DOI:
10.1039/C1RA00922B
(Review Article)
RSC Adv., 2012,
2, 59-63
Recent advances in the development of dental composite resins
Received
20th October 2011
, Accepted 10th November 2011
First published on 23rd November 2011
Abstract
Dental composite resins are often favoured as direct dental restorative material due to their aesthetic quality, and they are increasingly replacing the controversial mercury-based amalgams. However, the commonly used dental composite resins present several important drawbacks, most importantly polymerisation shrinkage and the possibility of potentially toxic chemicals such as bisphenol A leaching out of the fillings. These problems have led to great interest in the development of improved composite resins. This review discusses the advantages and problems of currently-used resins, then focuses on recent developments, outlining several strategies aimed at improving the properties of dental resins. Finally, this review highlights the use of natural compounds for the development of dental resins, which is an emerging trend in the design of materials for bio-medical applications where biocompatibility is essential.
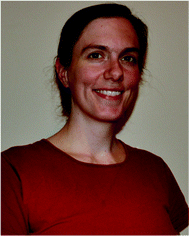 Christine Lavigueur | Christine Lavigueur received her BSc in chemistry with Honours from McGill University in Canada in 2003, and obtained her PhD in chemistry from Simon Fraser University in Canada in 2008 from the group of Prof. Williams where she studied discotic liquid crystals. From 2008 to 2011, she worked as a postdoctoral fellow at Radboud University Nijmegen (Netherlands) with Profs. Nolte and Cornelissen and at Université de Montréal with Prof. Zhu on the self-assembly of biohybrid and thermoresponsive compounds and on biomaterials based on natural compounds. Her research interests span all areas of organic materials chemistry. |
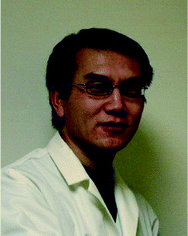 X. X. Zhu | X. X. Zhu received his BSc degree in chemistry from Nankai University in China, and his PhD degree from McGill University in Canada. After postdoctoral work at CNAM, France and the University of Toronto, he joined the Chemistry Department of Université de Montréal in 1992, where he is now a professor and holds the Canada Research Chair in polymeric biomaterials. He and his group make use of natural compounds such as bile acids in the preparation of polymers for use in biomedical and pharmaceutical applications. He is author of over 180 research publications and several patents and book chapters. |
Introduction
Direct filling materials, which can be applied in paste form and hardened in the tooth, allow easy dental restoration to replace damaged tissue and rebuild tooth morphology, thus restoring integrity and function. Amalgams, composed of mercury and metals such as silver, tin or copper, have been used as direct filling materials for more than 150 years.1 They have been among the most widely used materials for dental fillings since the early twentieth century but controversy about their toxicity remains,2,3 and they have been banned in some European countries.4
Amalgams are increasingly being replaced by aesthetic restorative materials, whose colour mimics enamel. Composite resins that can be cured using visible light are the most widely used material for direct aesthetic restoration. They are primarily constituted of an inorganic filler, which imparts rigidity, hardness and strength to the filling, and an organic resin matrix, which provides sufficient fluidity for easy application, and allows subsequent polymerisation for rapid setting of the filling. The filler and matrix are bonded together by a silane coupling agent.5
A separate binding agent is used to directly attach the composite resin filling to the dentine or enamel. This provides an additional advantage compared to amalgam fillings, which are retained by undercuts that require the removal of healthy tooth tissue.6
Other types of aesthetic direct fillings have been developed, such as compomers and glass ionomers, which are capable of slowly releasing fluoride.7–9 However, composite resins are the most commonly used direct aesthetic restorative material.
This review will focus on the polymer matrix component of composite resins, first briefly presenting currently used resins before discussing recent developments and new trends in the design of monomers for dental composite resins.
