DOI:
10.1039/C0SC00645A
(Edge Article)
Chem. Sci., 2011,
2, 1135-1140
Highly enantioselective palladium-catalyzed umpolung allylation of aldehydes†
Received
24th December 2010
, Accepted 16th March 2011
First published on 7th April 2011
Abstract
Compared with well-established electrophilic π-allylpalladium chemistry, the catalytic asymmetric reactions via umpolung of π-allylpalladium have received limited success. Although extensive efforts have been devoted, only modest enantioselectivities have been obtained in the palladium-catalyzed asymmetric umpolung allylation reactions. In this context, we disclose a highly enantioselective palladium-catalyzed umpolung allylation of aldehydes with allylic alcohols and their derivatives. By using sterically hindered chiral monodentate spiro phosphite ligands, we have accomplished the asymmetric allylation reaction with high yields and excellent enantioselectivities (up to 97% ee). These results represent the highest level of enantioselectivity for the umpolung allylation reactions. The present palladium-catalyzed asymmetric allylation reaction uses readily available allylic alcohols and their derivatives instead of sensitive allylic organometallic reagents, which provides a competitive alternative approach for preparation of versatile chiral homoallylic alcohols. A preliminary mechanism of palladium-catalyzed umpolung allylation reaction was discussed based on the experimental observations.
Introduction
π-Allylpalladium is a well-known and fundamental organometallic intermediate in palladium-catalyzed organic reactions.1 Following Trost's pioneering work,2 the asymmetric electrophilic substitution of π-allylpalladium, particularly asymmetric allylic alkylations (AAA), has become one of the most investigated benchmark reactions in current asymmetric synthesis (Scheme 1, left part).3 In fact, Trost et al. have demonstrated the amphiphilic property of π-allylpalladium species two decades ago.4 A variety of strategies have been developed to realize the “umpolung” of electrophilic π-allylpalladium to furnish nucleophilic transformations (Scheme 1, right part).5 With SnCl2, Et2Zn, Et3B, InI and other activating reagents, the nucleophilic additions of allyl to carbonyl compounds or iminesvia umpolung of π-allylpalladium have been accomplished and widely applied in organic synthesis.6 Compared with asymmetric electrophilic substitutions of π-allylpalladium, the asymmetric nucleophilic addition via umpolung of π-allylpalladium is much unexplored. Until 2004, Zanoni et al.7 reported the first catalytic asymmetric allylation of aldehydesvia umpolung of π-allylpalladium. By using chiral monodentate phosphorous ligands they obtained a moderate enantioselectivity (up to 75% ee) in the Pd-catalyzed asymmetric allylation of aldehydes with allylic acetate in the presence of Et2Zn. In 2005, we8 described a Pd-catalyzed asymmetric umpolung allylation of aldehydes with allylic alcohols in the presence of Et3B.9 By using chiral spiro phosphine ligands, up to 83% ee of enantioselectivities were achieved. From a synthetic point of view, the palladium-catalyzed asymmetric umpolung allylation of carbonyl compounds provides a new approach to chiral homoallylic alcohols, which are versatile intermediates for the synthesis of various useful compounds.10 Moreover, as π-allylpalladium can be easily generated from allylic compounds according to well established procedures,3 a large number of available allylic compounds can be used as allylic donors in the umpolung allylation reaction. Thus, the umpolung allylation provides an opportunity for avoiding the direct use of allylic organometallic reagents, which are predominantly allylic donors in current catalytic asymmetric allylations but suffer from many drawbacks such as air- or moisture-sensitivity and the need for a multi-step preparation.11 Attracted by their fundamental importance for modern palladium chemistry and great potential utilities in asymmetric carbon–carbon bond formation reactions, Feringa,12 Hou,13 and Shi14 tested an impressive range of chiral ligands, respectively for further improvement of the enantioselectivity of this useful transformation. Unfortunately, all of the tested catalysts afforded low to modest enantioselectivity (less than 81% ee).15 The low level of chiral induction in the umpolung allylation reaction is mainly attributed to the lack of efficient chiral ligands and the indistinct umpolung mechanism. Recently, we found that the highly bulky chiral monodentate spiro phosphites are very efficient ligands for palladium-catalyzed umpolung asymmetric allylation reactions. Herein we report the first highly enantioselective palladium-catalyzed allylation of aromatic, aliphatic, and α,β-unsaturated aldehydes with allylic alcohols and their derivatives in excellent enantioselectivity (up to 97% ee).
