DOI:
10.1039/C2RA21533K
(Communication)
RSC Adv., 2012,
2, 10189-10194
Syntheses, crystal structures and photoluminescent properties of silver(I) and cadmium(II) coordination polymers constructed from flexible hexadentate di-Schiff base ligands†
Received
24th July 2012
, Accepted 31st August 2012
First published on 6th September 2012
Abstract
A novel flexible S2N4 di-Schiff base, 2,2′-((1,4-phenylenebis(methylene))bis(sulfanediyl))bis(N-(pyridin-2-ylmethylene)aniline (pbbpa), was synthesized and used to construct two Ag(I) and Cd(II) coordination polymers, namely [Ag4(pbbpa)(NO3)4]n (1) and [Cd2(pbbpa)(NO3)4]n (2), which exhibited complicated 3D frameworks, incorporating Ag–C bonds and a 2D 63-hcb net, respectively. Their thermal stabilities, UV-vis absorption and photoluminescent properties were also discussed.
1. Introduction
During the past decades, a great deal of effort has been devoted to the assembly of metal–organic frameworks (MOFs) with multidentate organic ligands, not only due to their novel structures and topologies, but also their appealing properties and potential applications in luminescence, magnetism and catalysis, gas sorption and separation.1 In contrast to rigid ligands, such as 4,4′-bipyridine and 4,4′-biphenyldicarboxylic acid, that show little or no conformational alteration when they coordinate with metal centers, flexible multidentate ligands can adopt different conformations and have more possible coordination modes according to the different geometric requirements of the metal ion.2 Recently, interesting structures constructed from flexible multidentate ligands have shown interesting rotaxane, catenane, and helicate characters.3 Among the multidentate ligands, Schiff base ligands have received much attention due to their multiple coordination sites with different coordination preferences.4 As we know, the shape, conformation and coordinating orientation of organic ligands are dominant in affecting the structures of the supramolecular aggregates. So the design and synthesis of new multifunctional ligands are important steps to the assembly of desired coordination compounds. Recently, we have been investigating the use of flexible ligands based on aromatic spacers linked to thioether-carboxylate arms to build coordination polymer arrays.5 These organic ligands favor modulable conformations (cis, trans, or any intermediate conformations between them),6 and would provide us with an alternative approach for the construction of frameworks with novel patterns that are not easily achievable with simple rigid linear ligands. Moreover, conformational flexibility and different binding modes could increase the possibility of forming supramolecular isomers.7
Inspired by the points mentioned above, we designed a novel hexadentate S-shaped di-Schiff base ligand which has two symmetric –CH2– double arms and two (pyridin-2-ylmethylene)aniline Schiff base arms linked by S donors. The –CH2– arms and the N and S donors endow this di-Schiff base ligand with flexible conformations and multiple coordination sites with different coordination preferences. Herein, we present the synthesis of this novel di-Schiff base ligand and explore its coordination chemistry towards Ag(I) and Cd(II) ions (Scheme 1).
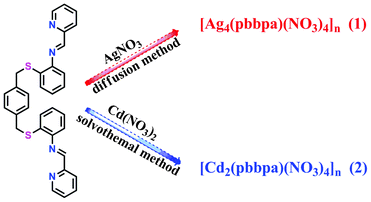 |
| Scheme 1 Synthetic procedures for Ag(I) and Cd(II) complexes based on the S2N4 di-schiff base ligand. | |
2. Experimental
2.1. Materials and methods
All the reagents and solvents employed were commercially available and used as received without further purification. Infrared spectra were recorded on a Nicolet AVATAT FT-IR330 spectrometer as KBr pellets in the frequency range 4000–400 cm−1. The elemental analyses (C, H, N content) were determined on a CE Instruments EA 1110 analyzer. Photoluminescence measurements were performed on a Hitachi F-7000 fluorescence spectrophotometer with solid powder on a 1 cm round quartz plate. TG curves were measured from 30 to 600 °C on a SDT Q600 instrument at a heating rate 10 °C min−1 under a N2 atmosphere (100 mL min−1). X-ray powder diffraction was performed on a Panalytical X-Pert Pro diffractometer with Cu-Kα radiation. UV-vis diffuse reflectance spectra were measured from 200 to 800 nm on a Carry 5000 UV-vis-NIR spectrophotometer.
2.2. Syntheses
2.2.1. Synthesis of 2,2′-((1,4-phenylenebis(methylene))bis(sulfanediyl))bis(N-(pyridin-2-ylmethylene)aniline (pbbpa).
Synthesis of (I): to a solution of KOH (1.3 g, 19.7 mmol) in methanol (40 mL) was added o-aminothiophenol (2.4 g, 19.2 mmol), followed by p-xylylene dibromide (2.0 g, 7.5 mmol) at room temperature with stirring. After stirring for 2 h, the solid obtained was filtered and washed with methanol (25 mL). Then the solid was washed with water (50 mL) and dried in vacuo to give I as a white solid (2.4 g, yield 90%). 1H NMR (300 MHz, CDCl3): 7.21 (q, 2H) , 7.13 (m, 2 H), 6.99 (s, 4 H), 6.69 (m, 4 H), 3.85 (s, 4 H).
