DOI:
10.1039/C3QO00059A
(Tutorial Account)
Org. Chem. Front., 2014,
1, 298-302
Chiral N,N′-dioxide ligands: synthesis, coordination chemistry and asymmetric catalysis†
Received
29th November 2013
, Accepted 5th January 2014
First published on 4th February 2014
Abstract
A class of conformationally flexible ligands composed of a tertiary amino oxide–amide backbone and a straight-chain alkyl spacer was developed. These C2-symmetric chiral N,N′-dioxide ligands could be straightforwardly synthesized from readily available amino acids and amines. They act as neutral tetradentate ligands to bind a wide variety of metal ions. Non-planar cis-α M(N,N′-dioxide) complexes enable an intriguing and easily fine-tuned chiral platform for a number of asymmetric reactions. Privileged N,N′-dioxide ligands frequently show wide substrate generality and exceptional levels of stereocontrol for a specific catalytic reaction. We describe approaches to the ligand design and synthesis, structure and bonding in coordination complexes, and the recent developments in asymmetric catalysis.
Introduction
Asymmetric catalysis has received considerable attention in the past few years, and it ranks among one of the most important objectives in organic synthesis. Enormous numbers of metal- and organo-catalysts have been developed in producing enantioenriched molecules with maximum efficiency.1–3 In particular, the inherent properties of chiral ligand–metal cooperative catalysis make it an attractive platform from which to develop catalytic processes of high reactivity and selectivity.4 The main families of successful ligands are greatly aided by several guiding principles. It is widely recognized that conformationally rigid scaffolds and multidentate properties of the ligand play critical roles in allowing it “privilege” in the formation of metal-based catalysts.3,5,6 Another frequently used principle in the design of synthetic catalysts is symmetry, because the presence of rotational symmetry in chiral ligands could limit the number of possible isomeric catalyst–substrate complexes.7,8 For example, a variety of privileged chiral ligands display C2-symmetry and rigid backbones such as bis(phosphine) compounds3,9 (e.g., BINAP, Duphos), spirobiindane based ligands,3 and bis(oxazolines).3,10 Additionally, with regard to the synthetic criteria, the ligand preparation should be accessible in a modular way and in only a few steps with high yield and optical purity from readily available starting materials. During recent years, our group has explored a new type of C2-symmetric ligands, namely, chiral N,N′-dioxides synthesized from cheap optically pure amino acids.11,12 These particular ligands have two alkyl amine oxide–amide subunits separated by a straight-chain alkyl spacer. They are full of conformationally flexible subunits13 that challenge the notion of the rigid feature of successful ligands (Fig. 1a). Interestingly, N,N′-dioxides provide an intriguing arsenal of chiral metal complex catalysts for asymmetric catalysis upon coordination with a variety of metal precursors (Fig. 1). In this paper, we summarize our efforts in the design and synthesis of chiral N,N′-dioxide ligands, and the study of their coordination chemistry, as well as their updated applications in asymmetric catalytic transformations.11
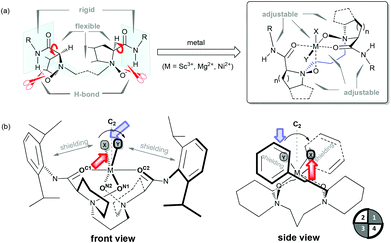 |
| Fig. 1 (a) General structures of chiral N,N′-dioxide/metal catalysts. (b) Molecular models of L–metal complex (the isopropyl substituents on the amides are omitted in the side view for clarity). | |
Design and syntheses of N,N′-dioxide ligands
N-Oxides are highly polar molecules. The reactions of tertiary amines or pyridine with peracids such as 3-chloroperoxybenzoic acid (mCPBA) and hydrogen peroxide easily generate the corresponding N-oxides. The basicity and ready availability of N-oxides greatly contributes to the abundance of their coordination chemistry.14 Nevertheless, the application of chiral N-oxide-based metal complexes in asymmetric catalysis remained limited to a few successful attempts15,16 until we initiated our project. Pyridine N-oxides as chiral controllers meet a difficulty of versatility, whereas tertiary amine oxides might suffer from the installation of a new stereocenter at nitrogen. Therefore, to rationally design chiral tertiary amine oxide ligands and catalysts is interesting and challenging.