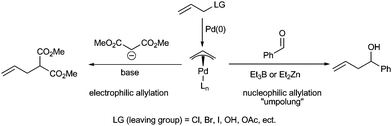 |
| Scheme 1 Amphiphilic π-allylpalladium complexes. | |
Results and discussion
Recently, we developed a number of chiral monodentate spiro phosphorous compounds based on a spirobiindane scaffold (Fig. 1),16 which provided a library of ligands for searching highly enantioselective ligands for palladium-catalyzed asymmetric allylation of aldehydes with allylic alcohols. The chiral ligands were evaluated in the reaction of 2-naphthylaldehyde (5a) with prop-2-en-1-ol (6a). In the presence of 5 mol% palladium acetate and 10 mol% phosphorous ligand, the allylation of 5a with 1.5 equiv. of 6a was performed in THF at 25 °C with Et3B as the umpolung reagent. When spiro phosphine ligand 1a was used, homoallylic alcohol product 7aa was isolated in 80% yield with 78% ee (Table 1, entry 1). Other spiro phosphine ligands 1b–1e bearing a different substituent on the P-phenyl ring gave the same level of enantioselectivity as that obtained with 1a, showing that the electronic and steric properties of phosphine ligands 1 have no influence on the enantioselectivity of the catalyst (entries 2–5). To improve the enantioselectivity of the reaction, we prepared a series of spiro phosphoramidites 2 and phosphites 3 and applied them in the palladium-catalyzed asymmetric allylation of aldehyde 5a. The phosphoramidite ligands 2 displayed even lower enantioselectivities (40–54% ee, entries 6–10). However, the results with the spiro phosphite ligands 3 varied depending on the R2group (entries 11–18). The enantioselectivity of the reaction was significantly enhanced by using sterically hindered spiro phosphite ligands 3c–e, which contained a bulky 2,6- or 3,5-substituted phenyl group (91–95% ee, entries 13–15). The yield of the reaction with ligand 3e was increased remarkably by conducting the reaction in Et2O, using Pd(dba)2 as the catalyst precursor, and adding 4 equiv. allylic alcohol. Under the optimized reaction conditions, the allylation product was isolated in 93% yield with 96% ee (entry 20). As a comparison, chiral phosphite ligand (S)-4 having a binaphthyl backbone was also examined in the allylation of aldehyde 5a, with the desired allylation product being obtained in only 5% yield with 73% ee (entry 22).
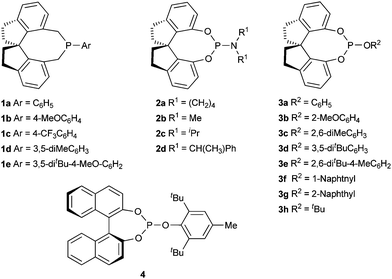 |
| Fig. 1 Chiral monophosphorous ligands. | |
Entry |
[Pd] |
Ligand |
Solvent
|
Time (h) |
Yieldb (%) |
Ee
c (%) |
Reaction conditions: [Pd]/ligand/5a/6a/Et3B = 0.014/0.028/0.28/0.42/1.4 (mmol), 1.6 mL solvent at 25 °C.
Isolated yield.
Determined by HPLC using Chiralcel OJ column.
4 equiv. allylic alcohol was used and the reaction was performed in 0.8 mL diethyl ether.
5 mol% ligand was used.