Synthesis of (II): I (1.408 g, 4 mmol) and 2-pyridinecarboxaldehyde (0.94 g, 8.8 mmol) were stirred in an acetonitrile–chloroform (v
:
v = 9
:
1) solvent mixture at room temperature for 3 h. The solution was stirred but the reaction never went to completion as checked by TLC, which showed the presence of unreacted amine even after 3 h. A second portion of 2-pyridinecarboxaldehyde (0.32 g, 3 mmol) was added and the reactants were stirred for another 3 h. Then, the solvent was evaporated in vacuo, and the crude product was purified by recrystallization from chloroform–methanol (0.954 g, yield 45%.). 1H NMR (300 MHz, CDCl3): 8.83 (d, 2H), 8.72 (d, 2H), 7.96 (s, 2H), 7.86–7.89 (m, 2H), 7.46–7.55 (m, 2H), 7.17–7.21 (q, 2H), 7.07–7.13 (m, 2H), 7.02 (s, 4H), 6.60–6.70 (m, 4H), 3.85 (s, 4H).
2.2.2. [Ag4(pbbpa)(NO3)4]n (1).
A buffer layer of chloroform (6 mL) was carefully layered over a chloroform (4 mL) solution of pbbpa (15 mg, 0.028 mmol). Then a solution of AgNO3 (45 mg, 0.26 mmol) in methanol (4 mL) was layered over the buffer layer. The solution was allowed to diffuse in the dark at room temperature for four days to yield a large amount of yellow block crystals. Yield: ca. 48%. Anal. Calc. (found) for C16H13Ag2N4O6S: C, 31.76 (31.33); H, 2.17 (2.94); N, 9.26 (9.94)%. IR (KBr): v (cm−1) = 1729 (s), 1635 (w), 1595 (w), 1396 (s), 1291 (m), 776 (m), 525 (m).
2.2.3. [Cd2(pbbpa)(NO3)4]n (2).
Pbbpa (2.65 mg, 0.005 mmol) and Cd(NO3)2·4H2O (5.3 mg, 0.017 mmol) were dissolved in acetonitrile. Then the solution was sealed in a glass tube, slowly heated to 90 °C from room temperature in 300 min, kept at 90 °C for 4000 min, and then slowly cooled to 30 °C in 600 min. The yellow block crystals that formed were collected, washed with DMF, and dried in air. Yield: ca. 56%. Anal. Calc. (found) for C32H26Cd2N8O12S2: C, 38.30 (38.67); H, 2.61 (2.66); N, 11.17 (11.67)%. IR (KBr): v (cm−1) = 1636 (s), 1384 (s), 1197 (m), 1133 (m), 788 (s).
3. Results and discussion
3.1. X-ray crystallography
Single crystals of complexes 1–2 with appropriate dimensions were chosen under an optical microscope and mounted on a glass fiber for data collection. Single-crystal X-ray diffraction was performed using a Bruker Apex II CCD diffractometer equipped with a fine-focus sealed-tube X-ray source (Mo-Kα radiation, graphite monochromated). In both cases, the highest possible space group was chosen. Both structures were solved by direct methods using SHELXS-978 and refined on F2 by a full-matrix least-squares procedure with SHELXL-97.9 Atoms were located from iterative examination of difference F-maps following least squares refinements of the earlier models. Hydrogen atoms were placed in calculated positions and included as riding atoms with isotropic displacement parameters 1.2–1.5 times the Ueq of the attached C atoms. Both structures were examined using the Addsym subroutine of PLATON10 to ensure that no additional symmetry could be applied to the models. Pertinent crystallographic data collection and refinement parameters are collected in Table 1. Selected bond lengths and angles for 1 and 2 are collected in Table 2.