In 1993, a complete diastereoselective synthesis of homochiral N-oxides from oxidation of N-alkylated prolinamide derivatives was discovered by O'Neil's group.17,18 The modular structure offers an opportunity for the design of a new chiral N-oxide library. After an initial success in the application of a biquinoline N,N′-dioxide19 and a mono N-oxide from L-prolinol,20 we were intrigued to design C2-symmetric N,N′-dioxides in view of the beneficial influence of symmetry and multidentate ligands in enantioselective catalysis. C2-Symmetric N,N′-dioxides21 are readily prepared through straightforward and cost-effective procedures as shown in Scheme 1. Chiral amino acids containing a secondary amino group, such as L-proline, L-pipecolic acid, L-ramipril, and (S)-tetrahydroisoquinoline-3-carboxylic acid, could easily be transformed into a number of chiral N,N′-dioxides. Take the synthesis of N,N′-dioxide L2 for example.22 Amino amide 3 could easily be obtained from (2S,3aS,6aS)-N-Boc-amino acid 1b. The corresponding C2-symmetric bisamide 4 was generated by coupling of the two amine units via 1,3-dibromopropane/K2CO3 in acetonitrile. It was then treated with mCPBA (2.5 equivalents) to give the N,N′-dioxide as a single enantiomer without resolution. Further details are given in the ESI.†
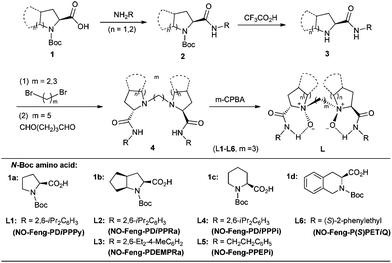 |
| Scheme 1 General synthesis route of C2-symmetric N,N′-dioxides and names of selected ligands. | |
Such a structurally diversified ligand library then leads to the discovery of novel classes of catalysts with very promising generality. In the early stages of research, N,N′-dioxides were used as chiral organocatalysts for Brønsted base and H-bond-assisted reactions.21 Nowadays, the utility of this kind of compounds is greatly expanded by using them as privileged ligands for Lewis acid-catalysed asymmetric reactions.11 In light of the potential application of chiral N,N′-dioxides, we decide to establish the market using NO-Feng-XXX as a brand name for the whole ligand family (Scheme 1). Both enantiomers of the ligands will be commercially available from J&K Scientific Ltd soon.
Structures of N,N′-dioxide–metal complexes
Generating organometallic complexes in situ can usually circumvent multiple purifications. When a chiral N,N′-dioxide is mixed with an inorganic salt while in an organic solvent at room temperature, a chiral metallic complex system is formed, which is used directly for asymmetric catalysis. Key coordination information can be obtained from the X-ray analysis of crystalline compounds of the N,N′-dioxide ligand L711 and the metal complexes22–25 (e.g., M = Sc3+, Mg2+, and Ni2+). In the presence of an appropriate metal ion, the intramolecular hydrogen-bonds of the N,N′-dioxide disappear, and thereafter, the amide and amine oxide moieties could switch laterally and inwardly to coordinate to the metal center due to the configurational freedom of the sp3 α-carbons13 and alkyl spacer (Fig. 1a). N,N′-Dioxide acts as a neutral tetradentate ligand that binds a metal ion securely through two amine oxide oxygens and two amide oxygens. The two amides are oriented in an approximately linear fashion pointing in opposite directions. Two coordination sites can be occupied by substrates, solvent molecules or counter anions. Notably, the conformation of the six-membered chelate ring determines an octahedral cis-α-N,N′-dioxide–metal complex. It differs from the major trans- and cis-β configuration of the privileged tetradentate salen–metal complexes.3,26 The cis-α-metal complexes of N,N′-dioxides are chiral and expected to be efficient asymmetric catalysts, since there are two coordination sites (X or Y) in a cis-position which can capture an ancillary bidentate ligand23 or two monodentate ligands.24,25
Fig. 1b illustrates the chiral structure created by an N,N′-dioxide L4–metal complex.24 As shown in the front view, the two amide subunits lie out of the horizontal OC1–M–OC2 plane (near a line), front right and rear left, respectively. The two piperidine rings and the alkyl linkage are located below the OC1–M–OC2 plane, whereas the above coordination sites ■ hold the positions diagonal to the amine oxide oxygens. Consequently, the two kinds of quadrant of the chiral structure (first and third versus second and fourth in the side view) are clearly discriminated spatially, where the first and third ones are sterically crowded by the amides, while the second and fourth ones are partly sterically influenced by the amine oxide moieties, opening for approach of substrates and reagents. It appears that the cis-auxiliary ligands ■ can be replaced by at least one of the reagents to give rise to the reacting intermediate, which is suitably oriented to favor a selective attack on one specific face, generating a good level of enantiodifferentiation.
