DOI:
10.1039/C1RA00305D
(Paper)
RSC Adv., 2011,
1, 79-82
Brønsted-acid catalyzed condensation-Michael reaction-Pictet–Spengler cyclization—highly stereoselective synthesis of indoloquinolizidines†
Received
14th June 2011
, Accepted 21st June 2011
First published on 26th July 2011
Abstract
A highly diastereoselective methodology for the efficient synthesis of functionalized indolo[2,3-a]quinolizidine skeletons in a one-pot operation has been developed. The protocol makes use of simple and inexpensive starting materials such as tryptamines, 1,3-dicarbonyl compounds and α,β-unsaturated aldehydes in the presence of a Brønsted acid catalyst.
Introduction
The synthesis of complex organic molecules with multiple stereocenters continues to be of interest for both academic and industrial laboratories.1 In particular, multicomponent domino and sequential reactions, amenable for the total synthesis of complex natural products and synthetic building blocks, are highly desirable.2,3 These methods allow for the rapid construction of complex molecular structures in an easy and efficient manner. They avoid costly and time consuming processes, including purification of the intermediates or additional steps involving protection/deprotection of functional groups. Cascade and sequential reactions in organic synthesis are generally very clean and have high atom economy.2
Indole alkaloids constitute an important class of natural products.4,5 In particular, the indolo[2,3-a]quinolizidine skeleton is a common structural unit found in a large number of pharmaceutically important products6 such as the antidepressant alkaloid yohimbine,7 the blood pressure lowering alkaloid reserpine, the antiarrhythmic alkaloids ajmaline and ajmalicine,8 and hirsutine alkaloids9 which are ganglionic blocking agents showing long-lasting neuroblocking activity (Fig. 1). These compounds were also shown to be useful in increasing femoral and vertebral blood flow.10 Despite their recognized value, the common methods for their synthesis involve multi-step synthetic routes for the construction of different bonds and establishment of functional groups. An organocatalytic reaction cascade has been reported by Franzén and co-workers for the synthesis of quinolizidine derivatives.11a,b Recently, Zhao and co-workers developed a one-pot two-step procedure for the synthesis of indolo[2,3-a]quinolizidines. Their procedure involves a prolinol catalyzed Michael addition followed by Pictet–Spengler cyclization that requires stoichiometric amounts of acid, long reaction times and high temperature.11
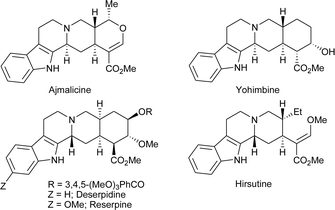 |
| Fig. 1
Natural products having an indoloquinolizidine moiety. | |
We now wish to report a simple and efficient protocol for the preparation of indoloquinolizidine skeleton starting from easily accessible starting materials such as tryptamine, β-keto esters or 1,3-diketones and α,β-unsaturated aldehydes.
When designing our new reaction sequence we envisioned a Brønsted acid catalyzed three component reaction in which the Brønsted acid would be capable of activating both, electrophiles and nucleophiles (Scheme 1).12,13
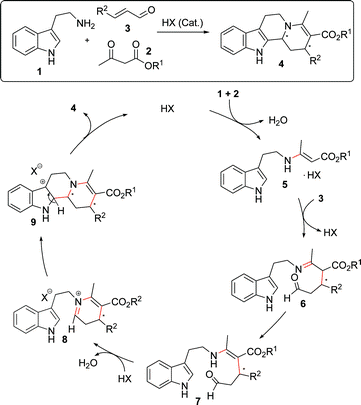 |
| Scheme 1 Hypothetical mechanism for the Brønsted-acid catalyzed reaction sequence. | |
We assumed that in an initial step, tryptamine (1) condenses with ketoester 2 to form an enaminone 5 that can undergo a Brønsted-acid catalyzed intermolecular Michael reaction with the unsaturated aldehyde 3 generating a new C–C bond. Subsequent tautomerization yields 7 which possesses the appropriate spatial orientation for the intramolecular formation of an iminium ion by the reaction of the secondary amine and aldehyde moieties. The resulting highly reactive iminium ion 8 undergoes a Brønsted-acid catalyzed Pictet–Spengler cyclization14 to form the final assembly of tetracyclic indolo[2,3-a]quinolizidine structure 4. Water is the only byproduct produced during the reaction.