Empirical formula |
C16H13Ag2N4O6S |
C32H26Cd2N8O12S2 |
Formula weight |
605.10 |
1003.53 |
T (K) |
298(2) |
298(2) |
Crystal system |
Monoclinic |
Orthorhombic |
Space group |
P21/n |
Fdd2 |
a (Å) |
11.0150(12) |
35.5141(11) |
b (Å) |
9.2725(10) |
37.5405(12) |
c (Å) |
18.251(2) |
10.7672(3) |
α (°) |
90.00 |
90.00 |
β (°) |
99.2030(10) |
90.00 |
γ (°) |
90.00 |
90.00 |
Volume (Å3) |
1840.1(3) |
14355.0(8) |
Z |
4 |
16 |
ρ
calc (mg mm−3) |
2.184 |
1.857 |
μ (mm−1) |
2.286 |
1.377 |
F(000) |
1180.0 |
7968.0 |
Crystal size (mm) |
0.15 × 0.11 × 0.10 |
0.12 × 0.10 × 0.08 |
Index ranges |
−14 ≤ h ≤ 14, −12 ≤ k ≤ 12, −23 ≤ l ≤ 23 |
−34 ≤ h ≤ 39, −41 ≤ k ≤ 41, −9 ≤ l ≤ 11 |
Reflections collected |
15090 |
15155 |
Independent reflections |
4239 [R(int) = 0.0418] |
4519 [R(int) = 0.0216] |
Data/parameters |
4239/289 |
4519/505 |
Goodness-of-fit on F2 |
1.034 |
1.015 |
Final R indexes [I > = 2σ (I)] |
R
1 = 0.0481, wR2 = 0.1219 |
R
1 = 0.0166, wR2 = 0.0359 |
Final R indexes [all data] |
R
1 = 0.0737, wR2 = 0.1344 |
R
1 = 0.0179, wR2 = 0.0365 |
Largest diff. peak/hole/e (Å−3) |
1.26/−0.87 |
0.34/−0.30 |
Flack parameter |
N/A |
−0.006(14) |
Table 2 Selected bond lengths (Å) and angles (°) for 1 and 2
Complex 1
|
|
|
|
|
Ag1–N2 |
2.359 (4) |
Ag2–O4 |
2.384 (7) |
Ag1–N1 |
2.419 (5) |
Ag2–O1 |
2.466 (4) |
Ag1–O1i |
2.460 (4) |
Ag2–S1 |
2.4700 (13) |
Ag1–C10ii |
2.685 (6) |
Ag2–O5 |
2.500 (7) |
Ag1–S1 |
2.9227 (16) |
|
|
|
|
|
|
N2–Ag1–N1 |
69.79 (17) |
O1i–Ag1–S1 |
93.51 (10) |
N2–Ag1–O1i |
155.28 (16) |
C10ii–Ag1–S1 |
100.23 (15) |
N1–Ag1–O1i |
115.91 (18) |
O4–Ag2–O1 |
96.8 (2) |
N2–Ag1–C10ii |
108.51 (17) |
O4–Ag2–S1 |
140.5 (2) |
N1–Ag1–C10ii |
115.1 (2) |
O1–Ag2–S1 |
121.27 (11) |
O1i–Ag1–C10ii |
91.12 (17) |
O4–Ag2–O5 |
50.78 (19) |
|
|
|
|
Symmetry codes: (i) −x + 3/2, y + 1/2, −z + 3/2; (ii) −x + 1, −y, −z + 1. |
|
|
|
|
Complex 2
|
|
|
|
|
Cd1–N4i |
2.316 (3) |
Cd2–N1 |
2.332 (3) |
Cd1–O5 |
2.363 (3) |
Cd2–O3 |
2.357 (2) |
Cd1–N3i |
2.384 (3) |
Cd2–N2 |
2.373 (3) |
Cd1–O8 |
2.494 (4) |
Cd2–O6 |
2.382 (3) |
Cd1–O10 |
2.504 (3) |
Cd2–O11ii |
2.447 (3) |
Cd1–O11 |
2.532 (3) |
Cd2–O2 |
2.585 (3) |
Cd1–O7 |
2.535 (3) |
Cd2–S1 |
2.7374 (8) |
Cd1–S2i |
2.8669 (10) |
|
|
|
|
|
|
N4i–Cd1–O5 |
84.14 (10) |
O10–Cd1–S2i |
73.83 (6) |
N4i–Cd1–N3i |
70.27 (9) |
O11–Cd1–S2i |
117.46 (6) |
O5–Cd1–N3i |
129.00 (9) |
O7–Cd1–S2i |
157.30 (7) |
N4i–Cd1–O8 |
124.54 (10) |
N1–Cd2–O3 |
132.91 (9) |
O5–Cd1–O8 |
78.04 (10) |
N1–Cd2–N2 |
70.54 (9) |
N3i–Cd1–O8 |
152.46 (10) |
O3–Cd2–N2 |
149.94 (9) |
N4i–Cd1–O10 |
134.36 (10) |
N1–Cd2–O6 |
77.23 (10) |
O5–Cd1–O10 |
139.90 (9) |
O3–Cd2–O6 |
92.51 (10) |
N3i–Cd1–O10 |
82.88 (9) |
N2–Cd2–O6 |
113.53 (9) |
O8–Cd1–O10 |
70.48 (10) |
N1–Cd2–O11ii |
85.53 (9) |
N4i–Cd1–O11 |
87.34 (9) |
O3–Cd2–O11ii |
80.58 (10) |
O5–Cd1–O11 |
145.66 (9) |
N2–Cd2–O11ii |
83.72 (10) |
N3i–Cd1–O11 |
77.97 (9) |
O6–Cd2–O11ii |
149.07 (9) |
O8–Cd1–O11 |
79.70 (10) |
N1–Cd2–O2 |
81.87 (9) |
O10–Cd1–O11 |
50.46 (8) |
O3–Cd2–O2 |
51.11 (8) |
N4i–Cd1–O7 |
75.12 (9) |
N2–Cd2–O2 |
146.35 (10) |
O5–Cd1–O7 |
74.58 (9) |
O6–Cd2–O2 |
77.18 (10) |
N3i–Cd1–O7 |
134.07 (9) |
O11ii–Cd2–O2 |
75.08 (10) |
O8–Cd1–O7 |
49.60 (10) |
N1–Cd2–S1 |
131.65 (7) |
O10–Cd1–O7 |
101.49 (9) |
O3–Cd2–S1 |
94.09 (6) |
O11–Cd1–O7 |
71.09 (9) |
N2–Cd2–S1 |
71.05 (7) |
N4i–Cd1–S2i |
124.34 (7) |
O6–Cd2–S1 |
92.43 (7) |
|
|
|
|
Symmetry codes: (i) −x + 3/4, y + 1/4, z − 1/4; (ii) x, y, z + 1. |
3.1. Synthesis and general characterization
The condensation reaction of 2,2′-((1,4-phenylenebis(methylene))bis(sulfanediyl))dianiline with 2-pyridinecarboxaldehyde gave the pbbpa ligand in good yield. The ratio of CH3CN
:
CHCl3 used in the condensation reaction is very significant for the successful preparation of the pbbpa ligand (Scheme 2). Complexes 1 and 2 were synthesized by diffusion and solvothemal methods, respectively. The compositions of 1 and 2 were further deduced from X-ray single crystal diffraction, elemental analysis, and IR spectroscopy. The solid FT-IR spectra (Fig. S1, ESI†) of complexes 1 and 2 show the characteristic NO3− anion stretching bands at around 1400 and 1200 cm−1, indicating the NO3− anion displaying more than one binding mode,11 which is consistent with the results of the X-ray diffraction analysis.