A wide variety of metal cations can associate with chiral N,N′-dioxides to form efficient catalysts. The metal list includes rare-earth metals (e.g., Sc3+, Y3+, La3+, Nd3+, Sm3+, Eu3+, Gd3+, Yb3+), transition metals (e.g., Ti4+, Fe3+, Fe2+, Co2+, Ni2+, Cu2+, Cu+, Ag+), and main group metals (e.g., Mg2+, In3+) etc. Therefore, the asymmetric catalysis could also stem from the inherent properties of the metals, such as Lewis acidity, or coordination sphere properties. A difference in the ionic radius of metal ions can be leveraged by the conformational flexibility of the ligands. Table 1 shows some significant structural properties of four metal complexes.22–25 Depending on the structures of the N,N′-dioxides and the metal precursors, the complexes show distorted octahedral coordinations to some extent. For example, the X–ON1–ON2–Y torsion angles of the L2–complexes, with Sc(III)22 and Mg(II)25 as the chelated cations, are −11.28° and −6.80°, respectively (Table 1). Comparatively, the distortion away from octahedral geometry is much smaller with compound L4–Ni(II)23 than the others. It highlights the importance not only of the cation but also of ligand structure in the formation of the chiral messenger in the reactions.
Table 1 Selected geometrical parameters of M(N,N′-dioxide)
Metal salt |
Sc(OTf)3 |
Sc(OTf)3 |
Mg(OTf)2 |
Ni(BF4)2 |
Note: angle: ∠1 (ON1–M–OC1), ∠2 (ON2–M–OC2), ∠3 (OC1–M–OC2), ∠4 (X–M–Y); torsion angle: α1 (X–ON1–ON2–Y), α2 (NH1–CAr1–CAr2–NH2); X/Y*: (tetrahydrofuran-2-yl)methanol (Fig. 1b and ref. 22–25). |
N-Oxide |
L1
|
L2
|
L2
|
L4
|
X/Y |
H2O/OTf− |
H2O/OTf− |
H2O/H2O |
* |
∠1 (°) |
81.54 |
82.43 |
85.68 |
91.13 |
∠2 (°) |
83.17 |
82.16 |
85.68 |
92.40 |
∠3 (°) |
174.35 |
173.74 |
176.15 |
177.35 |
∠4 (°) |
87.89 |
87.71 |
81.175 |
77.79 |
α1 (°) |
−12.51 |
−11.28 |
−6.80 |
1.19 |
α2 (°) |
90.19 |
77.09 |
73.41 |
103.35 |
M–ON1 (Å) |
2.0374 |
2.0559 |
2.0096 |
1.9851 |
M–ON2 (Å) |
2.0390 |
2.0345 |
2.0153 |
1.9770 |
M–OC1 (Å) |
2.0972 |
2.0927 |
2.0640 |
2.0350 |
M–OC2 (Å) |
2.0809 |
2.1188 |
2.0443 |
2.0305 |
CCDC code |
704000 |
882608 |
804337 |
750144 |
Applications in asymmetric catalysis
A great number of asymmetric catalytic reactions can be catalysed by N,N′-dioxide–metal complexes under mild reaction conditions with simple procedures. Several representative examples reported after 2010 have been selected in Table 2 to showcase the diversity of enantioselective reactions realized.27 An early successful example is the hetero-Diels–Alder reaction between Danishefsky-type dienes and a wide range of aldehydes, which is efficiently catalysed by L4–In(OTf)3 complex in high yields, diastereoselectivities, and enantioselectivities.36 It was found that the activity of the pre-prepared catalyst could be maintained even after more than half a year. In some cases, the catalysts were also active without exclusion of water. For example, chiral N,N′-dioxide–Sc(OTf)3 systems could act as efficient catalysts for the asymmetric reduction of ketones with aq. KBH4 as the reducing reagent,33 and epoxidation of α,β-unsaturated carbonyl compounds using H2O2 (0.3%–30% aq.) without additives.38