|
1 |
Pd(OAc)2 |
(S)-1a |
THF
|
12 |
80 |
78 |
2 |
Pd(OAc)2 |
(S)-1b |
THF
|
20 |
90 |
77 |
3 |
Pd(OAc)2 |
(S)-1c |
THF
|
12 |
93 |
79 |
4 |
Pd(OAc)2 |
(S)-1d |
THF
|
24 |
66 |
79 |
5 |
Pd(OAc)2 |
(R)-1e |
THF
|
20 |
96 |
80 |
6 |
Pd(OAc)2 |
(R)-2a |
THF
|
36 |
55 |
50 |
7 |
Pd(OAc)2 |
(R)-2b |
THF
|
18 |
36 |
45 |
8 |
Pd(OAc)2 |
(R)-2c |
THF
|
18 |
72 |
40 |
9 |
Pd(OAc)2 |
(Sa,S,S)-2d |
THF
|
12 |
90 |
50 |
10 |
Pd(OAc)2 |
(Sa,R,R)-2d |
THF
|
12 |
54 |
54 |
11 |
Pd(OAc)2 |
(S)-3a |
THF
|
60 |
27 |
62 |
12 |
Pd(OAc)2 |
(S)-3b |
THF
|
60 |
41 |
73 |
13 |
Pd(OAc)2 |
(S)-3c |
THF
|
55 |
39 |
91 |
14 |
Pd(OAc)2 |
(S)-3d |
THF
|
60 |
51 |
94 |
15 |
Pd(OAc)2 |
(S)-3e |
THF
|
60 |
47 |
95 |
16 |
Pd(OAc)2 |
(S)-3f |
THF
|
40 |
trace |
— |
17 |
Pd(OAc)2 |
(S)-3g |
THF
|
40 |
trace |
— |
18 |
Pd(OAc)2 |
(S)-3h |
THF
|
40 |
trace |
— |
19 |
Pd(dba)2 |
(S)-3e |
Et2O
|
72 |
70 |
96 |
20d |
Pd(dba)2 |
(S)-3e |
Et2O
|
72 |
93 |
96 |
21d,e |
Pd(dba)2 |
(S)-3e |
Et2O
|
72 |
68 |
96 |
22d |
Pd(dba)2 |
(S)-4 |
Et2O
|
72 |
5 |
73 |
A variety of aldehydes were investigated in the allylation reaction under the optimal conditions. All the tested aldehydes, 5, reacted smoothly with allylic alcohol 6a to generate corresponding homoallylic alcohols 7aa–7ma in moderate to high yields with excellent enantioselectivities (Table 2, entries 1–13). The substrates containing ortho-substituents (entries 3 and 4) or strong electron-withdrawing groups (entries 12 and 13) provided slightly lower enantioselectivities. Hetero-aromatic aldehydes, such as 2-furaldehyde (5n) and 2-thienaldehyde (5o) were also suitable substrates for the allylation reaction (entries 14 and 15). The highest enantioselectivity (97% ee) was obtained with 2-furaldehyde 5n as an allylic acceptor. In addition to aromatic aldehydes, the catalyst Pd(dba)2/3e was also efficient for the allylation of α,β-unsaturated aldehyde 5p, giving the homoallylic alcohol 6p in 88% yield with 94% ee, albeit a higher catalyst loading (10 mol%) was required (entry 16).
Entry |
R |
Product |
Yield (%) |
Ee (%) |
Reaction conditions were the same as those in Table 1, entry 20.
10 mol% catalyst was used.
|
1 |
2-Naphthyl (5a) |
7aa
|
93 |
96 (S) |
2 |
C6H5 (5b) |
7ba
|
65 |
95 (S) |
3 |
2-CH3C6H4 (5c) |
7ca
|
70 |
91 (S) |
4 |
2-ClC6H4 (5d) |
7da
|
90 |
89 (–) |
5 |
3-MeOC6H4 (5e) |
7ea
|
87 |
96 (–) |
6 |
3-CH3C6H4 (5f) |
7fa
|
72 |
96 (–) |
7 |
3-ClC6H4 (5g) |
7ga
|
82 |
93 (–) |
8 |
4-MeOC6H4 (5h) |
7ha
|
63 |
94 (S) |
9 |
4-CH3C6H4 (5i) |
7ia
|
69 |
95 (S) |
10 |
4-FC6H4 (5j) |
7ja
|
77 |
96 (–) |
11 |
4-ClC6H4 (5k) |
7ka
|
81 |
95 (S) |
12 |
4-CF3C6H4 (5l) |
7la
|
83 |
92 (S) |
13 |
3,4-diClC6H4 (5m) |
7ma
|
86 |
92 (–) |
14 |
2-Furyl (5n) |
7na
|
52 |
97 (S) |
15 |
2-Thienyl (5o) |
7oa
|
60 |
96 (S) |
16b |
E-PhCH CH (5p) |
7pa
|
88 |
94 (S) |
For further extending the scope of substrates, we examined the substituted allylic alcohols as allylation reagents. When 2-methylprop-2-en-1-ol (6b) or 2-phenylprop-2-en-1-ol (6c) were reacted with 2-naphthaldehyde (5a) or benzaldehyde (5b), respectively, homoallylic alcohols bearing a 2,2-substituted olefin, which are very useful building blocks in organic syntheses,17 were produced in high yields and excellent enantioselectivities (Scheme 2). In contrast, the 3-substituted allylic alcohols including cinnamyl alcohol and crotyl alcohol and the 1-phenyl allylic alcohol all showed extremely low reactivity under the same reaction conditions, leading to no allylation products. These results indicated that the highly bulky phosphite ligand 3e prevented the formation of the π-allylpalladium species having a substituent on either C1 or C3.18
The asymmetric allylation of aliphatic aldehydes has been widely used as a key step in the synthesis of natural products and pharmaceutical compounds.19 Encouraged by the success in palladium-catalyzed umpolung allylation of aromatic aldehydes, we then tried more challenging aliphatic aldehydes. To our delight, the present catalyst was also efficient for the allylation of 3-phenylpropanal (5q) with allylic alcohol 6a. In the presence of 10 mol% of catalyst, the allylation of 5q performed smoothly and produced homoallylic alcohol (R)-7qa in high yield (86%) with excellent enantioselectivity (93% ee) (Scheme 3). More importantly, the competitive electrophilic allylation product 8 at the α-position of the aldehyde was not detected in our reaction, which was different from the results reported by Tamaru who obtained mainly α-allylation products by using a PPh3 ligand.20
