DOI:
10.1039/C3GC41467A
(Paper)
Green Chem., 2014,
16, 221-225
Efficient synthesis of quinazoline-2,4(1H,3H)-diones from CO2 using ionic liquids as a dual solvent–catalyst at atmospheric pressure†
Received
23rd July 2013
, Accepted 13th September 2013
First published on 16th September 2013
Abstract
The highly efficient transformation of CO2 into value-added chemicals is an interesting topic in green chemistry. In this work, we studied the synthesis of quinazoline-2,4(1H,3H)-diones from CO2 and 2-aminobenzonitriles in a series of ionic liquids (ILs). It was found that 1-butyl-3-methylimidazolium acetate ([Bmim]Ac), a simple and easily prepared IL, could act as both solvent and catalyst, the reactions could be carried out very efficiently at atmospheric pressure of CO2, and a high yield of the products was obtained. Further study indicated that the IL was also very efficient for converting other 2-aminobenzonitriles into their corresponding quinazoline-2,4(1H,3H)-diones in high yields at atmospheric pressure. Moreover, the separation of the products from the IL was very easy, and the IL could be reused at least five times without considerable loss in catalytic activity.
Introduction
Nowadays, the utilization of CO2 as a non-toxic, inexpensive, abundant and renewable C1 resource has received extensive attention. However, the development of highly-efficient routes to transform CO2 into value-added chemicals is a long-standing task because of both thermodynamic and kinetic problems.1
Ionic liquids (ILs) have some very attractive properties, such as being non-volatile, non-flammable, and having high thermal stabilities and excellent solubility in both organic and inorganic substances. In addition, the functions of ILs can be designed for different processes by changing the structures of their cations or anions. ILs have great potential in applications such as chemical reactions, material synthesis, separation and fractionation, etc.2,3 Catalytic reactions have been widely studied using ILs as solvents and/or catalysts. Especially, basic ILs, as a class of important functional ILs, have been extensively used in the Knoevenagel condensation,4 the hydrogenation of CO2 to formic acid,5 the Michael addition,6 the acetylation of alcohols including glucose,7 the preparation of 5-aryl-2-oxazolidinones from aziridines and CO2,8 the synthesis of disubstituted urea from amines and CO2,9 and so on.
Quinazoline-2,4(1H,3H)-diones are an important class of pharmaceutical intermediates. They can be synthesized by reactions of anthranilic acid derivatives with ureas,10 CO with 2-nitrobenzamide,11 anthranilic acid with chlorosulfonyl isocyanate or potassium cyanate,12 anthranilamides with phosgene,13 methyl anthranilate with iso(thio)cyanates,14 and diethylformamide with aromatic aminonitriles.15 However, these synthetic routes have some obvious disadvantages such as requirements for special and/or toxic reagents like phosgene.
In recent years, the synthesis of quinazoline-2,4(1H,3H)-diones using CO2 and 2-aminobenzonitriles as raw materials has attracted considerable interest (Scheme 1), and various kinds of catalysts have been used to promote the reactions, such as 1,8-diazabicyclo[5.4.0]undec-7-ene (DBU),16,17 CsCO3,18 1-butyl-3-methylimidazolium hydroxide ([Bmim]OH),19 MgO–ZrO2,20 1,1,3,3-tetramethylguanidine,21N-methyltetrahydropyrimidine22 and so on. However, these reaction systems have drawbacks such as long reaction times, high pressures of CO2, the use of organic solvents, and difficulties in recycling the catalysts. Recently, Kimura and co-workers discovered that TBA2[WO4] (TBA = tetra-n-butylammonium) could catalyze the reactions efficiently at atmospheric pressure using N-methylpyrrolidone or dimethyl sulfoxide as the solvents.23,24 Our group reported that this kind of reaction could proceed smoothly in water without any catalyst at a higher pressure and temperature.25
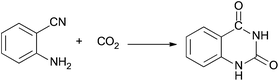 |
| Scheme 1 Reaction of CO2 and 2-aminobenzonitrile to quinazoline-2,4(1H,3H)-dione. | |
It is interesting to develop highly-efficient and green routes to prepare quinazoline-2,4(1H,3H)-diones from CO2 and 2-aminobenzonitriles under mild conditions. In this work, we studied the reaction in different ILs. It was found that 1-butyl-3-methylimidazolium acetate ([Bmim]Ac), a simple IL, could act as both solvent and catalyst for the reactions, and quinazoline-2,4(1H,3H)-diones could be synthesized very efficiently at atmospheric pressure. In addition, the separation of the products from the IL was very simple, and the IL could be reused at least five times without considerable loss in catalytic activity.