Table 2 Selected asymmetric reactions catalysed by N,N′-dioxide–metal complexes
L/Metal |
Important applications |
L1/Y(OTf)3 |
Homologation of α-ketoesters and α-diazoesters28 |
L2/Sc(OTf)3 |
Roskamp–Feng reaction;29 electrophilic addition of α-diazoesters to ketones;30 ring expansion of isatins with α-diazoesters;22 Baeyer–Villiger oxidation;31 inverse electron-demand aza-Diels–Alder reaction;32 reduction reaction33 |
L2/Ni(BF4)2 |
[8 + 2] cycloaddition34 |
L3/Mg(ClO4)2 |
1,3-dipolar cycloaddition of nitrile imines35 |
L4/In(OTf)3 |
Hetero-Diels–Alder reaction36 |
L4/Sc(OTf)3 |
α-Functionalization of 3-substituted oxindoles,37 epoxidation of enones with H2O238 |
L4/Ni(ClO4)2 |
Ene reaction39 |
L5/Sc(OTf)3 |
Bromoamination40 and iodoamination reaction |
L6/Sc(OTf)3 |
α-Arylation of 3-substituted oxindoles41 |
The general applicability of chiral N,N′-dioxide–metal complexes make them useful not only for the practical synthesis of enantiomerically pure compounds but also for the discovery of novel asymmetric processes. Some examples are given to show the particular catalytic characteristics of chiral N,N′-dioxide–Sc(OTf)3 complexes in the control of chemo-, regio- and enantioselectivity. α-Diazocarbonyl compounds have versatile reactivity in organic synthesis.22,28–30 Using the highly efficient chiral L2–Sc(OTf)3 catalyst, different reactions of α-diazoesters occur with aldehydes and ketones. Asymmetric Roskamp reaction (also known as Roskamp–Feng reaction)42 is a challenging transformation, since it involves the addition of the α-diazoester to an aldehyde and a H-shift process in a chemo- and enantioselective fashion to yield chiral α-ketoesters without racemization. A series of α-alkyl-α-diazoesters with aromatic aldehydes could be used to give the chiral α-alkyl-β-ketoesters chemoselectively in 87–98% ee (Scheme 2a).29 The catalyst loading could be reduced to 0.05 mol%. It allowed for convenient separation of the catalyst and the product by flash filtration which thus prevented racemization of the product. However, an unexpected C–N bond forming reaction of the α-diazoester with ketone takes place with the same catalyst.30 This new reaction involves the electrophilic addition of α-diazoesters to α-alkyl ketones under mild reaction conditions. Optically active alkylhydrazone compounds are obtained in excellent yields (up to 98%) and enantioselectivities (up to 99% ee) for the first time (Scheme 2b). Additionally, L2–Sc(OTf)3 catalysed ring-expansion reaction of α-diazoesters and isatins gives 2-quinolone derivatives,22 whereas succinate derivatives are afforded from L1– or L4–Y(OTf)3 complex-promoted homologation of α-ketoesters and α-diazoesters.28 In another notable example, asymmetric haloamination of enones catalysed by L5–Sc(OTf)3 system provided chiral α-halo-β-amino ketones with up to 99% ee and 99
:
1 dr (Scheme 2c).12,40 The issues of the control of both regioselectivity and enantioselectivity of the reaction are well addressed.
