DOI:
10.1039/C1RA00599E
(Paper)
RSC Adv., 2012,
2, 156-160
Received
16th August 2011
, Accepted 26th September 2011
First published on 1st November 2011
Abstract
Controlled drug delivery is highly desired for many medicinal cases where time and spatial dependency is required. The present paper provides a promising but also simple molecular architecture for photoinduced release of chemical compounds with primary amines based on one-and two-photon absorption. Silane coupling to the surface and biotin/streptavidin chemistry ensure the stability of the system.
Introduction
Medicinal products are most commonly administrated orally or through injection.1 Due to the wide range of conventional dosage and the requirement of passing through certain biological tissues both of these drug delivery methods can cause serious inconveniencies to patients that neither of these two methods can be applied to many highly potent drug candidates.1,2 Lately several new drug delivery systems have been developed using both physical and chemical methods.3–5 All the new methods aimed at releasing drugs at the desired time with the desired dose and even at the desired location. o-Nitrobenzyl alcohol derivatives are a group of well-studied photo-labile linkers. The photo-cleavage of this group of compounds requires no reagent other than light which gives little side-product during photocleavaging.6,7 In addition, this group of compounds has high compatibility with acid and base. Because of these advantages o-nitrobenzyl alcohol derivatives have been widely used as photo protecting groups in peptide, nucleotide and carbohydrate synthesis.6,8,9 Two-photon excitation requires high photon densities provided by two tightly focused NIR femtosecond lasers. When the photoreactive compound absorbs two photons simultaneously it will be excited as if it absorbed one photon of twice the energy (UV photon) as the actual exciting NIR photon.10,11 The resulting excitation will be confined to a (sub-)femtolitre focal volume with small aspect ratios.12 The wavelength used in two-photon excitation in the following work was 750 nm. Light at this wavelength causes limited damage to biological systems and can penetrate tissues on the order of hundreds of micrometres to centimetres.2 Two photon-excitation has been applied in cataract treatment.13 Recent studies show that two-photon excitation can induce photo-cleavage of coumarin carbamates14 and of the o-nitrobenzyl group.15,16 In the following work we combined photo-cleavable nitrobenzyl linkers (PC linker) and two-photon excitation towards developing a versatile drug delivery system. The molecule loaded on the surface in the system can be released by illumination at a wavelength of 750 nm. The amount of molecules released can be controlled by varying the amount of time and intensity of the irradiation. More importantly, this system can be applied to various proteins, peptides and small molecules bearing a primary amine.
Two structures containing the o-nitrobenzyl based PC linker were designed and constructed on the surfaces. The properties of controlled releasing of desired compounds with both one-photon and two-photon excitation were studied on these structures and their control substrates. The substrates were characterized using both ellipsometry and fluorescence microscopy (FM).
Results and discussion
SiO2 substrates were coated with (3-aminopropyl)triethoxysilane (APTES) while protected against moisture and oxygen. This step installed an active primary amine group on the surface. Commercially available N-hydrosuccinimide-photocleavable-longchain-biotin (NHS-PC-LCws-biotin), a compound, containing PC linker, can be attached to the surface by simply incubating the APTES coated substrates with the NHS-PC-LCws-biotin in phosphate buffer saline (PBS). Under a slightly basic aqueous environment the N-hydroxysuccinimide ester (NHS) group quickly reacted with the exposed primary amine group on the surface and left a biotin group exposed (Fig. 1a and 1b).17 Continuous UV-vis absorption spectra were recorded for NHS-PC-LCws-biotin in solution to monitor the change of this PC linker under the irradiation of 365 nm UV lamp (Fig. 1c) from 1 min to 120 min. UV irradiation induced the cleavage of the PC linker and generated a ketone. The resulting spectra showed that the absorption of the photo labile linker decreased at 273 nm and a new peak at 328 nm appeared. When the PC linker was desorbed from the surface upon UV irradiation one mole of photo labile linker would break into one mole of ketone and one mole of amine, and also release one mole of carbon dioxide.6 Utilizing the high affinity between biotin and streptavidin, Dylight 488 conjugated streptavidin was installed on the surface. This structure was demonstrated in Fig. 2a as substrate 1. To better observe the photocleavaging property of structure 1, a control compound was prepared using the commercially available compound sulfo-NHS-biotin instead of NHS-PC-LCws-biotin (Fig. 1b) to synthesize the control substrate 1 (1control). Irradiation of the substrate 1control should not induce any photocleavaging. Hence, no releasing of dye labeled streptavidin should be observed. The surface modification can be monitored by FM and ellipsometry. The ellipsometry measurements showed that after coating the substrate with structure 1 the organic layer on the silicon surface gained in thickness of between 7–15 nm. Silicon can quench the fluorescence from the dye located within the Förster distance of ≈5 nm. In structure 1 setup, where the dye was located slightly more than 5 nm away, the silicon wafer was still able to absorb a significant amount of fluorescence emitted from Dylight 488.