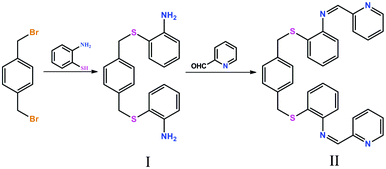 |
| Scheme 2 Synthetic procedures of the S2N4 di-Schiff base ligand. | |
3.2. Structure description of [Ag4(pbbpa)(NO3)4]n (1)
X-ray single-crystal diffraction analysis reveals that 1 is a complicated 3D framework. It crystallizes in the monoclinic crystal system with a space group of P21/n, and an asymmetric unit that contains two Ag(I) ions, half a pbbpa ligand and two NO3− ions. Ag1, O4, O5 and Ag1A, O4A, O5A are disordered over two sites with occupancies of 0.90
:
0.10. The pbbpa ligand is located on a crystallographic inversion center. As depicted in Fig. 1a, Ag1 and Ag2 are located in distorted square pyramidal (τ5 = 0.07)12a and tetrahedral (τ4 = 0.59)12b geometries, respectively. The coordination environment of Ag1 is completed by two N atoms from one (pyridin-2-ylmethylene)aniline arm, one S donor, one O atom of an NO3− ion and one C atom. Ag2 is coordinated by three O atoms from two NO3− ions and one S atom. The average Ag–O, Ag–N and Ag–S bond lengths are 2.452(5), 2.389(4) and 2.696(2) Å, respectively, comparable to those of related complexes.13 Notably, one slightly long Ag–C bond of 2.685(6) Å was observed, and the angle of Ag1–C10–Cg is 99.5(3)° (Cg is the centroid of the ring C7–C12), indicating the Ag–π coordination bond. This Ag–C distance is obviously below the margin of significant Ag–C interaction,14 and statistical analysis has demonstrated that this contact can be considered as weak bonding.15 Chen’s group reported a Ag(I) coordination polymer, namely, [Ag2(hmt)2(bna)]n (bna = 2,2′-dihydroxy-1,1′-binaphthalene-3,3′-dicarboxylate), in which the aromatic carboxylates bind to the Ag(I) atoms via a unique η2-coordination mode involving their aromatic rings with Ag–C distances of 2.633(9)–2.710(9) Å, in addition to the normal coordination modes utilizing their carboxyl groups.16 Although Ag–π interactions have a more recognized history in organometallic chemistry, and were well discussed in one comprehensive review by Munakata,17 coordinating Schiff base fragments to Ag(I) ions through a C atom as the π-donor has rarely been reported.
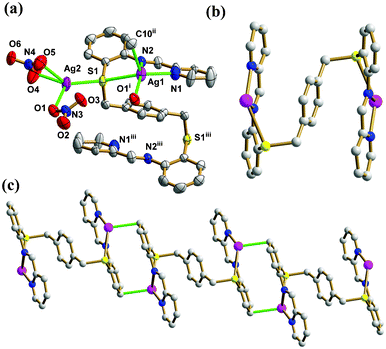 |
| Fig. 1 (a) Thermal ellipsoid (50%) plot of 1 showing the coordination environment of the Ag(I) ions. (b) The ball and stick view of the binuclear Ag2(pbbpa) subunit. (c) The ball and stick view of the 1D chain with the Ag–C bond highlighted in green (symmetry codes: (i) −x + 3/2, y + 1/2, −z + 3/2; (ii) −x + 1, −y, −z + 1.; (iii) −x + 2, −y, −z + 1). Color legend: Ag, purple; S, yellow; C, gray; O, red; N, blue. | |
The flexible pbbpa ligand is folded in an S-shape, such that the (pyridin-2-ylmethylene)aniline arms are positioned on different sides with respect to the central benzene ring, giving an overall anti-conformation (torsional angle across the benzene ring S1–C13⋯C13iii–S1iii = 180°, symmetry code: (iii) −x + 2, −y, −z + 1). In the anti-conformation of pbbpa, the pyridine and benzene rings of (pyridin-2-ylmethylene)aniline are twisted by 5.3(9)° and 36.8(5)° relative to the central benzene ring, respectively. The pyridine and benzene rings of (pyridin-2-ylmethylene)aniline are non-coplanar, with a dihedral angle of 35.8(3)°. The pbbpa ligand adopts a μ6-κ1N:κ1N:κ1C:κ2S:κ1N:κ1N:κ1C:κ2S binding mode, and binds two Ag(I) ions to form a binuclear subunit (Fig. 1b). The Ag–C bond plays an important role in extending the binuclear Ag(I) subunit into a 1D chain along the a axis (Fig. 1c). The two NO3− ions show monodentate bridging μ2-κ1O, κ1O and bidentate chelating μ1-κ1O:κ1O′ modes, respectively. The former type of NO3− ion bridges the 1D chain into a 2D sheet (Fig. 2a), which is further stacked into the complicated 3D framework by bridging S donors (Fig. 2b). The non-classic C–H⋯O hydrogen bonds, ranging from 3.066(7) to 3.335(9) Å, reinforce the 3D framework, in which the distances between aromatic rings are too long to take part in π⋯π interactions, with the shortest distance between centroids being 4.455(4) Å.