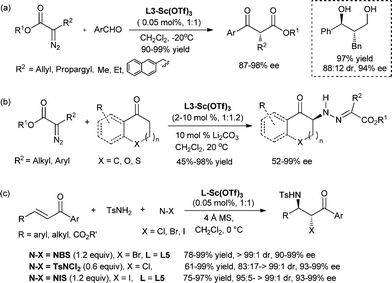 |
| Scheme 2 Asymmetric catalytic new reactions. | |
Conclusions
In general, the C2-symmetric N,N′-dioxide ligands developed by our group form well-organized tetradentate complexes with various metal salts. In a number of asymmetric reactions, N,N′-dioxide–metal complexes allow for a remarkable stereochemical outcome under mild reaction conditions. The popularity of privileged N,N′-dioxide ligands is due, at least in part, to their flexible configuration, perfectly C2-symmetric, as well as the array of structures accessible from readily available reagents and simple preparation. The exciting results obtained with such a flexible backbone will stimulate further opportunity to develop ideal chiral ligands. There are still unanswered questions concerning the process of the chirality transfer from the chiral N,N′-dioxide ligand–metal complex catalyst to the product. More detailed study is anticipated to shed light on the mechanism, which will make the behaviour of the catalyst predictable. We believe that chiral N,N′-dioxides will continue contributing to improving the chemistry of asymmetric catalysis.
Conflict of interests
The authors declare no competing financial interest.
Acknowledgements
We thank the National Natural Science Foundation of China (21321061), and the National Basic Research Program of China (973 Program: 2010CB833300) for financial support.
Notes and references
-
Comprehensive Asymmetric Catalysis, ed. E. N. Jacobsen, A. Pfaltz and H. Yamamoto, Springer, New York, 1999 Search PubMed.
-
New Frontiers in Asymmetric Catalysis, ed. K. Mikami and M. Lautens, John Wiley & Sons, Hoboken, NJ, 2007 Search PubMed.
-
Privileged chiral ligands and catalysts, ed. Q.-L. Zhou, Wiley-VCH, Weinheim, 2011 Search PubMed.
-
C. A. Sandoval and R. Noyori, in Organic Chemistry- Breakthroughs and Perspectives, ed. K.-L. Ding and L.-X. Dai, Wiley-VCH, Weinheim, 2012, ch. 9, pp. 335–363 Search PubMed.
- T. P. Yoon and E. N. Jacobsen, Science, 2003, 299, 1691 CrossRef CAS PubMed.
- J. H. Xie and Q.-L. Zhou, Acc. Chem. Res., 2008, 41, 581 CrossRef CAS PubMed.
- J. K. Whitesell, Chem. Rev., 1989, 89, 1581 CrossRef CAS.
- C. Moberg, Isr. J. Chem., 2012, 52, 653 CrossRef CAS.
- T. Clark and C. Landis, Tetrahedron: Asymmetry, 2004, 15, 2123 CrossRef CAS PubMed.
- G. Desimoni, G. Faita and K. A. Jørgense, Chem. Rev., 2011, 111, PR284 CrossRef CAS PubMed.
- X. H. Liu, L. L. Lin and X. M. Feng, Acc. Chem. Res., 2011, 44, 574 CrossRef CAS PubMed.
- S. X. Huang and K.-L. Ding, Angew. Chem., Ind. Ed., 2011, 123, 7878 CrossRef.
- N. Kumagai and M. Shibasaki, Angew. Chem., Int. Ed., 2013, 52, 223 CrossRef CAS PubMed.
- N. M. Karayannis, Coord. Chem. Rev., 1973, 93 CrossRef CAS.
- G. Chelucci, G. Murineddu and G. A. Pinna, Tetrahedron: Asymmetry, 2004, 15, 1373 CrossRef CAS PubMed.
- A. V. Malkov and P. Kočovský, Eur. J. Org. Chem., 2007, 29 CrossRef CAS.
- A. O'Neil, N. D. Miller, J. Peake, J. V. Barkley, C. M. R. Low and S. B. Kalindjian, Synlett, 1993, 515 CrossRef.
- A. O'Neil, N. D. Miller, J. V. Barkley, C. M. R. Low and S. B. Kalindjian, Synlett, 1995, 617 CrossRef PubMed.
- B. Liu, X. M. Feng, F. X. Chen, G. L. Zhang, X. Cui and Y. Z. Jiang, Synlett, 2001, 1551 CrossRef CAS.
- Y. C. Shen, X. M. Feng, G. L. Zhang and Y. Z. Jiang, Synlett, 2002, 1353 CAS.
- Y. H. Wen, X. Huang, J. L. Huang, Y. Xiong, B. Qin and X. M. Feng, Synlett, 2005, 2445 CAS.