Results and discussion
We initiated our studies by reacting 1.0 equiv. of tryptamine (1) with 1.1 equiv. of ethylacetoacetate (2a) in the presence of 10 mol% of Brønsted acid catalyst at room temperature. After the complete formation of the enaminone 5a, 1.5 equiv. of trans-cinnamaldehyde (3a) were added to the reaction mixture. Of the many Brønsted acids we studied, diphenylphosphate (DPP) gave the best results. The order of addition of reaction partners was found to be crucial for the success of the reaction. Other Brønsted acids such as p-TsOH or TFA also catalyzed the reaction sequence, but the yields were low. The reaction was studied in different solvents and we found that DCM gives the best results (Table 1). The reaction between tryptamine (1) and ethylacetoacetate (2a) in the presence of 10 mol% of DPP in DCM gave the intermediate enaminone 5a in 2 h. This compound was then reacted with the trans-cinnamaldehyde (3a) in the same pot to give the indolo[2,3-a]quinolizidine skeleton 4a in 76% yield after 12 h. While other solvents such as THF or mixtures of solvents such as DCM/THF or toluene/THF furnished the product in low yields, toluene alone led to a complicated mixture. Finally, we found that adding a slight excess of cinnamaldehyde (3a, 2.0 equiv.) provides the product in a good yield of 83% (Table 1, entry 5).
Table 1
Solvent evaluation in the Brønsted-acid catalyzed reaction sequence
We were delighted to find that the reaction exhibited high diastereoselectivity for the Pictet–Spengler cyclization and gave indolo[2,3-a]quinolizidine 4a with a cis relation between the phenyl group and the proton at the second chiral center (only one diastereomer was detected by 1H NMR of the crude reaction mixture).15
The scope of this three component reaction sequence was studied by employing different 1,3-dicarbonyl compounds and unsaturated aldehydes under the optimized conditions. The reaction showed good compatibility with a wide range of α,β-unsaturated aldehydes. Cinnamaldehydes 3b–g, substituted with different functional groups on the aromatic rings were successfully used in the reaction (Table 2, entries 2–7). Both electron-donating (para-methoxy, para-dimethylamino) and electron-withdrawing (ortho- and para-nitro) substituted aldehydes 3b–e provided the desired products 4b–e in good yields and high diastereoselectivities (Table 2, entries 2–5). The scope of the reaction was further extended by the use of ortho- and para-bromo substituted cinnamaldehydes 3f–g as the corresponding products can be further functionalized by metal-catalyzed cross-couplings or other reactions. When using 2-furylacrolein 3h, the key cyclized product 4h was isolated in 88% yield (Table 2, entry 8). The reaction was further extended to aliphatic substituted unsaturated aldehydes 3i–k. In these cases the corresponding indoloquinolizidine products 4i–k were isolated with slightly lower yields but high diastereoselectivities (Table 2, entries 9–11).
Table 2 Scope of different aldehydes in the Brønsted-acid catalyzed reaction sequence

|
Entrya |
3
|
R |
Product |
Yieldb (%) |
dec (%) |
General conditions: (1) 1.0 equiv. 1, 1.5 equiv. 2a, DPP (10 mol%), DCM, 4 Å MS, rt, 2h. (2) 1.5 equiv. 3a, 12h.
Yield of isolated product after column chromatography.
Only one diastereomer was detected by 1H NMR of the crude reaction mixture.
|
1 |
3a
|
Ph |
4a
|
83 |
>95 |
2 |
3b
|
o-NO2Ph
|
4b
|
80 |
>95 |
3 |
3c
|
p-NO2Ph
|
4c
|
79 |
>95 |
4 |
3d
|
p-MeOPh
|
4d
|
82 |
>95 |
5 |
3e
|
p-(Me2N)Ph
|
4e
|
77 |
>95 |
6 |
3f
|
p-BrPh
|
4f
|
85 |
>95 |
7 |
3g
|
o-BrPh
|
4g
|
84 |
>95 |
8 |
3h
|
2-furyl
|
4h
|
88 |
>95 |
9 |
3i
|
n-Bu
|
4i
|
78 |
>95 |
10 |
3j
|
i-Pr
|
4j
|
74 |
>95 |
11 |
3k
|
Me |
4k
|
71 |
>95 |
In order to further expand the scope of the reaction, we explored other ketoesters in this Brønsted-acid catalyzed reaction sequence. For this purpose, differently substituted acetoacetates 2a–d, as activated methylene compounds, were employed in the reaction (Table 3, Entries 1–4). Using allylacetoacetate 2d as the reaction partner is particularly interesting due to the useful allyl ester functionality present in the final product. To further illustrate the power of this Brønsted-acid catalyzed reaction, other nucleophiles like diketones were examined under the same reaction conditions. The enaminone derived from acetylacetone (2e) reacted with cinnamaldehyde (3a) and para-bromocinnamaldehyde (3f) providing the desired products 4o–p in good yields of 84 and 82% respectively (Table 3, entries 5–6).