Currently-used resins
Currently used formulations for light-activated composite resins are complex mixtures including many components. In addition to the three main constituents, the monomers, inorganic fillers, and bonding agents, they also include a photo-initiator (and often a co-initiator) to enable photopolymerisation of the matrix, stabilisers to increase the shelf-life of the uncured resin, and pigments to better match the colour of the teeth. The fillers can be made of a variety of materials such as ground quartz, fused silica, or silicate glasses, and can be of different sizes, from a few nanometres to a few micrometres. The polymer matrix itself is typically constituted of a mixture of different dimethacrylate monomers, in order to impart the desired properties to the resulting resin.1,5,6,10
Common monomers
While a fairly wide variety of monomers are currently used in composite resins, three occupy a dominant place in the market: BisGMA (bisphenol A diglycidyldimethacrylate), UDMA (urethane dimethacrylate), and TEGDMA (tri-ethylene glycol dimethacrylate); they are shown in Fig. 1. Most other monomers are variations on these structures.5,11
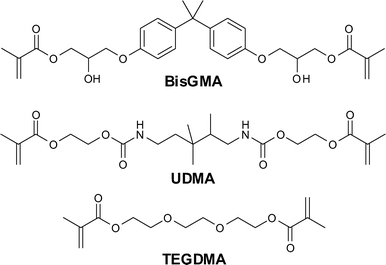 |
| Fig. 1 Structure of the most commonly used monomers in dental composite resins. | |
BisGMA is a high molecular weight monomer that can form strong, stiff resins. It is however very viscous, hence only a small amount of inorganic fillers can be added while still maintaining a paste consistency suitable for direct application. Therefore, BisGMA is combined with lower viscosity monomers, such as the low molecular weight TEGDMA, to obtain a more fluid monomer mixture to which more filler particles can be added. The presence of TEGDMA also helps increase cross-linking density in the resin. UDMA has a lower viscosity than BisGMA, it can therefore be used on its own, but is also often combined with other monomers to improve overall performance.5,11,12
Advantages and problems
Composite resins present several advantages over amalgam. They are particularly favoured for anterior teeth, where aesthetics are more important. They also allow more healthy tooth tissue to be preserved since composite resins can be bonded directly to the tooth, rather than being maintained in place by undercuts.
Arguably the main problem associated with composite resins is polymerisation shrinkage, which occurs because the monomers are brought closer together when they form covalent bonds during polymerisation. Shrinkage can lead to the formation of a gap between the filling and the tooth, allowing leakage and the development of secondary caries. It can also cause the appearance of micro-cracks in the filling, and apply potentially damaging stress to the tooth. The ultimate consequence of polymerisation shrinkage is a reduction of the service life of composite resin restorations.5,13–15
The thermal and mechanical properties of composite resins largely depend on the inorganic fillers, but the polymer matrix can also be designed to improve properties, for instance by increasing the degree of polymerisation and the cross-linking density. In the early days of composite resins, wear of the polymer matrix leading to release of large filler particles was a major issue, particularly for posterior restorations that bear more stress during mastication. Advances in composite resins, including the use of smaller filler particles, have greatly decreased wear, to the point that it is no longer considered to be a major problem.13,16,17
One of the motivations to replace amalgam by other materials is to avoid the use of potentially toxic mercury. However, composite resins can leach unreacted monomers as well as decomposition products, many of which are toxic. In particular, BisGMA, which incorporates a bisphenol A group, is known to be toxic. There are also a few known cases of allergic reactions triggered by composite resins. Overall, the biocompatibility of composite resins is considered sufficiently good for use, but further improvements would be possible.10,18,19
Recent developments
Most current research efforts in the field of dental resins are directed towards reducing polymerisation shrinkage. Other important goals are to improve the biocompatibility and the mechanical properties of the composite resins. The main strategies used to address these needs are discussed below.
Most polymerisations result in at least some shrinkage of the material due to the creation of new bonds bringing the atoms closer together, from a van der Waals distance to a covalent distance. From the stand point of dental resins, this shrinkage is a clearly undesirable property. The use of ring-opening polymerisation (ROP) could help circumvent this problem, since the polymerisation reaction involves the breaking of a bond for every new bond formed.
Mixtures of epoxy monomers and polyols are among the earlier examples of the use of ROP for visible light-cured dental resins.20 The inclusion of polyols allows a faster set with high conversion and crosslink density, leading to resins with good mechanical properties. The resulting materials exhibited less shrinkage than the traditional resins, as expected with ROP. However, the polymers displayed a greater water uptake, which limits the long-term strength of the composites.
Bicycloacrylates, which can undergo radical ring-opening polymerisation, have also been investigated for use in dental resins.21,22 An example of a typical monomer is shown in Fig. 2a. The monomers are quite stable, even in the presence of humidity or acidic and basic impurities, they show good reactivity leading to the formation of high molecular weight polymers, and they have low cytotoxicity. They also successfully decrease shrinkage compared to commonly used monomers.