The allylation reactivity of aliphatic aldehydes was lower than aromatic aldehydes. When the catalyst loading was decreased from 10 mol% to 5 mol%, the allylation reaction of aldehyde 5q with allylic alcohol 6a became very sluggish and the allylation product 7qa was isolated with only 58% yield. However, the yield of allylation product can be improved to 83% by adding 80 mg silica gel (Scheme 3). The reason for the improvement of the reaction yield by silica gel is unclear.
Under the optimal reaction conditions, the allylations of various aliphatic aldehydes with allylic alcohols processed smoothly to produce the corresponding homoallylic alcohols in good yields (57–91%) with high enantioselectivities (83–94% ee) (Scheme 4). Besides the wide substrate scope, another impressive feature of the current allylation reaction is its high tolerance with functional groups. For instance, the aliphatic aldehydes containing ether (5r–5t), ester (5u), amide (5x), ketone (5y), olefin (5z), and indole (5w) at the side chain underwent the allylation reaction to give corresponding homoallylic alcohols in good yields with high enantioselectivities. The present catalyst reacted specifically with aldehydes, and other electrophilic functional groups such as ketone, ester, and amide were inert under the standard reaction conditions. The high chemoselectivity to aldehydes of the palladium-catalyzed umpolung allylation reaction indicated a high potential for its utilities in organic synthesis. Similar to aromatic aldehydes, the aliphatic aldehydes can react with 2-substituted prop-2-en-1-ols 6b–6e efficiently. The 2-substituents of allylic alcohol had almost no effect on the enantioselectivity, and all the tested 2-substituted allylic alcohols afforded essentially identical enantioselectivities (83–86% ee). The 2-methylprop-2-en-1-ol 6b gave a higher yield compared with 2-aryl-substituted allylic alcohols 6c–6e.
Besides aldehydes, different ketones as well as aldimines have also been evaluated under the standard reaction conditions. When simple ketone acetophenone and aldimineN-benzylidene-4-methylbenzenesulfonamide were used, no allylation reaction took place. However, the active ketone 1-methylindoline-2,3-dione (isatine) performed the allylation reaction to give the corresponding allylation product in 87% yield within 16 h, albeit with only 17% ee.
As aforementioned, many allylic compounds with appropriate leaving groups can form π-allylpalladium intermediate via oxidative addition to Pd(0) under mild conditions.3 Thus the palladium-catalyzed umpolung allylation of aldehyde can use various allylic compounds as an allyl donor, theoretically. To demonstrate the merit of palladium-catalyzed umpolung allylation in the choice of allyl donors, we examined many commonly used allylic compounds in the allylation reaction. By using allyl acetate instead of allylic alcohol 6a as the allyl donor, the palladium-catalyzed asymmetric allylation of 2-naphthaldehyde (5a) proceeded smoothly to give homoallylic alcohol 7aa in 98% yield but with only moderate enantioselectivity (38% ee) under the standard conditions. Gratifyingly, when we replaced Et3B with Et2Zn, the Pd/3e-catalyzed allylation of 2-naphthylaldehyde with allyl acetate afforded 7aa in 97% yield with 95% ee (Table 3, entry 1). Under optimal reaction conditions with Et2Zn, a number of readily available allyl donors such as allylic halides (entries 2 and 3), allylic esters (entries 4–6), and allylic ethers (entry 7) have been successfully applied in the asymmetric allylation of 2-naphthylaldehyde. The leaving groups of the allyl donors slightly affect the reactivity and enantioselectivity of allylation reaction. All tested allyl donors afforded good to high yield (61–97%) and excellent enantioselectivity (90–95% ee) (entries 1–7).