Table 3 Scope of different dicarbonyl substrates in the DPP catalyzed reaction sequencea
2a: R = OEt, 2b: R = Oi-Pr, 2c: R = Ot-Bu, 2d: R = OAllyl, 2e: R = Me. Only one diastereomer was detected by 1H NMR of the crude reaction mixture.
|
|
The high diastereoselectivities observed in this Brønsted-acid catalyzed reaction sequence are most likely a consequence of the steric repulsion occurring between the indole ring and the equatorial β-proton during the Pictet–Spengler cyclization. The indole ring selectively reacts with the iminium ion from the opposite side of the bulky aromatic or aliphatic group. Notable advantages associated with this transformation are the mild reaction conditions and wide availability of different dicarbonyl compounds and unsaturated aldehydes which allows the easy functionalization of the products.
Given the success of diphenylphosphate (DPP) in catalyzing this transformation, and the interest our group has in asymmetric Brønsted acid catalysis,13 we decided to investigate this highly diastereoselective sequential reaction in the presence of different chiral BINOL-derived phosphoric acids.16–18 A systematic examination of different reaction parameters such as solvent, temperature and concentration was carried out. Enaminone 5a, synthesized by the reaction of tryptamine (1) with ethylacetoacetate (2a), was treated with 1.5 equiv. of cinnamaldehyde (3a) in the presence of BINOL-derived phosphoric acids. Using 5 mol% of the chiral phosphoric acid 10 substituted with sterically demanding triphenylsilyl groups in the 3,3′-positions gave the product 4a in 68% yield and 49% ee after 4 days (Scheme 2). However, further studies need to be performed to improve the selectivity of the reaction.
Conclusions
In summary, we have developed a highly efficient and diastereoselective one-pot reaction of tryptamine with 1,3-dicarbonyl compounds and unsaturated aldehydes. Notably, in the course of the reaction, four new bonds are constructed of which two are C–C bonds generating chiral centers. Several unsaturated aldehydes have been employed and afforded the corresponding products in good yields and high diastereoselectivities. The usefulness of this method is certified by its efficiency, easy operability, mild reaction conditions, cheap starting materials as well as excellent diastereoselectivity.
Acknowledgements
The authors would like to thank Swiss National Science Foundation (SNF) for a postdoc fellowship to CMRV and the DFG for financial support. MR gratefully acknowledges the ERC for a starting grant.
References
-
(a) K. C. Nicolaou, T. Montagnon and S. A. Snyder, Chem. Commun., 2003, 5, 551 RSC;
(b) K. C. Nicolaou, D. J. Edmonds and P. C. Bulger, Angew. Chem., Int. Ed., 2006, 45, 7134 CrossRef CAS;
(c) R. M. de Figueiredo and M. Christmann, Eur. J. Org. Chem., 2007, 2575 CrossRef CAS;
(d) M. J. Gaunt, C. C. C. Johansson, A. McNally and N. T. Vo, Drug Discovery Today, 2007, 12, 8 CrossRef CAS.
- For selected reviews on domino reactions:
(a) L. F. Tietze and U. Beifuss, Angew. Chem., Int. Ed. Engl., 1993, 32, 131 CrossRef;
(b) L. F. Tietze, Chem. Rev., 1996, 96, 115 CrossRef CAS;
(c) L. F. Tietze and A. Modi, Med. Res. Rev., 2000, 20, 304 CrossRef CAS;
(d)
L. F. Tietze, G. Brasche and K. M. Gericke, Domino Reactions in Organic Synthesis, Wiley-VCH, Weinheim, 2006 Search PubMed;
(e) H. C. Guo and J. A. Ma, Angew. Chem., Int. Ed., 2006, 45, 354 CrossRef CAS;
(f) H. Pellissier, Tetrahedron, 2006, 62, 1619 CrossRef CAS;
(g) H. Pellisier, Tetrahedron, 2006, 62, 2143 CrossRefFor reviews on organocatalytic domino reactions see:
(h) D. Enders, C. Grondal and M. R. M. Hüttl, Angew. Chem., Int. Ed., 2007, 46, 1570 CrossRef CAS;
(i) X. Yu and W. Wang, Org. Biomol. Chem., 2008, 6, 2037 RSC;
(j) T. Poisson, Synlett, 2008, 147 CrossRef CAS;
(k) A.-N. Alba, X. Companyó, M. Viciano and R. Rios, Curr. Org. Chem., 2009, 13, 1432 CrossRef CAS;
(l) C. Grondal, M. Jeanty and D. Enders, Nature Chem., 2010, 2, 167 CrossRef CAS.