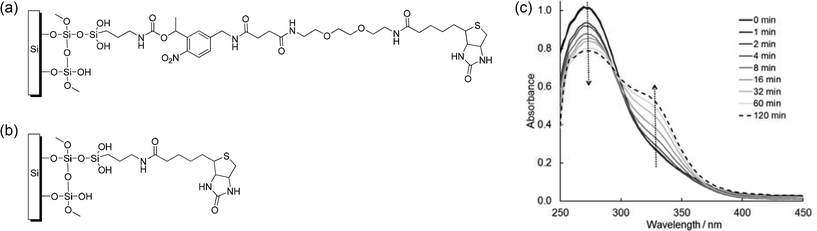 |
| Fig. 1 (a) Molecular structure of the surface modification with o-nitrobenzyl group. (b) Surface modification without nitrobenzyl group for control experiments. (c) Change of the UV-vis spectra of 5 mM NHS-PC-LCws-biotin in DMSO with exposure times from 0 min to 120 min with λ = 365 nm. | |
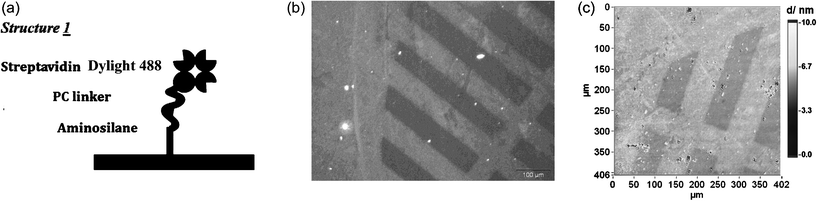 |
| Fig. 2 (a) Structure 1 for releasing streptavidin. (b) Surface patterned by single-photon excitation applying a copper mask. Fluorescence image (λex = 480 nm, t = 30 s) of surface modified with structure 1; irradiation at λ = 365 nm for 1 h at a distance of 4 cm results in shielded areas and areas with desorption of model compound. (c) Imaging ellipsometry gives a three-dimensional impression of the surface patterned by one-photon excitation (same surface as in 2b). | |
One-photon patterning was carried out by irradiating the substrates with a UV-LED lamp for 1.5 h at λ ≈ 365 nm. One drop of 1 mM semicarbazide hydrochloride water solution was added onto the top of the surface during irradiation to reduce the side-reaction. After the irradiation, the substrates were incubated in washing buffer (10% 20× SSC-buffer, 3.81 mM SDS and 453 μM Tween 20) for 30 min and then rinsed with pure water before further measurement. The photo labile linker was broken during the irradiation and the protein Dylight 488 labeled streptavidin as the top layer attached to the surface was released and washed away in the washing step. As a result, a darker image should be observed under FM. To better observe this effect a copper mask with a striped pattern was used to cover the substrates during irradiation. A patterned fluorescence image was observed after the irradiation of structure 1 decorated substrate (Fig. 2b). However, some patterned images were observed on substrate 1control, even though with a weaker contrast. We attributed this phenomenon to photo bleaching.18 Imaging ellipsometry, as label-free technology, was employed to study the photo-cleavaging effect. Using this technology the photobleaching effect can be excluded. The image resulting from imaging ellipsometry showed a significant decrease of layer thickness due to the releasing of streptavidin (Fig. 2c).