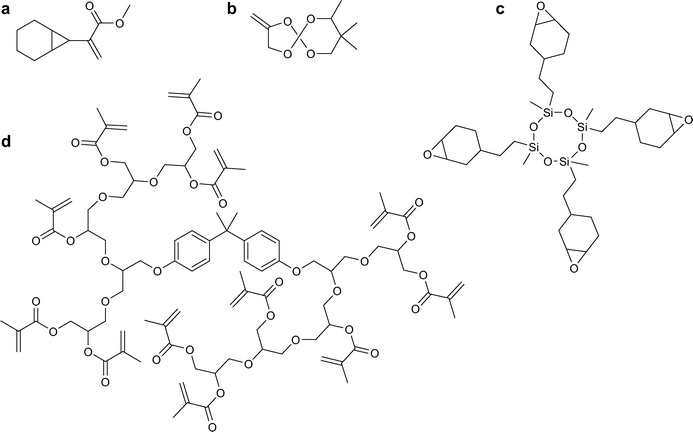 |
| Fig. 2 Examples of dental resin monomers used for ring-opening polymerisation: a) a bicycloacrylate;21 b) a spiro orthocarbonate;24 c) silorane;25 and of a hyperbranched monomer.26 | |
Spiro orthocarbonates (SOCs, example in Fig. 2b) are interesting candidates to reduce shrinkage because they can undergo double ring-opening polymerisation, with two bonds broken for every new bond created, leading to volume expansion upon polymerisation.23,24 However, they cannot be used on their own because of poor curing characteristics and insufficient mechanical strength. Promising results have been obtained by mixing SOCs with BisGMA or BisGMA derivatives, with a dramatic decrease in shrinkage and good mechanical properties, although the curing time needs to be increased.
A new type of monomer has been developed by combining siloxanes, used for their hydrophobicity, and oxiranes, which can undergo cationic ring-opening polymerisation. The resulting monomers, called siloranes (Fig. 2c), display low shrinkage and good mechanical properties.30
BisGMA is too viscous to be used on its own, it is therefore blended with the lower molecular weight TEGDMA, resulting in a fluid mixture to which filler particles can be added while still maintaining a texture that allows easy placement in the tooth. However, the presence of TEGDMA greatly increases polymerisation shrinkage. Indeed, the amount of shrinkage that occurs upon polymerisation is directly linked to the number of polymerisable group per weight of polymer, and TEGDMA is a low molecular weight monomer. Increasing the molecular weight of the monomers is however not a straightforward way to reduce shrinkage, since it also typically leads to an unacceptable increase in viscosity.
Hyperbranched polymers can present an appealing solution to this conundrum, since their viscosity does not increase linearly with molecular weight.31 A variety of hyperbranched monomers have been prepared for dental resin applications, with or without a bisphenol A core (Fig. 2d), and they have displayed promising properties: low viscosity, low shrinkage, and good mechanical strength.26,32 The two approaches described thus far have also been combined, with the development of hyperbranched polymers with epoxy end groups for ring-opening polymerisation.33
Other strategies
A variety of other strategies have been explored to improve the properties of resins, with particular focus on polymerisation shrinkage. One simple approach is to prepare analogues of BisGMA with improved properties. For example, as shown in Fig. 3, the hydroxyl groups were replaced with urethane27 or methoxy34 substituents to reduce the viscosity by avoiding the formation of strong hydrogen bonds, and methyl groups28,29 were added to the core to increase the molecular weight and reduce shrinkage. Fluorinated BisGMA derivatives36 and other fluorinated methacrylate monomers37 have been prepared in order to obtain inert hydrophobic polymers, although the mechanical strength of the resulting resins are usually slightly lower than those of conventional resins.
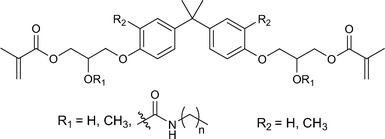 |
| Fig. 3 Examples of BisGMA analogues aimed at reducing viscosity and polymerisation shrinkage.27–29 | |
Another strategy to decrease polymerisation shrinkage is the use of liquid crystalline monomers that can remain in a mesophase within the uncured dental composite. The larger free volume caused by the partial order within the liquid crystalline phase helps alleviate shrinkage during polymerisation.38–41
The thiol-ene reaction has been proposed as an alternative to the more commonly-used radical polymerisation. Thiol-ene polymerisations proceed through a step growth mechanism, meaning that low molecular weight oligomers are produced in the initial stages of the reaction, which significantly delays gelation of the resin and reduces shrinkage. Furthermore, fewer unreacted monomers are present in the cured composites, thus reducing the risk that toxic compounds will leach from the fillings.42,43 A combined methacrylate-thiol-ene system has successfully combined these advantages with the good mechanical properties obtained in methacrylate-based resins.44
New trends
Recent developments have led to promising strategies to improve the performance of dental resins, but it remains difficult to simultaneously reduce polymerisation shrinkage, improve mechanical properties, and reduce toxicity. An emerging trend in the design of materials for bio-medical applications is to use natural compounds in order to improve biocompatibility. For example, the natural compound sesamin has been used as co-initiator in composite resins, as a replacement for conventional amines.45 Natural compounds could also be used as the basic scaffold in the development of monomers for dental resins.