-
(a) D. J. Ramón and M. Yus, Angew. Chem., Int. Ed., 2005, 44, 1602 CrossRef;
(b)
J. Zhu and H. Benaymé, Multicomponent Reactions, Wiley-VCH, Weinheim, 2005. CrossRef;
(c) D. M. D'Souza and T. J. J. Mueller, Chem. Soc. Rev., 2007, 36, 1095 RSC.
-
(a)
M. Hesse, Alkaloids: Nature's Curse or Blessing?Wiley-VCH, New York, 2002. Search PubMed;
(b) J. Stockigt and S. Panjikar, Nat. Prod. Rep., 2007, 24, 1382 RSC;
(c) S. Hibino and T. Choshi, Nat. Prod. Rep., 2001, 18, 66 RSC;
(d)
G. A. Cordell, The Alkaloids: Chemistry and Biology, Academic Press, San Diego, 1998, 50, p. 260 Search PubMed;
(e) L. Yang, M. Hill, M. Wang, S. Panjikar and J. Stockigt, Angew. Chem., Int. Ed., 2009, 48, 5211 CrossRef CAS.
- M. Somei and F. Yamada, Nat. Prod. Rep., 2004, 21, 278 RSC.
-
(a)
A. Basha and A. Atta-ur-Rahman, Frontiers in Natural Product Research: Indole Alkaloids, Harwood Academic Publishers, Amsterdam, 1998. Search PubMed;
(b) L. F. Tietze and Y. Zhou, Angew. Chem., Int. Ed., 1999, 38, 2045 CrossRef CAS;
(c) T. Koike, Y. Shinohara, S. Tobinaga and N. Takeuchi, Chem. Pharm. Bull., 2000, 48, 1898 CAS;
(d) P. Shiqu, Z. Li, C. Mengshen and E. Winterfeldt, Liebigs Ann. Chem., 1993, 141 CrossRef.
- G. Sanacora, R. M Berman, A. Cappiello, D. A Oren, A. Kugaya, N. Liu, R. Gueorguieva, D. Fasula and D. S. Charney, Neuropsychopharmacology, 2004, 29, 1166 CrossRef CAS.
-
(a) J. Li, T. Wang, X. Yu, A. Peterson, R. Weber, D. Soerens, D. Grubisha, D. Bennett and J. M. Cook, J. Am. Chem. Soc., 1999, 121, 6998 CrossRef CAS;
(b) J. Li and J. M. Cook, J. Org. Chem., 1998, 63, 4166 CrossRef CAS.
-
(a) M. Lounasmaa, J. Miettinen, P. Hanhinen and R. Jokela, Tetrahedron Lett., 1997, 38, 1455 CrossRef CAS;
(b) E. Wenkert, Y. D. Vankar and J. S. Yadav, J. Am. Chem. Soc., 1980, 102, 7971 CrossRef CAS;
(c) N. Aimi, E. Yamanaka, J. Endo, S. Sakai and J. Haginiwa, Tetrahedron, 1973, 29, 2015 CrossRef CAS;
(d) N. Aimi, E. Yamanaka, J. Endo, S. Sakai and J. Haginiwa, Tetrahedron Lett., 1972, 11, 1081 CrossRef.
-
A. Buzaz, United Stated Patent 4353911. Search PubMed.
-
(a) J. Franzen and A. Fisher, Angew. Chem., Int. Ed., 2009, 48, 787 CrossRef CAS;
(b) W. Zhang and J. Franzen, Adv. Synth. Catal., 2010, 352, 499 CrossRef CAS. For Lewis base approches, see:
(c) X. Wu, X. Dai, L. Nie, H. Fang, J. Chen, Z. Ren, W. Cao and G. Zhao, Chem. Commun., 2010, 46, 2733 RSC;
(d) H. Fang, X. Wu, L. Nie, X. Dai, J. Chen, W. Cao and G. Zhao, Org. Lett., 2010, 12, 5366 CrossRef CAS;
(e) X. Dai, X. Wu, H. Fang, L. Nie, J. Chen, H. Deng and W. Cao, Tetrahedron, 2011, 67, 3034 CrossRef CAS;
(f)
M. Rueping, C. M. R. Volla and G. Raabe, unpublished results..
-
(a) H. Yamamoto and M. B. Boxer, Chimia, 2007, 61, 279 CrossRef CAS;
(b) H. Yamamoto, Proc. Jpn. Acad., Ser. B, Phys. Biol. Sci., 2008, 84, 134 CrossRef CAS;
(c) H. Yamamoto, Tetrahedron, 2007, 63, 8377 CrossRef CAS;
(d) C. H. Cheon and H. Yamamoto, Chem. Commun., 2011, 47, 3043 RSC;
(e)
H. Yamamoto and K. Ishihara ed. Acid Catalysis in Modern Organic Synthesis Vols. 1 and 2 Wiley-VCH, Weinheim, 2008. Search PubMed.