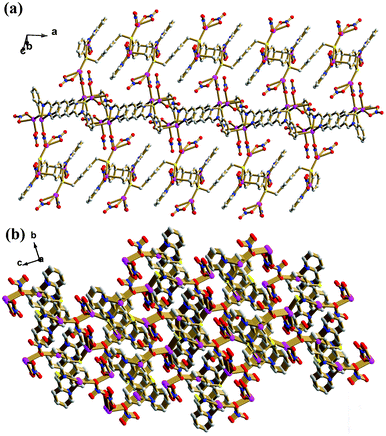 |
| Fig. 2 (a) The ball and stick view of the 2D sheet of 1. (b) The 3D framework of 1. Color legend: Ag, purple; S, yellow; C, gray; O, red; N, blue. | |
3.3. Structure description of [Cd2(pbbpa)(NO3)4]n (2)
When Ag(I) was replaced by the Cd(II) ion, we obtained complex 2 as a 2D 63-hcb net. X-ray single-crystal diffraction analysis revealed that 2 crystallizes in the orthorhombic crystal system with a space group of Fdd2, and its asymmetric unit contains two Cd(II) ions, one pbbpa ligand and four NO3− ions. As shown in Fig. 3a, Cd1 adopts an eight-coordinate square antiprismatic geometry which is completed by five O atoms from three different NO3− ions, two N atoms and one S atom from the same pbbpa ligand. Cd2 is seven-coordinate, with four O atoms from three different NO3− ions, two N atoms and one S atom from the same pbbpa ligand, giving a distorted pentagonal bipyramidal coordination geometry. The Cd–O, Cd–N and Cd–S bond lengths are in the range of 2.363(3)–2.585(3), 2.316(3)–2.384(3) and 2.7374(8)–2.8669(10) Å, respectively, comparable to similar complexes.18 The pbbpa ligand in 2 adopted a similar anti-conformation (torsion angle across the benzene ring S1–C13⋯C20–S2 = 165.88(19)°). In the anti-pbbpa ligand, the two pyridine rings of (pyridin-2-ylmethylene)aniline are twisted by 14.9(2)° and 2.9(2)° relative to the central benzene ring, respectively, while the twist angles between benzene rings of (pyridin-2-ylmethylene)aniline and the central benzene ring are 27.5(2)° and 35.3(2)° . The dihedral angles between pyridine and benzene rings of (pyridin-2-ylmethylene)aniline are 25.3(2)° and 32.4(2)°, respectively. Conversely, the pbbpa ligand only uses the N and S donors to coordinate a pair of Cd(II) ions to form a Cd2(pbbpa) subunit. In 2, four independent NO3− ions exhibit three different coordination modes; these are μ2-κ1O:κ1O:κ1O′ (monodentate bridging + bidentate chelating), μ1-κ1O:κ1O′ (bidentate chelating), and μ2-κ1O:κ1O′ (bidentate bridging), which play different roles in the construction of the resulting 2D network (Scheme S1, ESI†). The μ2-κ1O:κ1O:κ1O′ (monodentate bridging + bidentate chelating) NO3− ions link binuclear Cd(II) subunits to form the 1D chain (Fig. 3b). The bidentate chelating NO3− ions occupy the remaining coordination sites and do not participate in the extension of the 1D chain. Furthermore, the μ2-κ1O:κ1O′ (bidentate bridging) NO3− ions extend the 1D chain into a 2D sheet (Fig. 3c). To better understand the structure of 2, the topological analysis approach was employed. If all the nodes in one net have identical connectivity, then according to Wells it is a platonic uniform net, and can be represented by the symbol (n,p).19 In the sheet of 2, all the Cd(II) ions are 3-connecting, and the shortest circuit is a six-membered ring. So this 2D sheet can be simplified to a 63-hcb net. The packing of the 2D sheets into a 3D framework is due to intersheet C–H⋯O hydrogen bonds ranging from 2.922(4) to 3.455(4) Å.
