DOI:
10.1039/C3QI00037K
(Communication)
Inorg. Chem. Front., 2014,
1, 49-52
Quantification of ion binding using electrospray mass spectrometry†
Received
10th September 2013
, Accepted 23rd October 2013
First published on 6th January 2014
Abstract
Mass spectrometry has predominately been used as a qualitative tool in the analysis of complexes with quantitative studies being difficult to validate. Here, we present a method where electrospray mass spectrometry is used to give both a qualitative and quantitative measure of ion binding with cross validation by solution 1H NMR, demonstrating it is possible to utilize gas-phase electrospray mass spectrometry continuous variation data to deduce binding stoichiometries in solutions where artifacts and nonspecific complexes are also observed.
The development of artificial synthetic receptors has been expanding greatly over the past decade1–4 as supramolecular principles are applied across an ever increasing range of molecules, materials, and systems.5–7 Over the past two decades analysis of such supramolecular complexes has become increasing straightforward with the utilization of soft ionization techniques, such as fast atom bombardment (FAB), matrix-assisted laser desorption (MALDI) and electrospray ionisation (ESI-MS)8–14 and studies using NMR methods such as the continuous method of variation (Job plot) and NMR titration experiments.15–20 In 2002, Miyake et al. used fast atom bombardment mass spectrometry (FAB-MS) to provide evidence of inclusion complex formation between a cyclophane and an aromatic guest by constructing a Job plot from the absolute intensity of the observed complexation.21,22 Also, Van Dorsselaer in 1996 conducted dilution based ESI studies to provide insight into thermodynamic equilibrium in solution.23 Surprisingly though, no method utilizing electrospray mass spectrometry (ESI-MS) has been developed to form Job plots. Given that ESI-MS is a soft ionisation technique, we hypothesized that it may be possible to probe the formation of certain coordination complexes with little perturbation. The nature of mass spectrometry is such that peaks from artifacts and nonspecific complexes may be observed along with the thermodynamically preferred species and so a clear indication of the preferred stoichiometry of the complex is unattainable from peak observation alone. With this method the true stoichiometry can be found. To explore this, we set out to develop an experiment protocol that utilizes the method of continuous variation to evaluate the binding stoichiometries of three receptor–ion pairings and compare both NMR and ESI-MS methods to illustrate that ESI-MS can be used as a tool in the quantitative and qualitative analysis of certain labile coordination complexes. This method also allowed the study of the stoichiometry of paramagnetic complexes which, due to the broadening and overlapping of peaks, has proven difficult using conventional NMR techniques. It has also allowed low concentration studies removing some of the issues that could arise when carrying out quantitative studies at higher concentrations. The soft nature of the ionization may also allow the observation of weak interactions that may not be seen with more harsh conditions.
The first receptor that was examined was the receptor cis,cis-1,3,5-tris-acetylamino-cyclohexane 1 which was synthesised from a ligand that has been extensively studied, cis,cis-1,3,5-triaminocyclohexane (cis-tach),24–26 and acetic anhydride (see Scheme 1). As the aim of the research was to evaluate the use of ESI-MS as a quantitative analytical tool, the ligand was designed to be simple and also to be able to bind spherical anions such as chloride, bromide and iodide.