Entry |
X |
Yield (%)b |
Ee (%)c |
Reaction conditions: Pd(dba)2/(R)-3e/5a/allylic reagent/Et2Zn = 0.014/0.028/0.28/0.42/0.28 (mmol), in 2 mL THF at 10 °C for 5 days.
Isolated yield.
Determined by HPLC using a chiral column.
|
1 |
OAc
|
97 |
95 (R) |
2 |
Br
|
81 |
90 (R) |
3 |
Cl
|
87 |
92 (R) |
4 |
OCO2Me
|
61 |
94 (R) |
5 |
OPO(OEt)2 |
85 |
93 (R) |
6 |
OCOPh
|
67 |
94 (R) |
7 |
OPh
|
71 |
94 (R) |
Mechanism consideration
Tamaru et al.6c explained the umpolung of allylpalladium by a transmetallation mechanism (Scheme 5, part A). They proposed that the allylpalladium intermediate transfers the allyl group to the more electropositive zinc or boron to generate an allylic zinc or allylic boron reagent, which completed the allylation with aldehyde to produce homoallylic alcohol. Whilst the mechanism rationalized the non-asymmetric reaction, it is difficult to reconcile with the results gained from asymmetric umpolung allylation reaction. Since the palladium complex is not involved in the allylation step according to Tamaru's mechanism, the palladium catalyst bearing chiral ligands cannot furnish a chiral induction and consequently gives no enantioselectivity. We conducted the experiments to study the boron–phosphite interaction viaNMR. When Et3B was introduced into a THF-D8 solution of ligand (R)-3e, the chemical shift of 31P (δ 122.9, s) remained unchanged. Additionally, when Et3B was introduced into a THF-D8 solution of Pd/(R)-3e, the chemical shift of coordinating P atom (31P, δ 16.8, s) was only shifted slightly to higher field (δ 18.6, s) and no new 31P signal appeared. These observations showed no chiral ligand coordinated to the boron and implied that the presumption of the chiral induction through chiral allylboron reagent is unreasonable. Because Pd(II) species are involved in the catalytic cycle, the chiral ligands ligated to Pd(II) may fulfill the chiral induction through a Lewis acid manner. However, when a bidentated ligand such as BINAP, BOX, Phox, etc. was used, the reaction is either fully prohibited or afforded a racemic product. This experiment result conflicted with a Lewis acid catalysis mechanism.
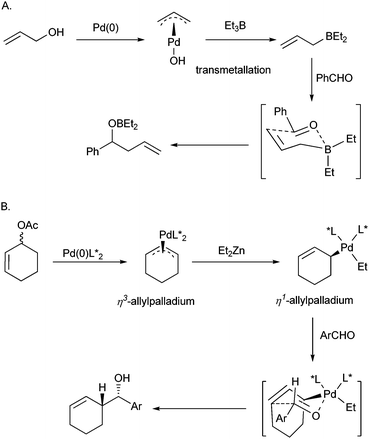 |
| Scheme 5 The proposal mechanisms of π-allylpalladium umpolung in the literature. A. Tamaru's transmetallation process. B. Minnaard and Feringa's Et2Zn assistant η3- to η1-allylpalladium transfer process. | |
To explain the chiral induction in the umpolung allylation reaction, Minnaard and Feringa12 proposed an alternative mechanism based on Szabó's observations on the nucleophilic η1-allylpalladium21 (Scheme 5, part B). They proposed that the Et2Zn transferred a ethyl group to η3-allylpalladium and promoted the formation of η1-allylpalladium intermediate. Subsequently, via a six-member ring transition state containing chiral palladium species, the chiral induction is realized.