Results and discussion
Effect of different catalysts
It is known that bases are effective catalysts for this kind of reaction in different organic solvents.21 In this work, various catalysts, Cs2CO3, KF·2H2O, Et3N, and Zn(Ac)2 were tested using 2-aminobenzonitrile (1a) as the substrate in solvent-free conditions at 90 °C and 0.1 MPa with a reaction time of 10 h, and the results are given in Table 1. The results showed that none of these catalysts were effective for this reaction under the experimental conditions.
Table 1 Reaction of 2-aminobenzonitrile with CO2 catalyzed by various inorganic catalystsa
Entry |
Catalyst |
Yieldb (%) |
Reaction conditions: 2-aminobenzonitrile 5 mmol, catalyst 0.1 mmol, solvent-free, CO2 0.1 MPa, 10 h, 90 °C.
Isolated yield.
|
1 |
Without catalyst |
0 |
2 |
Cs2CO3 |
0 |
3 |
KF·2H2O |
0 |
4 |
Et3N |
0 |
5 |
Zn(Ac)2 |
0 |
The reaction was then carried out using various ILs as both catalysts and solvents, and the data are given in Table 2. Unfortunately, all of our initial attempts with the ILs 1-butyl-3-methylimidazolium dihydrophosphate ([Bmim]H2PO4), 1-butyl-3-methylimidazolium hydrosulfate ([Bmim]HSO4), 1-butyl-3-methylimidazolium chloride ([Bmim]Cl), 1-butyl-3-methylimidazolium tetrafluoroborate ([Bmim]BF4), 1-butyl-3-methylimidazolium nitrate ([Bmim]NO3), 1-butyl-3-methylimidazolium dibutyl phosphate ([Bmim](C4H9O)2PO2) and 1-butyl-3-methylimidazolium dicyanamide ([Bmim]N(CN)2) failed to produce the target product (entries 1–7). However, when we conducted the reaction with 1-butyl-3-methylimidazolium trifluoroacetate ([Bmim]CF3Ac), a 1% yield of the product was obtained (entry 8). Encouraged by this preliminary result and the knowledge of this class of reaction,23,24 a series of ILs were screened to optimize the solvent–catalyst systems using 2-aminobenzonitrile as the model substrate. The yields of 2a in tetramethylguanidine acetate ([TMG]Ac), 1,8-diazabicyclo[5.4.0]undec-7-ene acetate ([DBU]Ac), imidazole ILs 1-ethyl-3-methylimidazolium acetate ([Emim]Ac), 1-butyl-3-methylimidazolium acetate ([Bmim]Ac), and 1-octyl-3-methylimidazolium acetate ([Omim]Ac) were 23% (entry 9), 69% (entry 10), 88% (entry 11), 92% (entry 12), and 85% (entry 13), respectively.
Table 2 Reaction of 2-aminobenzonitrile with CO2 in various ILsa
Entry |
Solvent–catalyst |
Yieldb (%) |
Reaction conditions: 2-aminobenzonitrile 5 mmol, IL 1.0 g, CO2 0.1 MPa, 10 h, 90 °C.