For the two-photon cleavaging we made use of a pulsed titanium-sapphire laser to irradiate the surface followed by a washing step as in the one-photon excitation experiment. The laser focus was scanned on the decorated surface to form 30 μm × 30 μm squared patches. Two-dimensional 9 × 9 arrays were formed on the decorated surfaces by varying the incident laser power along the x-axis from 66 mW to 45 mW with steps of 2.6 mW and by varying the scanning speed along the y-axis from 200 μm s−1 to 840 μm s−1 with steps of 80 μm s−1.
Structure 1 was easy to prepare and responded to both one-photon and two-photon excitation. Being a drug delivery system, structure 1 had many disadvantages. First, structure 1 lacked versatility. Only compounds that had high affinity with biotin could be utilized in this structure for photo-releasing. Moreover, the exposed streptavidin was released with a long tail that came from the photo-labile linker. To improve the system structure 2 was designed and synthesized (Fig. 3a). To synthesize structure 2, NHS-PC-LCws-biotin was incubated with Alexa 555 conjugated ovalbumin to form the complex through the linkage between NHS group and amine groups on the protein. This complex was then installed on the sulfo-NHS-biotin and the streptavidin coated substrate through the binding of biotin and streptavidin. Control compound for structure 2 (2control) was also prepared by replacing the NHS-PC-LCws-biotin with sulfo-NHS-biotin.
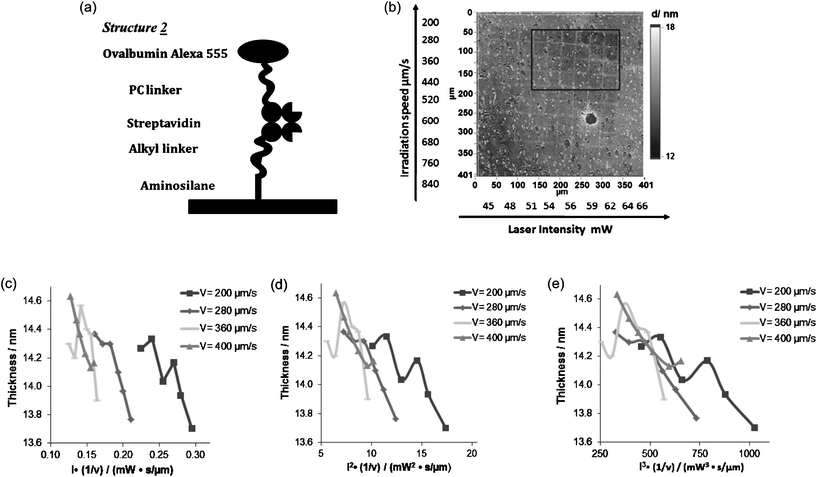 |
| Fig. 3 (a) Structure 2 for releasing model drug with primary amino group. (b) Ellipsometry image of structure 2 coated surface after two-photon excitation. (c)–(e) Plot of the release of Ovalbumin Alexa 555 and the dose of laser assuming a one-, two- and three photon excitation | |
Compared to structure 1, structure 2 had several improvements. First, in structure 2 the dye was further away from the silicon surface. Ellipsometry showed that the thickness of the organic layer was increased to 11–18 nm in structure 2 compared to 7–15 nm in structure 1. The fluorescence quenching was proportional to the distance between the dye and silicon surface. As a result, structure 2 provided a brighter fluorescence image under FM (data not shown). More importantly, structure 2 greatly improved the versatility of the photo-releasing system. The chemical compound was connected to the PC linker through NHS coupling, compared to structure 1 which was connected to biotin. Hence a wide range of proteins and drug like molecules that have primary amines would be able to apply in this system. Finally, because of the change of connectivity of the structure the released chemical compound completely retained the original structure.