The presumption of η3- to η1-allylpalladium transfer process assisted by Et2Zn explained the chiral induction in Pd-catalyzed asymmetric umpolung allylation reactions. However, the coordination number of palladium reaches five in the transition state in the Minnaard and Feringa's mechanism, which is uncommon in Pd(II) coordination patterns. Because all the tested bidentate ligands gave racemic products and one equivalent monodentated ligand (Pd/3e = 1
:
1) afforded the same level of enantioselectivity (Table 1, entry 21) in the Pd-catalyzed asymmetric umpolung allylation reaction, it is most likely that only one chiral ligand coordinated with palladium in the chiral induction step.
Based on our experimental observations and aforementioned analysis, we proposed an allylpalladium umpolung mechanism illustrated in Scheme 6. Firstly, the oxidative addition of allylic alcohol to Pd(0), activated by Et3B, forms η3-allylpalladium species II (step a), which is electrophilic. Et3B transfers an ethyl group to palladium to form a η1-allylpalladium intermediate III (step b). The ligated ethyl group enhances the electron density of palladium and thus facilitates the transformation of electrophilic η3-allylpalladium II to nucleophilic η1-allylpalladium III. The exchange of a ligand of intermediate III with an aldehyde forms a Pd–aldehyde complex, which generates a homoallylic alcohol product through a six-member ring transition state (TS). The Pd(0) catalyst is regenerated by reductive coupling or elimination of ethyl groups in palladium species IV and the catalytic cycle is accomplished (step d). We believe that the higher stability and rigidity of ligand 3e with a spirobiindane backbone compared with the ligand 4 with a binaphthyl backbone were beneficial to stabilization of the TS and thus exhibited higher reactivity and enantioselectivity (Table 1, entries 20 and 22).
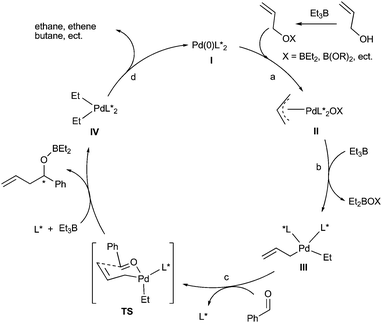 |
| Scheme 6 A plausiable π-allylpalladium umpolung mechanism. | |
Although our experiments supported the mechanism involving organometal-assisted η3- to η1-allylpalladium transfer process, the other competitive umpolung mechanisms may also exist during the transformation, which make the chiral control difficult in this reaction. As the enantioselectivity of the umpolung allylation is temperature sensitive, we believe Tamaru's transmetallation umpolung process may proceeds as a background reaction especially when Et2Zn is used as activating reagent.
Conclusions
We have developed a highly enantioselective Pd-catalyzed umpolung allylation of aldehydes with allylic alcohols and their derivatives. By using sterically hindered chiral spiro phosphite ligands, various homoallylic alcohols were prepared in high yields with excellent enantioselectivities. These results represent the highest level of enantioselectivities for umpolung allylations and are comparable to the best results achieved with allylic organometallic reagents. The broad scopes of substrates and allyl donors and high tolerance to the functional group indicated a potential for wide application of the Pd-catalyzed asymmetric umpolung allylation reaction in the synthesis of chiral homoallylic alcohols.
Acknowledgements
We thank the National Natural Science Foundation of China, the National Basic Research Program of China (973 Program, 2010CB833300, 2011CB808600), and the Ministry of Health of China (2009ZX09501-017) for financial support.
Notes and references
-
(a)
J. Tsuji, Palladium Reagents and Catalysts: Innovations in Organic Synthesis, John Wiley & Sons, Chichester, WS, 1995, chapter 4.2 Search PubMed;
(b)
J. Tsuji, Palladium Reagents and Catalysts: New Perspectives for the 21st Century,John Wiley & Sons, Chichester, WS, 2004, chapter 4 Search PubMed;
(c)
R. H. Crabtree, the Organometallic Chemistry of the Transition Metals,Wiley-Interscience, Hoboken, NJ, ed. 4, 2005, chapters 5 and 14 Search PubMed.
- B. M. Trost and P. E. Strege, J. Am. Chem. Soc., 1977, 99, 1649–1651 CrossRef CAS.