Isolated yield.
|
1 |
[Bmim]H2PO4 |
0 |
2 |
[Bmim]HSO4 |
0 |
3 |
[Bmim]Cl |
0 |
4 |
[Bmim]BF4 |
0 |
5 |
[Bmim]NO3 |
0 |
6 |
[Bmim](C4H9O)2PO2 |
0 |
7 |
[Bmim]N(CN)2 |
0 |
8 |
[Bmim]CF3Ac |
1 |
9 |
[TMG ]Ac |
23 |
10 |
[DBU]Ac |
69 |
11 |
[Emim]Ac |
88 |
12 |
[Bmim]Ac |
92 |
13 |
[Omim]Ac |
85 |
The results above demonstrate that the activities of the ILs depend on both their cations and anions and the anion counterparts of the ILs appear to affect the reaction more significantly. All the ILs with an Ac− anion were active for the reaction. The main reason was that acetic acid is a weak acid and therefore the ILs are bases, and the reaction can be promoted by basic catalysis. The cations of the ILs also influenced reaction singificantly. For the ILs with the Ac− anion, the activities followed the order of [Bmim]Ac > [Emim]Ac > [Omim]Ac > [DBU]Ac > [TMG]Ac. The excellent activity of the imidazolium-based ILs may result from their optimal basicity for the reaction. Moreover, another reason for the reaction to proceed efficiently was that the ILs are a good solvent for both reactants.
The data in Table 1 illustrate that the reaction did not occur in the absence of catalyst. In order to prove the catalytic activity of the IL [Bmim]Ac for this reaction, control experiments employing catalytic amounts of the IL for the reaction were conducted using DMF (N,N-dimethylformamide) as the solvent, and the results are presented in Table 3. No product was formed when the reaction was carried out in the absence of the IL (entry 1), while the reaction occurred as the IL was added into the solvent, and the yield of product 2a increased with the increased amount of the IL, demonstrating that the IL was an active catalyst for the reaction. Therefore, it could be concluded that the IL acted as both solvent and catalyst for the reaction.
Table 3 The reaction of 2-aminobenzonitrile and CO2 in DMF with and without [Bmim]Aca
Entry |
[Bmim]Ac (mol%) |
Solvent |
Yieldb (%) |
Reaction conditions: 2-aminobenzonitrile 5 mmol, DMF 1.0 mL, CO2 0.1 MPa, 10 h, 90 °C.
Isolated yield.
|
1 |
0 |
DMF |
0 |
2 |
5 |
DMF |
14 |
3 |
30 |
DMF |
72 |
4 |
100 |
— |
92 |
Effect of temperature
The influence of temperature on the yield of 2a was investigated in [Bmim]Ac at 0.1 MPa in the temperature range of 60–120 °C with a reaction time of 10 h, and the results are shown in Fig. 1. It was shown that the yield of the product depended strongly on the reaction temperature in the lower temperature region. The yield reached 92% at 90 °C, and then stayed unchanged with further increasing of temperature, indicating that all the reactant could be converted at 90 °C.
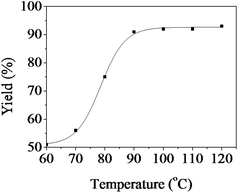 |
| Fig. 1 The dependence of the quinazoline-2,4(1H,3H)-dione isolated yield on temperature. Reaction conditions: 2-aminobenzonitrile 5 mmol, [Bmim]Ac 1.0 g, CO2 0.1 MPa, 10 h. Isolated yield. | |
Influence of reaction time
The dependence of the yield of 2a on the reaction time is presented in Fig. 2. The reaction was conducted at 90 °C and 0.1 MPa. As can be seen from Fig. 2, the isolated yield of 2a increased with increasing reaction time at the beginning, and a yield of 92% could be achieved in 10 h. No further increase in the yield was observed with prolonged reaction time, suggesting that all the reactant was transformed in 10 h.