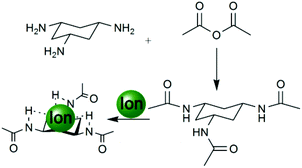 |
| Scheme 1 A simple tripodal cis-tach anion receptor 1 synthesised from cis-tach and acetic anhydride refluxed with triethylamine in methanol for 24 hours. | |
In order to obtain the stoichiometries of the 1–anion complexes, an ESI-MS method was developed analogous to the method of continuous variation (or Job plots)27 used with NMR where standard solutions (0.02 M) of 1 and the salt (KCl/KBr/KI) were made up in methanol and varying ratios of each solution were taken and mixed together (with the overall concentration remaining the same) e.g. 1 mL of 1 solution mixed with 9 mL of the salt solution, 2 mL of 1 solution mixed with 2 mL of the salt solution, 3 mL of 1 solution mixed with 7 mL of the salt solution up to 9 mL of 1 solution mixed with 1 mL of the salt solution. ESI-MS at 30 °C of the reaction mixtures was then carried out (where the acquisition parameters were kept the same for each experiment) and the intensity of the corresponding peak recorded. The recorded change in intensity multiplied by the mole fraction (where mole fraction is [Host]/[Host] + [Guest]) was then plotted against mole fraction to give a maximum value at 0.5 mole fraction and therefore a stoichiometry of 1
:
1 (1
:
A) for each guest e.g. the Job plot of 1 with KCl in methanol was achieved by plotting the recorded change in intensity of the [C6H12N3(C2H3O)3Cl]− (290.1 m/z) peak (see Fig. 2) multiplied by mole fraction ([Host]/[Host] + [Guest])27 against mole fraction to give a maximum at 0.5, indicative of a 1
:
1 stoichiometry (see Fig. 1, part A). It should be noted here that a small abundance of higher order stoichiometries were also present in the spectrum but applying the same method to these species did not give a continued variation and so we can postulate that this method will only work on the species with the correct thermodynamically preferred stoichiometry. This method was also applied to 1 in the presence of KBr (see Fig. 1 parts C and D) and KI to produce ESI-MS Job plots both with maxima at 0.5 (see ESI† for experimental details and MS parameters).
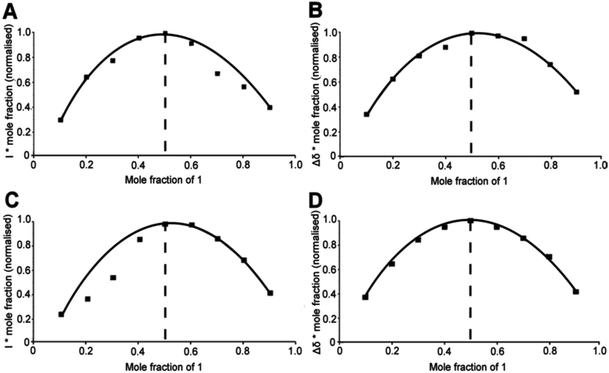 |
| Fig. 1 (A) MS (CH3OH) Job plot of 1 + Cl− showing a 1 : 1 (1 : Cl−) stoichiometry; (B) 1H NMR (d6-DMSO) Job plot of 1 + Cl− showing a 1 : 1 (1 : Cl−) stoichiometry; (C) MS (CH3OH) Job plot of 1 + Br− showing a 1 : 1 (1 : Br−) stoichiometry; (D) 1H NMR (d6-DMSO) Job plot of 1 + Br− showing a 1 : 1 (1 : Br−) stoichiometry. | |
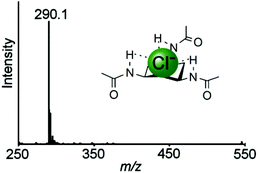 |
| Fig. 2 Mass spectrum at 30 °C of the receptor 1 in the presence of KCl giving the species [C6H12N3(C2H3O)3Cl]− (290.1 m/z). | |
By using conventional 1H NMR methods, the complexation of the receptor 1 to the three different anions Cl−, Br− and I− was also studied and compared. The experimental set up was as before, with the mass spectrometry experiment where standard solutions (0.02 M) of 1 and the salt (KCl/KBr/KI) were made up in d6-DMSO. Varying ratios of each solution were then taken and mixed together (with the overall concentration remaining the same). 1H NMR experiments were carried out for each of the mixtures and the receptor where a plot of Δδ (where Δδ = the shift of the receptor − (shift of the receptor + guest)) multiplied by the mole fraction was then plotted against mole fraction. It was observed that the NH chemical shifts of the receptor shifted upfield in the presence of the anionic guest, indicating that complexation had occurred (see Fig. 3). A Job plot was then produced for each anion with the maximum at 0.5 for each, corresponding to a stoichiometry of 1
:
1 (see Fig. 1 parts B and D, Fig. 3 and the ESI†).