One-photon and two-photon excitation were carried out on substrates 2 and 2control. Same patterned images were recorded for both substrates by FM after both one-photon and two-photon excitation indicating photo bleaching. Using imaging ellipsometry, patterned images were recorded in a gradient fashion as demonstrated in the modeled ellipsometry image only on substrate 2 (Fig. 3b). No thickness change was measured in the control decorated substrates.
In Fig. 3b the darkness was proportional to the thickness of the organic layer. The darker the area the thinner the area was. The thickness of each area was then modeled and plotted. Due to the background noise only the squares in the black square area (Fig. 3b) were analysed (4 rows × 6 lines). In the analysis, the thickness (t) of the organic layer was assumed to be proportional to the fraction of deprotection θdep. As discussed in Jonas and Kreiter's paper, this relation had unknown factors but was well defined.14 If the photo-cleavaging process was one-photon absorption then the θdep should be dependent on the intensity of the laser (I). If the photocleavaging process was n-photon absorption then the θdep should be dependent on the n-th power of the intensity of the laser.15 The thicknesses of the organic layers (t) were plotted against the laser properties: In (1/v), where v is the laser scanning speed. We were observing the collapsing of curves by varying n from 1 to 3 (Fig. 3c–3e). The better collapsing of the curves indicated the better consistency of the relationship between the thickness of the organic layer and the laser property. Based on our observations when n = 2 and n = 3 the curves showed the best consistency.
Experimental section
Polished silicon wafers (Siltronic, cut to an average size 20 mm × 15 mm, SiO2 thickness 300 μm ± 15 μm) and glass slides (Menzel, 18 mm × 18 mm × 1 mm) were first cleaned in 1% alkaline cuvette cleaner solution (Fluka). The solution was kept at 50 °C for 30 min. The substrates were then rinsed with ultrapure water and dried with air stream. To activate the surfaces the substrates were immersed in a mixture of 3
:
1 (30 ml/10 ml) ammonia (25%, Merck Germany) and hydrogen peroxide (30%, Sigma-Aldrich). The mixture was heated to 90 °C and was kept at this temperature for 30 min. After rinsing with ultrapure water and drying with air stream, the substrates were immersed in the mixture of 3 ml dry toluene (HPLC grade dried over molecular sieve 4 Å, Sigma-Aldrich), 20 μltriethylamine (Sigma-Aldrich) and 20 μl (3-aminopropyl)triethoxysilane (99%, Sigma-Aldrich) under argon atmosphere for 20 h. The substrates were then cleaned by first storing in toluene for 1 h and then in chloroform (spectrum grade, Sigma-Aldrich) for 1 h. After that the substrates were blown dry. The completion of silanization on the silicon surface was confirmed by the change of thickness of the organic layer on the surface determined by ellipsometry.
Surface modification
Structure 1.
For each substrate 2.5 μl 1 mg/1 ml NHS-PC-LCws-biotin/anhydrous DMF (1.3 mM, Ambergen, USA) stock was diluted in 3 ml phosphate buffer saline (PBS, pH 7.4, Sigma-Aldrich) to obtain 1 μM dilution. Cleaned and silanized substrates were immersed in the dilution for 2 h. The substrates were then rinsed with ultrapure water and dried with air flow. Dylight 488 conjugated streptavidin (Thermo Fisher Scientific) was coated on the substrates in a similar way by immersing the substrates in 3 ml 56 nM protein PBS dilution for 2 h.
The control substrates were prepared in the same way using 1 mg/1 ml sulfo-NHS-biotin/DMSO stock (2.26 mM, Thermo Scientific) PBS dilution instead of NHS-PS-LCws-biotin.
Structure 2.