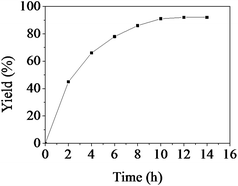 |
| Fig. 2 The dependence of the quinazoline-2,4(1H,3H)-dione isolated yield on reaction time. Reaction conditions: 2-aminobenzonitrile 5 mmol, [Bmim]Ac 1.0 g, CO2 0.1 MPa, 90 °C. Isolated yield. | |
Different substrates
To demonstrate the generality of this approach to the synthesis of quinazoline-2,4(1H,3H)-diones, we studied the reactions of various 2-aminobenzonitrile derivatives and CO2 at 90 °C and 0.1 MPa in [Bmim]Ac, and the results are summarized in Table 4. The reaction of 2-aminobenzonitrile 1a with CO2 gave a 92% yield of the quinazoline-2,4(1H,3H)-dione 2a under suitable reaction conditions (entry 1). The presence of an electron-donating group had a slight influence on the reaction and a yield of 95% of the 6,7-dimethoxyquinazoline-2,4(1H,3H)-dione 2b was obtained (entry 2). Furthermore, the reactivity of halogen substituted 2-aminobenzonitriles was also investigated (entries 3–5). It was observed that the reaction between 2-amino-5-chlorobenzonitrile 1c and CO2 proceeded smoothly providing a 91% yield of the 6-chloroquinazoline-2,4(1H,3H)-dione 2c (entry 3). Apart from 2-amino-5-chlorobenzonitrile, the reaction of 2-amino-5-bromobenzonitrile 1d was also found to occur smoothly with an 86% yield of 6-bromoquinazoline-2,4(1H,3H)-dione 2d (entry 4). However, the yield of the 7-chloroquinazoline-2,4(1H,3H)-dione 2e was lower (76%) for the reaction between 2-amino-4-chlorobenzonitrile 1e and CO2 due to the electron-withdrawing affect on the basicity.19,21
Table 4 Synthesis of various quinazoline-2,4(1H,3H)-diones 2a–ea
Entry |
Substrate |
Product |
Yieldb (%) |
Reaction conditions: reactant 5 mmol, [Bmim]Ac 1.0 g, CO2 0.1 MPa, 10 h, 90 °C.
Isolated yield.
Reaction time: 24 h.
|
1 |
|
|
92 |
2 |
|
|
95 |
3 |
|
|
91 |
4 |
|
|
86 |
5 |
|
|
76c |
Catalyst recyclability
The reusability of [Bmim]Ac was also examined for the synthesis of 2a from CO2 and 2-aminobenzonitrile under the optimized reaction conditions and the results are shown in Fig. 3. After the reaction, the IL was separated from the product and reactant by adding water into the stainless-steel batch reactor, and then the IL was used directly for the next run after drying. The detailed procedures are described in the Experimental section. The results in Fig. 3 illustrate that the IL could be reused at least five times without considerable loss of catalytic activity. 1H NMR and 13C NMR spectra show that the structure of the IL was not changed after reuse for five times.
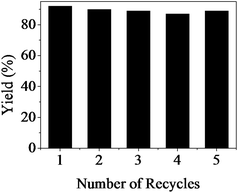 |
| Fig. 3 The recyclability of the IL [Bmim]Ac for the synthesis of quinazoline-2,4(1H,3H)-dione. Reaction conditions: 2-aminobenzonitrile 5 mmol, [Bmim]Ac 1.0 g, CO2 0.1 MPa, temperature 90 °C, 10 h. Isolated yield. | |
Reaction mechanism
On the basis of the knowledge of this class of reactions19,21 and the experimental results, a plausible mechanism for the [Bmim]Ac-catalyzed formation of quinazoline-2,4(1H,3H)-dione (2a) from the reaction of 2-aminobenzonitrile (1a) and CO2 is proposed as depicted in Scheme 2. Firstly, the acetate anion attacks the amino group, captures a proton, and activates the reactant 1a to produce an intermediate 3a. Then, the amide 3a reacts with CO2 rapidly, leading to the formation of a carbamate ester 4a. After that, 5a is formed via an intramolecular nucleophilic cyclization of 4a. Subsequently, the rearrangement of 5a, forming the isocyanate intermediate 6a, gives 7a. Finally, the final product 2a is obtained from the stabilization of 7a. In addition, the formation of the isocyanate intermediate 6a assisted by the o-cyano group appears to be important in the whole catalytic cycle.17