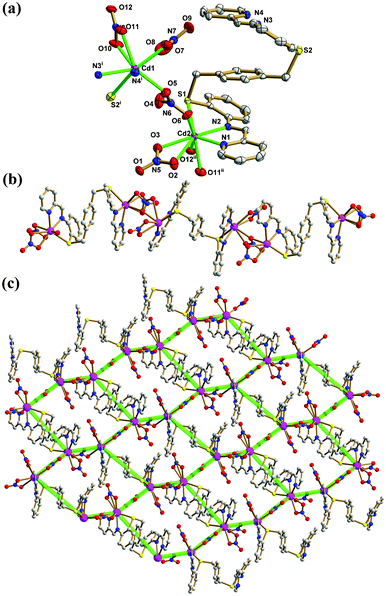 |
| Fig. 3 (a) Thermal ellipsoid (50%) plot of 2 showing the coordination environment of Cd(II) ions. (b) The ball and stick view of the 1D chain. (c) The 2D 63-hcb net (symmetry codes: (i) −x + 3/4, y + 1/4, z − 1/4; (ii) x, y, z + 1). Color legend: Cd, purple; S, yellow; C, gray; O, red; N, blue. | |
Thermogravimetric (TG) curves (Fig. S2, ESI†) were measured in a N2 atmosphere. For 1 and 2, no weight loss was observed before 164 and 247 °C, respectively. Further heating led to the collapse of the frameworks, accompanying the loss of the organic ligand pbbpa. The powder X-ray diffraction (PXRD) patterns of 1 and 2 are coincident with the simulated pattern derived from the X-ray single crystal data, implying that the bulk sample is same as the single crystal (Fig. 4). The dissimilarities in intensity may be due to the preferred orientation of the crystalline powder samples.
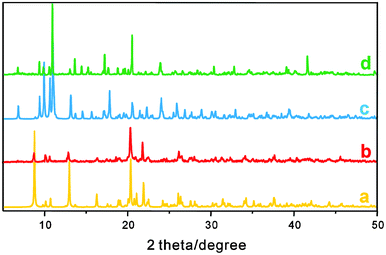 |
| Fig. 4 PXRD patterns for (a) simulated 1, (b) as-synthesized 1, (c) simulated 2, (d) as-synthesized 2. | |
3.5. UV-vis spectra and photoluminescence properties of 1 and 2
The absorption spectra of free ligand, 1 and 2 are shown in Fig. S3;† all complexes show similar high-energy absorption bands in the range of 200–500 nm, which are attributed to the intraligand π→π* transitions of the pbbpa ligand. As shown in Fig. S4,† the free ligand pbbpa displays photoluminescence, with an emission maximum at 414 nm (λex = 320 nm). It can be presumed that this peak originates from the π→π* transition. The emission spectra of 1 and 2 are shown in Fig. 5. The emission of 1 is very similar to that of the free ligand, so the luminescence of 1 can probably be assigned to the intraligand (IL) π→π* transition20 of the pbbpa ligand. Due to the d10 configuration of Cd(II) ion, the emission of 2 is neither metal-to-ligand charge transfer (MLCT) nor ligand-to-metal transfer (LMCT) in nature, since the Cd(II) ion is difficult to oxidize or reduce.21 Thus, it also should be assigned to the IL transition.
4. Conclusions
In this study, a flexible hexadentate S-shaped di-Schiff base was designed and synthesized by the condensation reaction of 2,2′-((1,4-phenylenebis(methylene))bis(sulfanediyl))dianiline and 2-pyridinecarboxaldehyde. Two silver(I) and cadmium(II) coordination polymers were successfully prepared by the reaction of this new ligand and various metal salts using different methods. This study demonstrates that the flexible double-armed Schiff base organic ligands are versatile building blocks in the construction of polymeric complexes. The thermal stabilities, UV absorption as well as emissive behaviors were also discussed. We are currently extending this result by preparing new flexible symmetric and asymmetric di-Schiff base ligands of this type containing different terminal coordination functional groups.
Acknowledgements
This work was supported by the National Natural Science Foundation of China (grant no. 21201110), the Independent Innovation Foundation of Shandong University (2011GN030), the Special Fund for Postdoctoral Innovation Program of Shandong Province (201101007 and 201102018) and the China Postdoctoral Science Foundation (2012M511492 and 2011M500725).