Monomers based on natural compounds
Bile acids are a family of natural compounds that are present in the body, their structure is shown in Fig. 4a. They are facially amphiphilic, and can self-assemble in aqueous solution.46Cholic acid, one of the primary bile acids, aids in the digestion of fats in the body through the formation of mixed micelles.47Bile acids possess between one and three hydroxyl groups as well as a carboxylic acid moiety, offering up to four functionalisation sites, which have been exploited in the development of a variety of bile acid-based polymeric materials.48–55 The biocompatibility, rigid core, and ease of functionalisation of bile acids makes them very attractive candidates for the development of composite resins. To this end, bile acid derivatives containing up to four (meth)acrylic groups have been made by the simple esterification of the hydroxyl groups on the bile acids.56
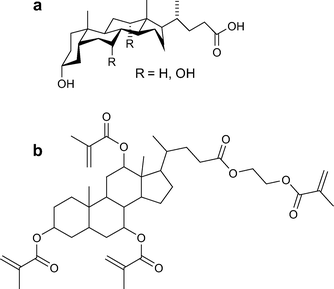 |
| Fig. 4 Structure of a) the naturally occurring bile acids and b) a cholic acid derivative bearing four methacrylate groups for use in dental resins.35 | |
The toxicity of composite resins can stem from uncured monomers leaching from the filling, or from (bio)degradation products. In order to evaluate bile acids as starting points for new dental resins, the cytotoxicity of four different bile acids as well as their simple ester and (meth)acrylate derivatives was measured and compared to the cytotoxicity of common dental monomers. Results showed that di-, tri-, and tetra-methacrylate derivatives of bile acids are significantly less cytotoxic than common dental monomers, most of them showing no effect on cell viability over their entire range of solubility. Potential final biodegradation products also displayed lower cytotoxicity than BisGMA or UDMA.57
Based on these promising results, multifunctional methacrylate derivatives of bile acids were prepared as cross-linkable monomers for dental composite resins, an example of which is shown in Fig. 4b. The obtained monomers have high molecular weights, which can reduce polymerisation shrinkage, and multiple polymerisable groups, which reduces the probability of having unreacted monomers that can leach out of the filling. Materials based on these new monomers displayed mechanical, thermal and physical properties comparable to those of materials based on common dental monomers, but with lower polymerisation shrinkage.35 These advantageous properties and the above-mentioned good biocompatibility make bile acid derivatives prime candidates for the development of new, improved dental resins. Further research in this direction is currently underway.
Conclusion
Currently-used composite resins provide an alternative to controversial mercury amalgams, but still suffer from a variety of problems, most notably polymerisation shrinkage and potential toxicity of leached compounds. Recent research has largely addressed these issues through a number of promising strategies, such as the use of ring-opening polymerisation, of hyperbranched monomers, or of improved BisGMA derivatives. Among them, the use of natural compounds as starting point for the development of monomers is particularly appealing. A series of multifunctional, high-molecular weight monomers derived from bile-acids have displayed promising physical properties and extremely low cytotoxicity. Further investigation of these prime candidates for dental composite resins are ongoing.
Acknowledgements
We thank NSERC and CIHR (CHRP program) and the Canada Research Chair program for financial support. CL thanks NSERC for a postdoctoral fellowship.
References
-
M. Gladwin and M. Bagby, Clinical Aspects of Dental Materials: Theory, Practice, and Cases, 3rd edn, Lippincott, Williams & Wilkins, Baltimore, 2009 Search PubMed.
-
D. Arenholt-Bindslev and P. Horsted-Bindslev, in Biocompatibility of Dental materials, ed. G. Schmalz and D. Arenholt-Bindslev, Springer-Verlag, Berlin, 2009, ch. 4, pp. 59–98 Search PubMed.