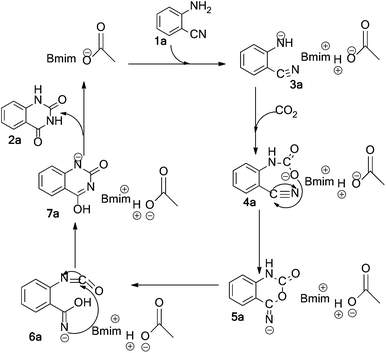 |
| Scheme 2 A plausible mechanism for the reaction of CO2 and 2-aminobenzonitrile catalyzed by [Bmim]Ac. | |
Conclusions
In summary, we found that quinazoline-2,4(1H,3H)-diones can be prepared under atmospheric pressure in high yields from the reaction of CO2 with 2-aminobenzonitriles in the presence of [Bmim]Ac which acts as a dual solvent–catalyst. After the reaction the IL can be easily recovered, and the [Bmim]Ac can be reused for five times without a considerable decrease in catalytic activity. This work provides an additional example that functional ILs have obvious advantages for conducting chemical reactions as catalysts and/or solvents. We believe that the highly-efficient, greener, inexpensive, active and stable catalytic system has potential application in the synthesis of quinazoline-2,4(1H,3H)-diones from CO2 and 2-aminobenzonitriles under mild conditions.
Experimental
Materials
CO2 was supplied by Beijing Analytical Instrument Factory with a purity of 99.99%. 2-Aminobenzonitrile (1a) (purity >98%) was purchased from J&K Scientific Ltd. 2-Amino-4,5-dimethoxybenzonitrile (1b) (purity >99%) was provided by Matrix Scientific. 2-Amino-5-chlorobenzonitrile (1c) (purity >99%) and 2-amino-5-bromobenzonitrile (1d) (purity >98%) were purchased from Fluorochem Ltd. 2-Amino-4-chlorobenzonitrile (1e) (purity >99%) was supplied by Sigma-Aldrich Co. Cs2CO3 and KF·2H2O were analytical grade and were purchased from Sinopharm Chemical Reagent Beijing Co., Ltd. Et3N and DMF (N,N-dimethylformamide) was analytical grade and provided by Beijing Chemical Reagents Company. The deuterated solvent (DMSO-d6) was obtained from Cambridge Isotope Laboratories, Inc. The ILs used were supplied by Lanzhou Institute of Chemical Physics; Chinese Academy of Sciences, which were dewatered before use.
Synthesis of quinazoline-2,4(1H,3H)-diones
As an example, the procedure using 2-aminobenzonitrile as the substrate is described, and those for other substrates were similar. In a typical experiment, 2-aminobenzonitrile (5 mmol, 0.591 g) and [Bmim]Ac (1.0 g) were loaded into a 22 mL stainless-steel batch reactor equipped with a magnetic stirrer. The air in the reactor was removed by blowing CO2 through the reactor. Then the CO2 pressure was kept at 0.1 MPa using a balloon with CO2. The reactor was placed in an oil bath at the desired temperature and the reaction mixture was stirred. After the reaction, the reactor was placed in ice water for 20 min. Then 25 mL water was added into the reactor. The mixture was centrifuged to precipitate the product and the aqueous solution of the IL was removed. The product was washed with water (3 × 25 mL) to remove the IL. Then the precipitate was washed with tert-butyl methyl ether 3 times (25 mL each time) to remove the reactant in the product. Finally, the mass of the product was determined by an electronic balance (Mettler-Toledo) with an accuracy of 0.1 mg after being dried at 95 °C for 3 hours. In the experiments to test the reusability of the IL, the IL was recovered by evaporating the water and drying at 70 °C for 24 h under vacuum. Then the IL was used for the next run.