Cleaned and silanized substrates were first immersed in 1 mg/1 ml sulfo-NHS-biotin/DMSO stock PBS solution for 2 h. For each substrate 2.5 μl sulfo-NHS-biotin stock solution was used. Then the substrates were immersed in 55 nM streptavidin PBS dilutions for 2 h. 5 μl 1 mg/1 ml NHS-PS-LCws-biotin/anhydrous DMF and 50 μl 1 mg/1 ml ovalbumin Alexa 555 (7 μM) stock was mixed with 3 ml PBS solution for each substrate. The solution was allowed to react for 2 h so that NHS-PC-LCws-biotin could attach to ovalbumin Alexa 555 through cross linking. The solution was then incubated with sulfo-NHS-biotin and a streptavidin coated substrate for 2 h. The last two steps were repeated to increase the yield.
The control substrates were prepared in the same way using 55 nM sulfo-NHS-biotin (1 mg/1 ml DMSO stock, 2.26 mM, Thermo Scientific) PBS solution instead of a NHS-PS-LCws-biotin solution.
UV-vis spectra were recorded on an Implen NanoPhotometer (Munich, Germany). 3 μl solutions were used for each scan.
UV irradiation
A UV-LED (90 mW, Seoul Semiconductor, South Korea) at 365 nm was used to cleave the photo-labile linker. The irradiation was carried out at a distance of 4 cm for 1.5 h. To characterize the photorelease, copper masks (Athene Sjorstrand, 75 μm and 150 μm wide slots) were used to cover the substrates to create patterns.
After one-photon excitation, the substrates were stored in washing buffer (10% 20× SSC-buffer, 3.81 mM SDS and 453 μM Tween 20) for 1 h to wash away cleaved chemicals. The substrates were then rinsed with pure water and dried with air stream.
Two-photon excitation
The two-photon excitation cleavage was done using a titanium-sapphire laser (Tsunami, Newport Spectra-Physics, Germany). The laser wavelength, pulse duration, and repetition rate were 750 nm, 100–120 fs, and 80 MHz, respectively. The laser was focused onto the functionalized surface by an objective lens (63 ×, NA 0.75, Zeiss). Ultraprecise linear-motor driven stages (ABL 10 100 Air Bearing Stage, Aerotech Inc., Pittsburgh PA) were used to move the laser focus according to a computer model in x-, y-, and z-directions. A rectangular box, 30 μm × 30 μm × 10 μm, was used as a model. The z-extension of the box was necessary to compensate for minor substrate tilting and to ensure that the laser focus hits the surface. Squared patterns were generated by irradiating a 9 × 9 array of boxes. Typical writing velocities and laser powers were in the range of 1.000 μm s−1 and 50 mW, respectively.
The fluorescence microscope used was an Olympus IX 81.4 × and 10 × objectives were used to observe the pattern. For observing the Dylight 488 dye, a U-MNB filter was used (excitation filter: 470–490 nm, emission filter: 520 IF, dichromatic mirror: 500 nm). For observing the Alexa 555, a U-MWIY filter was employed (excitation filter: 545–580 nm, emission filter: 610 IF, dichromatic mirror: 600 nm).
A spectroscopic imaging ellipsometer with a laser emitting at λ = 532 nm (Nanofilm EP3, Accurion, Germany) was employed to measure the organic layer thickness on the silicon wafers. For each measurement a four zone nulling (compensator position fixed at 45°) at three different coordinate dependent points on the sample surfaces was done. An angle of incidence of 42° was selected. The parameter settings used for optical modeling were: n(Si) = 4.1653; k(Si) = 0.049; n(SiO2) = 1.4605; k(SiO2) = 0; n(silane) = 1.45; k(silane) = 0 (n is the refraction index and k is the extinction coefficient). After coupling reactions the same values for n(silane) and k(silane) were assumed to be standard for organic materials. Additional to optical thickness, measurements of the patterned surfaces were characterized by imaging ellipsometry. The integrated CCD camera has a resolution of 768 × 572 pixels, but only 600 × 400 pixels are effective usable for imaging. Profiles were made over a range of about 400 × 400 μm2 at 10 × magnification and a maximum possible lateral resolution of 2 μm.