References
-
(a) T. T. Luo, H. C. Wu, Y. C. Jao, S. M. Huang, T. W. Tseng, Y. S. Wen, G. H. Lee, S. M. Peng and K. L. Lu, Angew. Chem., Int. Ed., 2009, 48, 9461 CrossRef CAS;
(b) G. Férey, Chem. Soc. Rev., 2008, 37, 191 RSC;
(c) R. A. Fisher and C. Wöll, Angew. Chem., Int. Ed., 2008, 47, 8164 CrossRef;
(d) S. Ma, D. Yuan, X. S. Wang and H. C. Zhou, Inorg. Chem., 2009, 48, 2072 CrossRef CAS;
(e) P. K. Thallapally, J. Tian, M. R. Kishan, C. A. Fernandez, S. J. Dalgarno, P. B. McGrail, J. E. Warren and J. L. Atwood, J. Am. Chem. Soc., 2008, 130, 16842 CrossRef CAS;
(f) L. Hou, Y. Y. Lin and X. M. Chen, Inorg. Chem., 2008, 47, 1346 CrossRef CAS;
(g) B. T. N. Pham, L. M. Lund and D. Song, Inorg. Chem., 2008, 47, 6329 CrossRef CAS;
(h) A. Lan, K. Li, H. Wu, D. H. Olson, T. J. Emge, W. Ki, M. Hong and J. Li, Angew. Chem., Int. Ed., 2009, 48, 2334 CrossRef CAS;
(i) K. L. Hu, M. Kuromo, Z. Wang and S. Gao, Chem.–Eur. J., 2009, 15, 12050 CrossRef CAS;
(j) J. Sun, F. N. Dai, W. B. Yuan, W. H. Bi, X. L. Zhao, W. M. Sun and D. F. Sun, Angew. Chem., Int. Ed., 2011, 50, 7061 CrossRef CAS;
(k) F. N. Dai, H. Y. He and D. F. Sun, J. Am. Chem. Soc., 2008, 130, 14064 CrossRef CAS;
(l) C. Zou, Z. J. Zhang, X. Xu, Q. H. Gong, J. Li and C. D. Wu, J. Am. Chem. Soc., 2012, 134, 87 CrossRef CAS;
(m) C. Yan, K. Li, S. C. Wei, H. P. Wang, L. Fu, M. Pan and C. Y. Su, J. Mater. Chem., 2012, 22, 9846 RSC;
(n) J. Zhang, S. M. Chen, R. A. Nieto, T. Wu, P. Y. Feng and X. H. Bu, Angew. Chem., Int. Ed., 2010, 49, 1267 CrossRef CAS;
(o) D. Q. Yuan, D. Zhao, D. J. Timmons and H. C. Zhou, Chem. Sci., 2011, 2, 103 RSC.
-
(a) P. L. Caradoc-Davies and L. R. Hanton, Chem. Commun., 2001, 1098 RSC;
(b) P. L. Caradoc-Davies, L. R. Hanton, J. M. Hodgkiss and M. D. Spicer, J. Chem. Soc., Dalton Trans., 2002, 1581 RSC;
(c) L. R. Hanton and K. Lee, J. Chem. Soc., Dalton Trans., 2000, 1161 RSC.
-
(a) A. Harada, Acc. Chem. Res., 2001, 34, 456 CrossRef CAS;
(b) C. Marchal, Y. Filinchuk, X. Y. Chen, D. Imbert and M. Mazzanti, Chem.–Eur. J., 2009, 15, 5273 CrossRef CAS;
(c) A. K. Bar, R. Chakrabarty and S. Munherjee, Inorg. Chem., 2009, 48, 10880 CrossRef CAS;
(d) J. Wang, Z. J. Lin, Y. C. Ou, Y. Shen, R. Herchel and M. L. Tong, Chem.–Eur. J., 2008, 14, 7218 CrossRef CAS.
-
(a) J. Doemer, J. C. Slootweg, F. Hupka, K. Lammertsma and F. E. Hahn, Angew. Chem., Int. Ed., 2010, 49, 6430 CrossRef CAS;
(b) W. Meng, B. Breiner, K. Rissanen, J. D. Thoburn, J. K. Clegg and J. R. Nitschke, Angew. Chem., Int. Ed., 2011, 50, 3479 CrossRef CAS;
(c) H. Mitsunuma and S. Matsunaga, Chem. Commun., 2011, 47, 469 RSC;
(d) C. Camp, V. Mougel, P. Horeglad, J. Pecaut and M. Mazzanti, J. Am. Chem. Soc., 2010, 132, 17374 CrossRef CAS;
(e) P. D. Frischmann, S. Guieu, R. Tabeshi and M. J. MacLachlan, J. Am. Chem. Soc., 2010, 132, 7668 CrossRef CAS.
-
(a) F. Dai, D. Sun and D. Sun, Cryst. Growth Des., 2011, 11, 5670 CrossRef CAS;
(b) F. Dai, S. Gong, P. Cui, G. Zhang, X. Qiu, F. Ye, D. Sun, Z. Pang, L. Zhang, G. Dong and C. Zhang, New J. Chem., 2010, 34, 2496 RSC;
(c) P. P. Cui, J. M. Dou, D. Sun, F. N. Dai, S. N. Wang, D. F. Sun and Q. Y. Wu, CrystEngComm, 2011, 13, 6968 RSC.
- X.-L. Zhao, D. Sun, T.-P. Hu, S. Yuan, L.-C. Gu, H.-J. Cong, H.-Y. He and D.-F. Sun, Dalton Trans., 2012, 41, 4320 RSC.
-
(a) B. Moulton and M. J. Zaworotko, Chem. Rev., 2001, 101, 1629 CrossRef CAS;
(b) B. Moulton and M. J. Zaworotko, Adv. Supramol. Chem., 2000, 7, 235 CrossRef CAS;
(c) L. Carlucci, G. Ciani, D. M. Proserpio and L. Spadacini, CrystEngComm, 2004, 6, 96 RSC;
(d) A. J. Blake, N. R. Brooks, N. R. Champness, M. Crew, A. Deveson, D. Fenske, D. H. Gregory, L. R. Hanton, P. Hubberstey and M. Schroder, Chem. Commun., 2001, 1432 RSC.
-
G. M. Sheldrick, SHELXS-97, Program for X-ray Crystal Structure Determination, University of Göttingen, Germany, 1997 Search PubMed.