The purified products were characterized by NMR and elemental analysis techniques. 1H NMR and 13C NMR studies were carried out with a Bruker AV400 (400 MHz, 100 MHz) NMR spectrometer with DMSO-d6 as the solvent. Elemental analysis for C, H and N were carried out with a Flash EA 1112 elemental analyzer. The characterization data of the products (2a–2e) are reported below.
Quinazoline-2,4(1H,3H)-dione (2a).
1H NMR (400 MHz, DMSO-d6) δ = 11.25 (s, 1H), 11.11 (s, 1H), 7.87 (dd, J = 8.3, 1.4 Hz, 1H), 7.63–7.59 (m, 1H), 7.16 (t, J = 7.4 Hz, 2H). 13C NMR (100 MHz, DMSO-d6) δ = 162.86, 150.34, 140.88, 134.87, 126.91, 122.22, 115.23, 114.36. C8H6N2O2: calcd C 59.26, H 3.73, N 17.28; found C 59.15, H 3.75, N 17.25.
6,7-Dimethoxyquinazoline-2,4(1H,3H)-dione (2b).
1H NMR (400 MHz, DMSO-d6) δ = 11.08 (s, 1H), 10.90 (s, 1H), 7.24 (s, 1H), 6.66 (s, 1H), 3.81 (s, 3H), 3.77 (s, 3H). 13C NMR (100 MHz, DMSO-d6) δ = 162.40, 154.89, 150.38, 145.01, 136.53, 107.14, 106.18, 97.75, 55.78, 55.69. C10H10N2O4: calcd C 54.05, H 4.54, N 12.61; found C 54.19, H 4.57, N 12.45.
6-Chloroquinazoline-2,4(1H,3H)-dione (2c).
1H NMR (400 MHz, DMSO-d6) δ = 11.35 (s, 2H), 7.79 (s, 1H), 7.66 (s, 1H), 7.17 (d, J = 8.8 Hz, 1H). 13C NMR (100 MHz, DMSO-d6) δ = 161.88, 150.10, 139.80, 134.78, 126.28, 125.90, 117.56, 115.79. C8H5N2O2Cl: calcd C 48.88, H 2.56, N 14.25; found C 48.92, H 2.65, N 14.13.
6-Bromoquinazoline-2,4(1H,3H)-dione (2d).
1H NMR (400 MHz, DMSO-d6) δ = 11.32 (s, 2H), 7.92 (s, 1H), 7.78 (d, J = 8.5 Hz, 1H), 7.10 (d, J = 8.6 Hz, 1H). 13C NMR (100 MHz, DMSO-d6) δ = 161.74, 150.02, 140.05, 137.47, 128.89, 117.75, 116.19, 113.81. C8H5N2O2Br: calcd C 39.86, H 2.09, N 11.62; found C 39.95, H 2.04, N 11.66.
7-Chloroquinazoline-2,4(1H,3H)-dione (2e).
1H NMR (400 MHz, DMSO-d6) δ = 11.30 (s, 2H), 7.86 (d, J = 7.4 Hz, 1H), 7.19 (d, J = 8.0 Hz, 1H), 7.15 (s, 1H). 13C NMR (100 MHz, DMSO-d6) δ = 162.09, 150.19, 141.97, 139.29, 129.01, 122.46, 114.68, 113.32. C8H5N2O2Cl: calcd C 48.88, H 2.56, N 14.25; found C 48.95, H 2.62, N 14.16.
Acknowledgements
The authors thank the Ministry of Science and Technology of China (2011CB808603), the National Natural Science Foundation of China (21173239, 21133009, 21021003), and the Chinese Academy of Sciences (KJCX2.YW.H30).
Notes and references
-
M. Aresta, Carbon Dioxide as Chemical Feedstock, Wiley-VCH, Weinheim, 2010 Search PubMed.
- V. I. Pârvulescu and C. Hardacre, Chem. Rev., 2007, 107, 2615 CrossRef PubMed.
- J. P. Hallett and T. Welton, Chem. Rev., 2011, 111, 3508 CAS.
- B. C. Ranu and R. Jana, Eur. J. Org. Chem., 2006, 3767 CrossRef CAS.