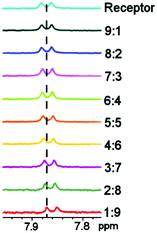 |
| Fig. 3
1H NMR (d6-DMSO) showing the shift of the amide NH when hydrogen bonding to chloride occurs; ratio is receptor to anion. | |
Job plot studies of 12-crown-4 with Na+ and Li+ were then carried out using ESI-MS and 1H NMR to demonstrate the observation of more complex stoichiometries. Both ESI-MS and 1H NMR job plots showed a 1
:
1 (12-crown-4
:
cation) stoichiometry when Li+ was coordinated to the crown ether and a stoichiometry of 2
:
1 (12-crown-4
:
Na) was found with both methods when Na+ was coordinated (see ESI† for the Job plots of 12-crown-4 with Na+ and Li+).
The method developed for the mass spectrometry experiments was then applied to a complex whose stoichiometry could not be obtained using 1H NMR methods due to its paramagnetic behavior. A Job plot of the complex [Co(C10H8N2)2(NO3)]−
282 (433.1 m/z) (see Fig. 4), which was formed when 2,2′-bipyridine was reacted in the presence of Co(NO3)2·6H2O in methanol, was produced where the maximum of the normalized intensity was at 0.67 mole fraction therefore showing a 2
:
1 receptor to metal stoichiometry (see Fig. 4).
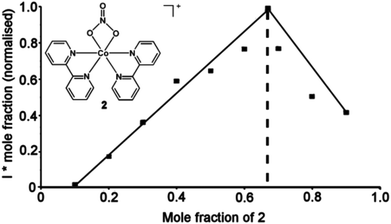 |
| Fig. 4 Reaction scheme and Job plot of [Co(C10H8N2)2(NO3)]−2 in the presence of Co(NO3)2·6H2O in methanol at 30 °C showing a 2 : 1 receptor : metal stoichiometry. | |
Conclusions
In conclusion, this research has shown that mass spectrometry, in the case of the above coordination complexes, can be used as both a qualitative and quantitative analytical tool. It can be used to demonstrate binding stoichiometries not only through observation of a species, but also through a continuous variation method. Often, with the conventional 1H NMR method, confirmation of complex formation can be problematic with broadening and overlapping of peaks, and so the use of ESI-MS to analyse the data removes some of the ambiguity that comes with using NMR methods.
Notes and references
- N. Busschaert, I. L. Kirby, S. Young, S. J. Coles, P. N. Horton, M. E. Light and P. A. Gale, Angew. Chem., Int. Ed., 2012, 51, 4426 CrossRef CAS PubMed.
-
(a) C. Caltagirone, J. R. Hiscock, M. B. Hursthouse, M. E. Light and P. A. Gale, Chem.–Eur. J., 2008, 14, 10236 CrossRef CAS PubMed;
(b) C. Lincheneau, R. M. Duke and T. Gunnlaugsson, Org. Biomol. Chem., 2012, 10, 6069 RSC.
- S. Comby, S. A. Tuck, L. K. Truman, O. Kotova and T. Gunnlaugsson, Inorg. Chem., 2012, 51, 10158 CrossRef CAS PubMed.
-
(a) P. V. Mason, N. R. Champness, S. R. Collinson, M. G. Fisher and G. Goretzki, Eur. J. Org. Chem., 2006, 1444 CrossRef CAS;
(b) S. K. Kim, D. E. Gross, D.-G. Cho, V. M. Lynch and J. L. Sessler, J. Org. Chem., 2011, 76, 1005 CrossRef CAS PubMed;
(c) S. K. Kim, B. P. Hay, J. S. Kim, B. A. Moyer and J. L. Sessler, Chem. Commun., 2013, 49, 2122 Search PubMed;
(d) S. O. Kang, R. A. Begum and K. Bowman-James, Angew. Chem., Int. Ed., 2006, 45, 7882 CrossRef CAS PubMed.
-
(a) A. C. Sather, O. B. Berryman and J. Rebek Jr., J. Am. Chem. Soc., 2010, 132, 13572 CrossRef CAS PubMed;
(b) P. M. P. Anderson, R. J. Gregory, S. Thompson, D. W. Souza, S. Paul, R. C. Mulligan, A. E. Smith and M. J. Welsh, Science, 1991, 253, 202 Search PubMed.