- For reviews, see:
(a)
A. Pfaltz and M. Lautens, in Comprehensive Asymmetric Catalysis, E. N. Jacobsen, A. Pfaltz and H. Yamamoto, ed.; Springer-Verlag: Heidelberg, 1999; Chapter 24 Search PubMed;
(b) B. M. Trost and D. L. V. Vranken, Chem. Rev., 1996, 96, 395–422 CrossRef CAS;
(c) G. Helmchen and A. Pfaltz, Acc. Chem. Res., 2000, 33, 336–345 CrossRef CAS;
(d) B. M. Trost and M. L. Crawley, Chem. Rev., 2003, 103, 2921–2943 CrossRef CAS.
-
(a) B. M. Trost and J. W. Herndon, J. Am. Chem. Soc., 1984, 106, 6835–6837 CrossRef CAS;
(b) B. M. Trost and R. Walchli, J. Am. Chem. Soc., 1987, 109, 3487–3488 CrossRef CAS.
- For reviews, see:
(a) Y. Tamaru, J. Organomet. Chem., 1999, 576, 215–231 CrossRef CAS;
(b) J. A. Marshall, Chem. Rev., 2000, 100, 3163–3185 CrossRef CAS;
(c) G. Zanoni, A. Pontiroli, A. Marchetti and G. Vidari, Eur. J. Org. Chem., 2007, 3599–3611 CrossRef CAS.
- For representative examples, see:
(a) Y. Masuyama, J. P. Takahara and Y. Kurusu, J. Am. Chem. Soc., 1988, 10, 4473–4474 CrossRef;
(b) Y. Tamaru, A. Tanaka, K. Yasui, S. Goto and S. Tanaka, Angew. Chem., Int. Ed. Engl., 1995, 34, 787–789 CrossRef CAS;
(c) M. Kimura, T. Tomizawa, Y. Horino, S. Tanaka and Y. Tamaru, Tetrahedron Lett., 2000, 41, 3627–3629 CrossRef CAS;
(d) S. Araki, T. Kamei, T. Hirashita, H. Yamamura and M. Kawai, Org. Lett., 2000, 2, 847–849 CrossRef CAS;
(e) O. A. Wallner and K. J. Szabó, Org. Lett., 2002, 4, 1563–1566 CrossRef CAS;
(f) R. Mukai, Y. Horino, S. Tanaka, Y. Tamaru and M. Kimura, J. Am. Chem. Soc., 2004, 126, 11138–11139 CrossRef CAS;
(g) N. Selander, A. Kipke, S. Sebelius and K. J. Szabó, J. Am. Chem. Soc., 2007, 129, 13723–13731 CrossRef CAS. For other transition metal-catalyzed nucleophilic allylations using allylic alcohols, see: Ni
(h) T. Hirashita, S. Kambe, H. Tsuji, H. Omori and S. Araki, J. Org. Chem., 2004, 69, 5054–5059 CrossRef CAS; Rh
(i) Y. Masuyama, Y. Kaneko and Y. Kurusu, Tetrahedron Lett., 2004, 45, 8969–8971 CrossRef CAS; Ir
(j) Y. Masuyama, T. Chiyo and Y. Kurusu, Synlett, 2005, 14, 2251–2253 CrossRef;
(k) M. Banerjee and S. Roy, J. Mol. Catal. A: Chem., 2006, 246, 231–236 CrossRef CAS.
- G. Zanoni, S. Gladiali, A. Marchetti, P. Picoinini, I. Tredic and G. Vidari, Angew. Chem., Int. Ed., 2004, 43, 846–849 CrossRef CAS.
- S.-F. Zhu, Y. Yang, L.-X. Wang, B. Liu and Q.-L. Zhou, Org. Lett., 2005, 7, 2333–2335 CrossRef CAS.
- The non-asymmetric Pd-catalyzed umpolung allylation of aldehydes with allylic alcohols was originally reported by Tamaru et al., see ref. 6c.
- For reviews, see:
(a)
W. R. Roush, in Comprehensive Organic Synthesis, B. M. Trost, I. Fleming and C. H. Heathcock, ed.; Pergamon: Oxford, UK, 1991; Vol. 2, p 1 Search PubMed;
(b) Y. Yamamoto and N. Asao, Chem. Rev., 1993, 93, 2207–2293 CrossRef CAS;
(c) J. A. Marshall, Chem. Rev., 1996, 96, 31–47 CrossRef CAS.