Conclusions
The described surface architecture enables a simple way for the photolabile immobilization of chemical compounds. Silane chemistry and the biotin/streptavidin system are well known for their stability. The surface architecture can be applied for all applications where the photorelease of chemical compounds bearing a primary amine is desired. In addition to the photocleavage in the UV spectral range, the application of two-photon absorption in the near infrared wavelength range enables mild conditions for the drug release. When cultivating biological cells on a surface substrate this method may be favourable since the cells are not affected by the UV light. Thinking about drug release in tissue the excitation in the NIR is advantageous compared to UV light due to the higher penetration depth and the focussing into a very small volume where the two-photon excitation takes place. Finally, for investigating the photocleavable surface architecture imaging ellipsometry suits well for the analysis since no reporter molecule is necessary.
Acknowledgements
We acknowledge financial support from the German Academic Exchange Service (DAAD) in the framework of the RISE Professional Programme.
References
-
D. Karsa and R. Stephenson, ed., Chemical aspects of drug delivery systems, The Royal Society of Chemistry, Cambridge, 1996 Search PubMed.
- B. P. Timko, T. Dvir and D. S. Kohane, Adv. Mater., 2010, 22, 4925–4943 CrossRef CAS.
- W. N. Charman, H.-K. Chan, B. C. Finnin and S. A. Charman, Drug Dev. Res., 1999, 46, 316–327 CrossRef CAS.
- R. Haag, Angew. Chem., Int. Ed., 2004, 43, 278–282 CrossRef CAS.
- J. T. Santini Jr., A. C. Richards, R. Scheidt, M. J. Cima and R. Langer, Angew. Chem., Int. Ed., 2000, 39, 2396–2407 CrossRef.
- C. G. Bochet, J. Chem. Soc., Perkin Transactions, 2002, 1, 125–142 Search PubMed.
- B. Chandra, R. Subramaniam, S. Mallik and D. K. Srivastava, Org. Biomol. Chem., 2006, 4, 1730–1740 CAS.
- A. Hasan, K.-P. Stengele, H. Giegrich, P. Cornwell, K. R. Isham, R. A. Sachleben, W. Pfleiderer and R. S. Foote, Tetrahedron, 1997, 53, 4247–4264 CrossRef CAS.
- M. C. Pirrung, Y. R. Lee, K. Park and J. B. Springer, J. Org. Chem., 1999, 64, 5042–5047 CrossRef CAS.
- K. Kamada, K. Ohta, T. Kubo, A. Shimizu, Y. Morita, K. Nakasuji, R. Kishi, S. Ohta, S. Furukawa, H. Takahashi and M. Nakano, Angew. Chem., Int. Ed., 2007, 46, 3544–3546 CrossRef CAS.
- M. Oheim, D. J. Michael, M. Geisbauer, D. Madsen and R. H. Chow, Adv. Drug Delivery Rev., 2006, 58, 788–808 CrossRef CAS.
- W. Denk, J. H. Strickler and W. W. Webb, Science, 1990, 248, 73–76 CAS.
- H.-C. Kim, S. Härtner, M. Béhe, T. M. Behr and N. A. Hampp, J. Biomed. Opt., 2006, 11, 034024 CrossRef.
- R. G. Wylie and M. S. Shoichet, J. Mater. Chem., 2008, 18, 2716–2721 RSC.
- M. Álvarez, A. Best, S. Pradhan-Kadam, K. Koynov, U. Jonas and M. Kreiter, Adv. Mater., 2008, 20, 1–5 CrossRef.
- S. Kantevari, C. J. Hoang, J. Ogrodnik, M. Egger, E. Niggli and G. C. R. Ellis-Davies, ChemBioChem, 2006, 7, 174–180 CrossRef CAS.
- G. W. Cline and S. B. Hanna, J. Org. Chem., 1988, 53, 3583–3586 CrossRef CAS.
- G. H. Patterson and D. W. Piston, Biophys. J., 2000, 78, 2159–2162 CrossRef CAS.
|
This journal is © The Royal Society of Chemistry 2012 |
Click here to see how this site uses Cookies. View our privacy policy here.