- Reviews on chiral Brønsted acid catalysis:
(a) C. Bolm, T. Rantanen, I. Schiffers and L. Zani, Angew. Chem., Int. Ed., 2005, 44, 1758 CrossRef CAS;
(b) T. Akiyama, J. Itoh and K. Fuchibe, Adv. Synth. Catal., 2006, 348, 999 CrossRef CAS;
(c) T. Akiyama, Chem. Rev., 2007, 107, 5744 CrossRef CAS;
(d) A. G. Doyle and E. N. Jacobsen, Chem. Rev., 2007, 107, 5713 CrossRef CAS;
(e) D. Kampen, C. M. Reisinger and B. List, Top. Curr. Chem., 2010, 291, 395 CrossRef CAS;
(f)
H. Yamamoto and N. Payette, in Hydrogen Bonding in Organic Synthesis (Ed. P. M. Pihko) Wiley-VCH, Weinheim, 2009, pp. pp. 73–140 Search PubMed.
-
(a) M. S. Taylor and E. N. Jacobsen, J. Am. Chem. Soc., 2004, 126, 10558 CrossRef CAS;
(b) J. Seayad, A. M. Seayad and B. List, J. Am. Chem. Soc., 2006, 128, 1086 CrossRef CAS;
(c) M. J. Wanner, R. N. van der Haas, K. R. de Cuba, J. H. van Maarseveen and H. Hiemstra, Angew. Chem., Int. Ed., 2007, 46, 7485 CrossRef CAS;
(d) N. V. Sewgobind, M. J. Wanner, S. Ingemann, R. de Gelder, J. H. van Maarseveen and H. Hiemstra, J. Org. Chem., 2008, 73, 6405 CrossRef CAS;
(e) M. E. Muratore, C. A. Holloway, A. W. Pilling, R. I. Storer, G. Trevitt and D. J. Dixon, J. Am. Chem. Soc., 2009, 131, 10796 CrossRef CAS;
(f) C. A. Holloway, M. E. Muratore, R. I. Storer and D. J. Dixon, Org. Lett., 2010, 12, 4720 CrossRef CAS;
(g) I. T. Raheem, P. S. Thiara, E. A. Peterson and E. N. Jacobsen, J. Am. Chem. Soc., 2007, 129, 13404–13405 CrossRef CAS;
(h) D. J. Mergott, S. J. Zuen and E. N. Jacobsen, Org. Lett., 2008, 10, 745 CrossRef CAS;
(i) I. T. Raheem, P. S. Thiara and E. N. Jacobsen, Org. Lett., 2008, 10, 1577 CrossRef CAS;
(j) R. S. Klausen and E. N. Jacobsen, Org. Lett., 2009, 11, 887–890 CrossRef CAS.
- See ESI† for further spectral details..
-
(a) T. Akiyama, J. Itoh, K. Yokota and K. Fuchibe, Angew. Chem., Int. Ed., 2004, 43, 1566 CrossRef CAS;
(b) D. Uraguchi and M. Terada, J. Am. Chem. Soc., 2004, 126, 5356 CrossRef CAS.
-
(a)
M. Rueping and E. Sugiono, Ernst Schering Foundation Symposium Proceedings, Vol. 2, 2007, p. 207 Search PubMed;
(b) M. Rueping, E. Sugiono and F. R. Schoepke, Synlett, 2010, 852 CrossRef CAS;
(c) M. Rueping, J. Dufour and F. R. Schoepke, Green Chem., 2011, 13, 1085–1105 RSC.
-
(a) M. Terada, Chem. Commun., 2008, 4097 RSC;
(b) M. Terada, Synthesis, 2010, 1929 CrossRef CAS;
(c) M. Terada, Bull. Chem. Soc. Jpn., 2010, 101 CrossRef CAS;
(d) A. Zamfir, S. Schenker, M. Freund and S. B. Tsogoeva, Org. Biomol. Chem., 2010, 8, 5262 CAS.
Footnote |
† Electronic supplementary information (ESI) available. See DOI: 10.1039/c1ra00305d |
|
This journal is © The Royal Society of Chemistry 2011 |
Click here to see how this site uses Cookies. View our privacy policy here.