-
(a) Y. Yang, B. Szamosfalvi, J. Yee, S. Frinak and E. V. Anslyn, Analyst, 2011, 136, 317 RSC;
(b) P. A. Gale, Coord. Chem. Rev., 2003, 240, 191 CrossRef CAS;
(c) P. D. Beer and P. A. Gale, Angew. Chem., Int. Ed., 2001, 40, 486 CrossRef CAS.
-
(a) S. K. Kim, G. I. Vargas-Zúñiga, B. P. Hay, N. J. Young, L. H. Delmau, C. Masselin, C.-H. Lee, J. S. Kim, V. M. Lynch, B. A. Moyer and J. L. Seesler, J. Am. Chem. Soc., 2012, 134, 1782 CrossRef CAS PubMed;
(b) J. J. Gassensmith, H. Furukawa, R. A. Smaldone, R. S. Forgan, Y. Y. Botros, O. M. Yaghi and J. F. Stoddart, J. Am. Chem. Soc., 2011, 133, 15312 CrossRef CAS PubMed;
(c) E. Busseron and J. Rebek Jr., Org. Lett., 2010, 12, 4828 CrossRef CAS PubMed.
- C. A. Schalley, Int. J. Mass Spectrom., 2000, 194, 11 CrossRef CAS.
-
(a) H. Zhang, M. Grabenauer, M. T. Bowers and D. V. Dearden, J. Phys. Chem. A, 2009, 113, 1508 CrossRef CAS PubMed;
(b) D. V. Dearden, T. A. Ferrell, M. C. Asplund, L. W. Zilch, R. R. Julian and M. F. Jarrold, J. Phys. Chem. A, 2009, 113, 989 CrossRef CAS PubMed;
(c) C. A. Schalley, J. Hoernschemeyer, X. Li, G. Silva and P. Weis, Int. J. Mass Spectrom., 2003, 228, 373 CrossRef CAS;
(d) C. A. Schalley, T. Martín, U. Obst and J. Rebek Jr., J. Am. Chem. Soc., 1999, 121, 2133 CrossRef CAS;
(e) T. Becherer, D. Meshcheryakov, A. Springer, V. Böhmer and C. A. Schalley, J. Mass Spectrom., 2009, 44, 1338 CrossRef CAS PubMed;
(f) T. Shiga, G. N. Newton, J. S. Mathieson, T. Tetsuka, M. Nihei, L. Cronin and H. Oshio, Dalton Trans., 2010, 39, 4730 RSC.
-
(a) S. E. Pierce, R. Kieltyka, H. F. Sleiman and J. S. Brodbelt, Biopolymers, 2008, 91, 233 CrossRef PubMed;
(b) J. B. Shaw, J. A. Madsen, H. Xu and J. S. Brodbelt, J. Am. Soc. Mass Spectrom., 2012, 23, 1707 CrossRef CAS PubMed;
(c) S. I. Smith, F. S. Guziec Jr., L. Guziec and J. S. Brodbelt, Analyst, 2009, 134, 2058 RSC;
(d) J. S. Brodbelt, Annu. Rev. Anal. Chem., 2010, 3, 67 CrossRef CAS PubMed;
(e) C. L. Mazzitelli, Y. Chu, J. J. Reczek, B. L. Iverson and J. S. Brodbelt, J. Am. Soc. Mass Spectrom., 2007, 18, 311 CrossRef CAS PubMed.
- D.-L. Long, C. Streb, Y.-F. Song, S. Mitchell and L. Cronin, J. Am. Chem. Soc., 2008, 130, 1830 CrossRef CAS PubMed.