-
V. B. Dhuru, in Contemporary Dental Materials, ed. V. B. Dhuru, Oxford University Press, Oxford, 2004, ch. 14, pp. 126–137 Search PubMed.
- M. Bender, Mercury Policy Project Search PubMed , Oslo, January 3rd, 2008. Retreived from http://www.reuters.com/article/2008/01/03/idUS108558+03-Jan-2008+PRN20080103, September 28, 2011.
-
A. Prasad and N. Sarkar, in Contemporary Dental Materials, ed. V. B. Dhuru, Oxford University Press, Oxford, 2004 Search PubMed.
-
J. F. McCabe and A. W. G. Walls, Applied Dental Materials, ninth edn, Blackwell Publishing, Oxford, 2008 Search PubMed.
-
S. Mitra, in Contemporary Dental Materials, ed. V. B. Dhuru, Oxford University Press, Oxford, 2004, ch. 8, pp. 66–88 Search PubMed.
- J. W. Nicholson, Dent. Mater., 2007, 23, 615 CrossRef CAS.
- J. W. Nicholson, Mater. Technol., 2010, 25, 8 CAS.
-
G. Schmalz, in Biocompatibility of Dental materials, ed. G. Schmalz and D. Arenholt-Bindslev, Springer-Verlag, Berlin, 2009, ch. 5, pp. 99–137 Search PubMed.
- G. Vasudeva, Journal of the California Dental Association, 2009, 37, 389 Search PubMed.
- M.-H. Chen, J. Dent. Res., 2010, 89, 549 CrossRef CAS.
- G. J. Christensen, J. Am. Dent. Assoc., 2007, 138, 1487 Search PubMed.
- R. R. Braga, R. Y. Ballester and J. L. Ferracane, Dent. Mater., 2005, 21, 962 CrossRef CAS.
- J. L. Ferracane, Dent. Mater., 2005, 21, 36 CrossRef CAS.
- J. L. Ferracane, Dent. Mater., 2006, 22, 689 CrossRef CAS.
- A. R. Le Roux and N. Lachman, Journal of the South African Dental Association, 2007, 62, 340 Search PubMed.
- A. C. O. S. AFFAIRS, J. Am. Dent. Assoc., 2003, 134, 463 Search PubMed.
- A. Bakopoulou, T. Papadopoulos and P. Garefis, Int. J. Mol. Sci., 2009, 10, 3861 CrossRef CAS.
- D. A. Tilbrook, R. L. Clarke, N. E. Howle and M. Braden, Biomaterials, 2000, 21, 1743 CrossRef CAS.
- A. de Meijere, V. Bagutski, F. Zeuner, U. K. Fischer, V. Rheinberger and N. Moszner, Eur. J. Org. Chem., 2004, 3669 CrossRef CAS.
- N. Moszner, F. Zeuner, U. K. Fischer, V. Rheinberger, A. de Meijere and V. Bagutski, Macromol. Rapid Commun., 2003, 24, 269 CrossRef CAS.
- E. J. Moon, J. Y. Lee, C. K. Kim and B. H. Cho, J. Biomed. Mater. Res., Part B, 2005, 73B, 338 CrossRef CAS.
- S. H. Yoo, K. Park, J. Kim and C. K. Kim, Macromol. Res., 2011, 19, 27 CrossRef CAS.
- W. Weinmann, C. Thalacker and R. Guggenberger, Dent. Mater., 2005, 21, 68 CrossRef CAS.
- A. K. Tomasik, M. Biernat and P. G. Parzuchowski, Polimery, 2010, 55, 284 CAS.
- C. A. Khatri, J. W. Stansbury, C. R. Schultheisz and J. M. Antonucci, Dent. Mater., 2003, 19, 584 CrossRef CAS.
- L. U. Kim, J. W. Kim and C. K. Kim, Biomaterials, 2006, 7, 2680 CAS.
- Y. Kim, C. K. Kim, B. H. Cho, H. H. Son, C. M. Um and O. Y. Kim, J. Biomed. Mater. Res., 2004, 70B, 82 CrossRef CAS.
- N. Ilie, E. Jelen, T. Clementino-Luedemann and R. Hickel, Dent. Mater. J., 2007, 26, 149 CrossRef CAS.
- A. W. Bosman, H. M. Janssen and E. J. Meijer, Chem. Rev., 99, 1665 CrossRef CAS.