- For reviews on catalytic asymmetric allylation of carbonyl compounds, see:
(a)
A. Yanagisawa, in Comprehensive Asymmetric Catalysis; E. N. Jacobsen, A. Pfaltz and H. Yamamoto, ed.; Springer-Verlag: Heidelberg, 1999; Chapter 27 Search PubMed;
(b) S. E. Denmark and J.-P. Fu, Chem. Rev., 2003, 103, 2763–2793 CrossRef CAS.
- G. P. Howell, A. J. Minnaard and B. L. Feringa, Org. Biomol. Chem., 2006, 4, 1278–1283 RSC.
- T.-Z. Zhang, L.-X. Dai and X.-L. Hou, Tetrahedron: Asymmetry, 2007, 18, 251–259 CrossRef CAS.
-
(a) W.-F. Wang, T. Zhang and M. Shi, Organometallics, 2009, 28, 2640–2642 CrossRef CAS;
(b) J.-J. Jiang, D. Wang, W.-F. Wang, Z.-L. Yuan, M.-X. Zhao, F.-J. Wang and M. Shi, Tetrahedron: Asymmetry, 2010, 21, 2050–2054 CrossRef CAS.
- For diastereoselective umpolung allylations of aldehydes by using chiral borate or aldehydes, see:
(a) M. Vogt, S. Ceylan and A. Kirschning, Tetrahedron, 2010, 66, 6450–6456 CrossRef CAS; for palladium-catalyzed umpolung allylation reactions of ketones and imines developed in this laboratory, see:
(b) X.-C. Qiao, S.-F. Zhu and Q.-L. Zhou, Tetrahedron: Asymmetry, 2009, 20, 1254–1261 CrossRef CAS;
(c) X.-C. Qiao, S.-F. Zhu, W.-Q. Chen and Q.-L. Zhou, Tetrahedron: Asymmetry, 2010, 21, 1216–1220 CrossRef CAS.
- J.-H. Xie and Q.-L. Zhou, Acc. Chem. Res., 2008, 41, 581–593 CrossRef CAS.
- For recent examples, see:
(a) G. E. Keck, C. A. Wager, T. T. Wager, K. A. Savin, J. A. Covel, M. D. McLaws, D. Krishnamurthy and V. J. Cee, Angew. Chem., Int. Ed., 2001, 40, 231–234 CrossRef CAS;
(b) A. B. SmithIII, V. A. Doughty, C. Sfouggatakis, C. S. Bennett, J. Koyanagi and M. Takeuchi, Org. Lett., 2002, 4, 783–786 CrossRef;
(c) M. Inoue and M. Nakada, J. Am. Chem. Soc., 2007, 129, 4164–4165 CrossRef CAS.
- By using less sterically hindered phosphine ligand 1a both 1-phenyl allylic alcohol and 3-phenyl allylic alcohol underwent the allylation reaction with benzaldehyde under a similar reaction conditions, see ref. 8.
- For recent examples, see:
(a) D. R. Williams and K. G. Meyer, J. Am. Chem. Soc., 2001, 123, 765–766 CrossRef CAS;
(b) P. P. Reddy, K.-F. Yen and B.-J. Uang, J. Org. Chem., 2002, 67, 1034–1035 CrossRef CAS;
(c) K.-P. Chan and T.-P. Loh, Org. Lett., 2005, 7, 4491–4494 CrossRef CAS;
(d) N. Gogoi, J. Boruwa and N. C. Barua, Eur. J. Org. Chem., 2006, 1722–1725 CrossRef CAS;
(e) E. G. Bowen and D. J. Wardrop, J. Am. Chem. Soc., 2009, 131, 6062–6063 CrossRef CAS.
- M. Kimura, Y. Horino, R. Mukai, S. Tanaka and Y. Tamaru, J. Am. Chem. Soc., 2001, 123, 10401–10402 CrossRef CAS.
- For a review, see: K. J. Szabó, Chem.–Eur. J., 2004, 10, 5268–5275 Search PubMed.
Footnote |
† Electronic supplementary information (ESI) available: Detailed experimental procedures, the synthesis and analysis data of new ligands, the analysis data of ee values of products (PDF). See DOI: 10.1039/c0sc00645a |
|
This journal is © The Royal Society of Chemistry 2011 |
Click here to see how this site uses Cookies. View our privacy policy here.