- Z. F. Zhang, Y. Xie, W. J. Li, S. Q. Hu, J. L. Song, T. Jiang and B. X. Han, Angew. Chem., Int. Ed., 2008, 47, 1127 CrossRef CAS PubMed.
- B. C. Ranu, S. Banerjee and R. Jana, Tetrahedron, 2007, 63, 776 CrossRef CAS PubMed.
- S. A. Forsyth, D. R. MacFarlane, R. J. Thomson and M. von Itzstein, Chem. Commun., 2002, 714 RSC.
- Z. Z. Yang, L. N. He, S. Y. Peng and A. H. Liu, Green Chem., 2010, 12, 1850 RSC.
- T. Jiang, X. Ma, Y. Zhou, S. Liang, J. Zhang and B. Han, Green Chem., 2008, 10, 465 RSC.
- M. C. Willis, R. H. Snell, A. J. Fletcher and R. L. Woodward, Org. Lett., 2006, 8, 5089 CrossRef CAS PubMed.
- M. Akazome, J. Yamamoto, T. Kondo and Y. Watanabe, J. Organomet. Chem., 1995, 494, 229 CrossRef CAS.
- H. Vorbruggen and K. Krolikiewicz, Tetrahedron, 1994, 50, 6549 CrossRef CAS.
- D. Q. Shi, G. L. Dou, Z. Y. Li, S. N. Ni, X. Y. Li, X. S. Wang, H. Wu and S. J. Ji, Tetrahedron, 2007, 63, 9764 CrossRef CAS PubMed.
- Z. G. Li, H. Huang, H. B. Sun, H. L. Jiang and H. Liu, J. Comb. Chem., 2008, 10, 484 CrossRef CAS PubMed.
- L. Jiarong, C. Xian, S. Daxin, M. Shuling, L. Qing, Z. Qi and T. Jianhong, Org. Lett., 2009, 11, 1193 CrossRef PubMed.
- T. Mizuno, T. Iwai and Y. Ishino, Tetrahedron Lett., 2004, 45, 7073 CrossRef CAS PubMed.
- T. Mizuno, M. Mihara, T. Nakai, T. Iwai and T. Ito, Synthesis, 2007, 2524 CrossRef CAS PubMed.
- Y. P. Patil, P. J. Tambade, S. R. Jagtap and B. M. Bhanage, Green Chem. Lett. Rev., 2008, 1, 127 CrossRef CAS.
- Y. P. Patil, P. J. Tambade, K. M. Deshmukh and B. M. Bhanage, Catal. Today, 2009, 148, 355 CrossRef CAS PubMed.
- Y. P. Patil, P. J. Tambade, K. D. Parghi, R. V. Jayaram and B. M. Bhanage, Catal. Lett., 2009, 133, 201 CrossRef CAS PubMed.
- J. Gao, L. N. He, C. X. Miao and S. Chanfreau, Tetrahedron, 2010, 66, 4063 CrossRef CAS PubMed.
- D. Nagai and T. Endo, J. Polym. Sci., Part A: Polym. Chem., 2009, 47, 653 CrossRef CAS.
- T. Kimura, K. Kamata and N. Mizuno, Angew. Chem., Int. Ed., 2012, 51, 6700 CrossRef CAS PubMed.
- T. Kimura, H. Sunaba, K. Kamata and N. Mizuno, Inorg. Chem., 2012, 51, 13001 CrossRef CAS PubMed.
- J. Ma, B. X. Han, J. L. Song, J. Y. Hu, W. J. Lu, D. Z. Yang, Z. F. Zhang, T. Jiang and M. Q. Hou, Green Chem., 2013, 15, 1485 RSC.
Footnote |
† Electronic supplementary information (ESI) available: 1H NMR and 13C NMR spectra of the products in Table 4 and IL [Bmim]Ac. See DOI: 10.1039/c3gc41467a |
|
This journal is © The Royal Society of Chemistry 2014 |
Click here to see how this site uses Cookies. View our privacy policy here.