-
(a) S. Fukuzumi, K. Ohkubo, D. S. Kim, J. S. Park, A. Jana, V. M. Lynch, D. Kim and J. L. Sessler, J. Am. Chem. Soc., 2011, 133, 15938 CrossRef CAS PubMed;
(b) J. S. Park, E. Karnas, K. Ohkubo, P. Chen, K. M. Kadish, S. Fukuzumi, C. W. Bielawski, T. W. Hudnall, V. M. Lynch and J. L. Sessler, Science, 2010, 329, 1324 CrossRef CAS PubMed;
(c) K. Tiefenbacher and J. Rebek Jr., J. Am. Chem. Soc., 2012, 134, 2914 CrossRef CAS PubMed;
(d) F. Cui, S. Li, C. Jia, J. S. Mathieson, L. Cronin, X.-J. Yang and B. Wu, Inorg. Chem., 2012, 51, 179 CrossRef CAS PubMed;
(e) A. Coskun, M. Hmadeh, G. Barin, F. Gándara, E. Choi, N. L. Strutt, D. B. Cordes, A. M. Z. Slawin, J. F. Stoddart, P. Sauvage and O. M. Yaghi, Angew. Chem., Int. Ed., 2012, 51, 2160 CrossRef CAS PubMed;
(f) J.-F. Ayme, J. E. Beves, D. A. Leigh, R. T. McBurney, K. Rissanen and D. Schultz, Nat. Chem., 2012, 4, 15 CrossRef CAS PubMed;
(g) S. K. Kim, J. L. Sessler, D. E. Gross, C.-H. Lee, J. S. Kim, V. M. Lynch, L. H. Delmau and B. P. Hay, J. Am. Chem. Soc., 2010, 132, 5827 CrossRef CAS PubMed.
- L. Vilà-Nadal, A. Rodríguez-Fortea, L.-K. Yan, E. F. Wilson, L. Cronin and J. M. Poblet, Angew. Chem., Int. Ed., 2009, 48, 144 CrossRef PubMed.
- A. V. Simionato, M. D. Cantú and E. Carrilho, Microchem. J., 2006, 82, 214 CrossRef CAS PubMed.
- D. K. Smith, Org. Biomol. Chem., 2003, 1, 3874 CAS.
- P. D. Beer, C. Hazelwood, D. Hesek, J. Hodacova and S. E. Stokes, Dalton Trans., 1993, 1327 RSC.
- P. A. Gale and T. Gunnlaugsson, Chem. Soc. Rev., 2010, 39, 3595 RSC.
- C. Markert and A. Pfaltz, Angew. Chem., Int. Ed., 2004, 43, 2498 CrossRef CAS PubMed.
- D. Feichtinger and D. A. Plattner, Angew. Chem., Int. Ed., 1997, 36, 1718 CrossRef CAS.
- M. T. Blanda, J. H. Horner and M. Newcom, J. Org. Chem., 1989, 54, 4626 CrossRef CAS.
- M. Koichi, Y. Kimura and M. Miyake, J. Mass Specrtom. Soc. Jpn., 2002, 50, 301 CrossRef.
- M. Koichi, Y. Kimura and M. Miyake, J. Mass Specrtom. Soc. Jpn., 2003, 51, 566 CrossRef.
- E. Leize, A. Jaffrezic and A. Van Dorsselaer, J. Mass Spectrom., 1996, 31, 537 CrossRef CAS.
- A. L. Pickering, G. Seeber, D.-L. Long and L. Cronin, Chem. Commun., 2004, 136 RSC.
- G. N. Newton, G. J. T. Cooper, D. Schuch, T. Shiga, S. Khanra, D.-L. Long, H. Oshio and L. Cronin, Dalton Trans., 2009, 1549 RSC.
- A. L. Pickering, G. J. T. Cooper, D.-L. Long and L. Cronin, Polyhedron, 2004, 23, 2075 CrossRef CAS PubMed.
- P. Job, Ann. Chim. Appl., 1928, 113 CAS.
- R. Ivaniková, I. Svoboda, A. Mašlejová, B. Papánková and H. Fuess, Acta Crystallogr., Sect. E: Struct. Rep. Online, 2006, 62, 3024 Search PubMed.
Footnote |
† Electronic supplementary information (ESI) available: Tables of 1H NMR and mass spectral data. See DOI: 10.1039/c3qi00037k |
|
This journal is © the Partner Organisations 2014 |
Click here to see how this site uses Cookies. View our privacy policy here.