-
United-States Pat., US 2011/0045444 A1, 2011 Search PubMed.
- Y. Zhang, D. Zhang, C. Qin and J. Xu, Polym. Compos., 2009, 30, 176 CrossRef CAS.
- J. W. Kim, L. U. Kim, C. K. Kim, B. H. Cho and O. Y. Kim, Biomacromolecules, 2006, 7, 154 CrossRef CAS.
- M. A. Gauthier, Z. Zhang and X. X. Zhu, ACS Appl. Mater. Interfaces, 2009, 1, 824 CAS.
- J. W. Stansbury and J. M. Antonucci, Dent. Mater., 1999, 15, 166 CrossRef CAS.
- S. Kurata and N. Yamazaki, Dent. Mater. J., 2011, 30, 103 CrossRef CAS.
- E. J. Boland, D. L. Carnes, M. MacDougall, N. Satsangi, H. R. Rawls and B. K. Norling, J. Biomed. Mater. Res., Part B, 2006, 79B Search PubMed.
- T. Buruiana, V. Melinte, G. Costin and E. C. Buruiana, J. Polym. Sci., Part A: Polym. Chem., 2011, 49, 2615 CrossRef CAS.
- N. Satsangi, H. R. Rawls and B. K. Norling, J. Biomed. Mater. Res., 2004, 71B, 153 CrossRef CAS.
- N. Satsangi, H. R. Rawls and B. K. Norling, J. Biomed. Mater. Res., Part B, 2005, 74B, 706 CrossRef CAS.
- T. Y. Lee, J. A. Carioscia, Z. Smith and C. N. Bowman, Macromolecules, 2007, 40, 1473 CrossRef CAS.
- H. Lu, J. A. Carioscia, J. W. Stansbury and C. N. Bowman, Dent. Mater., 2005, 21, 1129 CrossRef CAS.
- N. B. Cramer, C. L. Couch, K. M. Schreck, J. A. Carioscia, J. E. Boulden, J. W. Stansbury and C. N. Bowman, Dent. Mater., 2010, 26, 21 CrossRef CAS.
- K. Wang, D. Yang, M. Xiao, X. Chen, F. Lu and J. Nie, Acta Biomater., 2009, 5, 2508 CrossRef CAS.
- D. Madenci and S. U. Egelhaaf, Curr. Opin. Colloid Interface Sci., 2010, 15, 109 CrossRef CAS.
- S. Mukhopadhyay and U. Maitra, Curr. Sci., 2007, 87, 1666 Search PubMed.
- W. Q. Chen, H. Wei, S. L. Li, J. Feng, J. Nie, X. Z. Zhang and R. X. Zhuo, Polymer, 2008, 49, 3965 CrossRef CAS.
- J. T. Luo, G. Giguère and X. X. Zhu, Biomacromolecules, 2009, 10, 900 CrossRef CAS.
- S. Strandman, F. Le Dévédec and X. X. Zhu, Macromol. Rapid Commun., 2011, 32, 1185 CrossRef CAS.
- T. Wan, Y. Liu, J. Q. Yu, S. Chen, F. Li, X. Z. Zhang, S. X. Cheng and R. X. Zhuo, J. Polym. Sci., Part A: Polym. Chem., 2006, 44, 6688 CrossRef CAS.
- T. Zou, S. L. Li, Z. Y. Hu, S. X. Cheng and R. X. Zhuo, J. Biomater. Sci., Polym. Ed., 2007, 18, 519 CrossRef CAS.
- H. L. Fu, T. Zou, S. X. Cheng, X. Z. Zhang and R. X. Zhuo, J. Tissue Eng. Regener. Med., 2007, 1, 368 CrossRef CAS.
- G. Giguère and X. X. Zhu, J. Polym. Sci., Part A: Polym. Chem., 2007, 45, 4173 CrossRef.
- G. Giguère and X. X. Zhu, Biomacromolecules, 2010, 11, 201 CrossRef.
- X. Hu, Z. Zhang, X. Zhang, Z. Li and X. X. Zhu, Steroids, 2005, 70, 531 CrossRef CAS.
- M. A. Gauthier, P. Simard, Z. Zhang and X. X. Zhu, J. R. Soc. Interface, 2007, 4, 1145 CrossRef CAS.
|
This journal is © The Royal Society of Chemistry 2012 |
Click here to see how this site uses Cookies. View our privacy policy here.