-
G. M. Sheldrick, SHELXL-97, Program for X-ray Crystal Structure Refinement, University of Göttingen, Germany, 1997 Search PubMed.
-
A. L. Spek, Implemented as the PLATON Procedure, a Multipurpose Crystallographic Tool, Utrecht University, Utrecht, the Netherlands, 1998 Search PubMed.
-
K. Nakamoto, Infrared and Raman Spectra of Inorganic and Coordination Compounds, Part B, 5th ed., Wiley-Interscience, New York, 1997, pp. 87–89 Search PubMed.
-
(a) A. W. Addison, T. N. Rao, J. Reedijk, J. Van Rijn and G. C. Verschoor, J. Chem. Soc., Dalton Trans., 1984, 1349 RSC;
(b) L. Yang, D. R. Powell and R. P. Houser, Dalton Trans., 2007, 955 RSC.
-
(a) D. Sun, L. Zhang, Z. Yan and D. Sun, Chem.–Asian J., 2012, 7, 1558 CrossRef CAS;
(b) D. Sun, G.-G. Luo, N. Zhang, R.-B. Huang and L.-S. Zheng, Chem. Commun., 2011, 47, 1461 RSC;
(c) D. J. Hutchinson, S. A. Cameron, L. R. Hanton and S. C. Moratti, Inorg. Chem., 2012, 51, 5070 CrossRef CAS;
(d) V. Domasevitch, P. V. Solntsev, I. Y. A. Gural'skiy, H. Krautscheid, E. B. Rusanov, A. N. Chernega and J. A. K. Howard, Dalton Trans., 2007, 3893 RSC;
(e) I. Y. A. Gural'skiy, D. Escudero, A. Frontera, P. V. Solntsev, E. B. Rusanov, A. N. Chernega, H. Krautscheid and K. V. Domasevitch, Dalton Trans., 2009, 2856 RSC;
(f) Y. F. Hsu, H. L. Hu, C. J. Wu, C. W. Yeh, D. M. Proserpio and J. D. Chen, CrystEngComm, 2009, 11, 168 RSC;
(g) C. J. Wu, M. J. Sie, H. L. Hsiao and J. D. Chen, CrystEngComm, 2011, 13, 4121 RSC.
- Q. M. Wang and T. C. W. Mak, Chem.–Eur. J., 2003, 9, 44 Search PubMed.
- M. Mascal, J. L. Kerdelhue, A. J. Blake and P. A. Cooke, Angew. Chem., Int. Ed., 1999, 38, 1968 CrossRef CAS.
- S. L. Zheng, M. L. Tong, S. D. Tan, Y. Wang, J. X. Shi, Y. X. Tong, H. K. Lee and X. M. Chen, Organometallics, 2001, 20, 5319 CrossRef CAS.
- M. Munakata, L. P. Wu and G. L. Ning, Coord. Chem. Rev., 2000, 198, 171 CrossRef CAS.
-
(a) D. B. Cordes, A. S. Bailey, P. L. Caradoc-Davies, D. H. Gregory, L. R. Hanton, K. Lee and M. D. Spicer, Inorg. Chem., 2005, 44, 2544 CrossRef CAS;
(b) Y. F. Hsu, H. L. Hu, C. J. Wu, C. W. Yeh, D. M. Proserpio and J. D. Chen, CrystEngComm, 2009, 11, 168 RSC;
(c) J. J. Cheng, Y. T. Chang, C. J. Wu, Y. F. Hsu, C. H. Lin, D. M. Proserpio and J. D. Chen, CrystEngComm, 2012, 14, 537 RSC;
(d) Z. K. Chan, T. R. Chen, J. D. Chen, J. C. Wang and W. Liu, Dalton Trans., 2007, 3450 RSC.
-
A. F. Wells, Three-dimensional nets and polyhedra, Wiley-Interscience, New York, 1977 Search PubMed.
-
(a) S. L. Zheng and X. M. Chen, Aust. J. Chem., 2004, 57, 703 CrossRef CAS;
(b) C. L. Chen, B. S. Kang and C. Y. Su, Aust. J. Chem., 2006, 59, 3 CrossRef CAS;
(c) X. Zhao, L. Zhang, H. Ma, D. Sun, D. Wang, S. Feng and D. Sun, RSC Adv., 2012, 2, 5543 RSC;
(d) J. Cui, Y. F. Yue, G. D. Qian and B. L. Chen, Chem. Rev., 2012, 112, 1126 CrossRef.
-
(a) A. Barbieri, G. Accorsi and N. Armaroli, Chem. Commun., 2008, 2185 RSC;
(b) V. W.-W. Yam, K. K.-W. Lo, W. K.-M. Fung and C.-R. Wang, Coord. Chem. Rev., 1998, 171, 17 CrossRef CAS.
Footnote |
† Electronic Supplementary Information (ESI) available: Synthetic and crystallographic details, additional measurements, figures and tables. CCDC numbers 888434 and 888435. For ESI and crystallographic data in CIF or other electronic format see DOI: 10.1039/c2ra21533k |
|
This journal is © The Royal Society of Chemistry 2012 |
Click here to see how this site uses Cookies. View our privacy policy here.