef CAS PubMed.
- J.-L. Wang, K.-K. Liu, L. Hong, G.-Y. Ge, C. Zhang and J. Hou, Selenopheno[3,2-b]thiophene-Based Narrow-Bandgap Nonfullerene Acceptor Enabling 13.3% Efficiency for Organic Solar Cells with Thickness-Insensitive Feature, ACS Energy Lett., 2018, 3, 2967–2976 CrossRef CAS.
- Y. Liu, J. Song and Z. Bo, Designing high performance conjugated materials for photovoltaic cells with the aid of intramolecular noncovalent interactions, Chem. Commun., 2021, 57, 302–314 RSC.
- Y. Shi, W. Li, X. Wang, L. Tu, M. Li, Y. Zhao, Y. Wang and Y. Liu, Isomeric Acceptor–Acceptor Polymers: Enabling Electron Transport with Strikingly Different Semiconducting Properties in n-Channel Organic Thin-Film Transistors, Chem. Mater., 2022, 34, 1403–1413 CrossRef CAS.
- Y. Wang, T. Hasegawa, H. Matsumoto, T. Mori and T. Michinobu, D–A1–D–A2 Backbone Strategy for Benzobisthiadiazole Based n-Channel Organic Transistors: Clarifying the Selenium-Substitution Effect on the Molecular Packing and Charge Transport Properties in Electron-Deficient Polymers, Adv. Funct. Mater., 2017, 27, 1701486 CrossRef.
- C. Zhu, Z. Zhao, H. Chen, L. Zheng, X. Li, J. Chen, Y. Sun, F. Liu, Y. Guo and Y. Liu, Regioregular Bis-Pyridal[2,1,3]thiadiazole-Based Semiconducting Polymer for High-Performance Ambipolar Transistors, J. Am. Chem. Soc., 2017, 139, 17735–17738 CrossRef CAS PubMed.
- C. P. Yu, S. Kumagai, T. Kushida, M. Mitani, C. Mitsui, H. Ishii, J. Takeya and T. Okamoto, Mixed-Orbital Charge Transport in N-Shaped Benzene- and Pyrazine-Fused Organic Semiconductors, J. Am. Chem. Soc., 2022, 144, 11159–11167 CrossRef CAS PubMed.
- C. Yang, Q. An, H.-R. Bai, H.-F. Zhi, H. S. Ryu, A. Mahmood, X. Zhao, S. Zhang, H. Y. Woo and J.-L. Wang, A Synergistic Strategy of Manipulating the Number of Selenophene Units and Dissymmetric Central Core of Small Molecular Acceptors Enables Polymer Solar Cells with 17.5% Efficiency, Angew. Chem., Int. Ed., 2021, 60, 19241–19252 CrossRef CAS PubMed.
- Y. Chen, Y. Zheng, Y. Jiang, H. Fan and X. Zhu, Carbon-Bridged 1,2-Bis(2-thienyl)ethylene: An Extremely Electron Rich Dithiophene Building Block Enabling Electron Acceptors with Absorption above 1000 nm for Highly Sensitive NIR Photodetectors, J. Am. Chem. Soc., 2021, 143, 4281–4289 CrossRef CAS PubMed.
- Y. Wang, T. Hasegawa, H. Matsumoto and T. Michinobu, Significant Difference in Semiconducting Properties of Isomeric All-Acceptor Polymers Synthesized via Direct Arylation Polycondensation, Angew. Chem., Int. Ed., 2019, 58, 11893–11902 CrossRef CAS PubMed.
- Y. Chen, R. Ma, T. Liu, Y. Xiao, H. K. Kim, J. Zhang, C. Ma, H. Sun, F. Bai, X. Guo, K. S. Wong, X. Lu and H. Yan, Side-Chain Engineering on Y-Series Acceptors with Chlorinated End Groups Enables High-Performance Organic Solar Cells, Adv. Energy Mater., 2021, 11, 2003777 CrossRef CAS.
|
This journal is © the Partner Organisations 2024 |
Click here to see how this site uses Cookies. View our privacy policy here.