- W. Li, X. H. Liu, X. Y. Hao, Y. F. Cai, L. L. Lin and X. M. Feng, Angew. Chem., Int. Ed., 2012, 51, 8644 CrossRef CAS PubMed.
- K. Zheng, X. H. Liu, J. N. Zhao, Y. Yang, L. L. Lin and X. M. Feng, Chem. Commun., 2010, 46, 3771 RSC.
- Y. L. Liu, D. J. Shang, X. Zhou, X. H. Liu and X. M. Feng, Chem.–Eur. J., 2009, 15, 2055 CrossRef CAS PubMed.
- K. Zheng, C. K. Yin, X. H. Liu, L. L. Lin and X. M. Feng, Angew. Chem., Int. Ed., 2011, 50, 2573 CrossRef CAS PubMed.
- K. Matsumoto, B. Saito and T. Katsuki, Chem. Commun., 2007, 3619 RSC.
- The earlier references have been covered in a recent review, see ref. 11. The aim here is to cover the updated developments in our group.
- W. Li, X. H. Liu, F. Tan, X. Y. Hao, J. F. Zheng, L. L. Lin and X. M. Feng, Angew. Chem., Int. Ed., 2013, 52, 10883 CrossRef CAS PubMed.
- W. Li, J. Wang, X. L. Hu, K. Shen, W. T. Wang, Y. Y. Chu, L. L. Lin, X. H. Liu and X. M. Feng, J. Am. Chem. Soc., 2010, 132, 8532 CrossRef CAS PubMed.
- W. Li, X. H. Liu, X. Y. Hao, X. L. Hu, Y. Y. Chu, W. D. Cao, S. Qin, C. W. Hu, L. L. Lin and X. M. Feng, J. Am. Chem. Soc., 2011, 133, 15268 CrossRef CAS PubMed.
- L. Zhou, X. H. Liu, J. Jie, Y. H. Zhang, L. L. Lin and X. M. Feng, J. Am. Chem. Soc., 2012, 134, 17023 CrossRef CAS PubMed.
- M. S. Xie, X. H. Chen, Y. Zhu, B. Gao, L. L. Lin, X. H. Liu and X. M. Feng, Angew. Chem., Int. Ed., 2010, 49, 3799 CrossRef CAS PubMed.
- P. He, X. H. Liu, H. F. Zheng, W. Li, L. L. Lin and X. M. Feng, Org. Lett., 2012, 14, 5134 CrossRef CAS PubMed.
- M. S. Xie, X. H. Liu, X. X. Wu, Y. F. Cai, L. L. Lin and X. M. Feng, Angew. Chem., Int. Ed., 2013, 52, 5604 CrossRef CAS PubMed.
- G. Wang, X. H. Liu, T. Y. Huang, Y. L. Kuang, L. L. Lin and X. M. Feng, Org. Lett., 2013, 15, 76 CrossRef CAS PubMed.
- Z. P. Yu, X. H. Liu, L. Zhou, L. L. Lin and X. M. Feng, Angew. Chem., Int. Ed., 2009, 48, 5195 CrossRef CAS PubMed.
- J. Li, Y. F. Cai, W. L. Chen, X. H. Liu, L. L. Lin and X. M. Feng, J. Org. Chem., 2012, 77, 9148 CrossRef CAS PubMed.
- Y. Y. Chu, X. H. Liu, W. Li, X. L. Hu, L. L. Lin and X. M. Feng, Chem. Sci., 2012, 3, 1996 RSC.
- K. Zheng, X. H. Liu, S. Qin, M. S. Xie, L. L. Lin, C. W. Hu and X. M. Feng, J. Am. Chem. Soc., 2012, 134, 17564 CrossRef CAS PubMed.
- Y. F. Cai, X. H. Liu, Y. H. Hui, J. Jiang, W. T. Wang, W. L. Chen, L. L. Lin and X. M. Feng, Angew. Chem., Int. Ed., 2010, 49, 6160 CrossRef CAS PubMed.
- J. Guo, S. X. Dong, Y. L. Zhang, Y. L. Kuang, X. H. Liu, L. L. Lin and X. M. Feng, Angew. Chem., Int. Ed., 2013, 52, 10245 CrossRef CAS PubMed.
-
Organic Syntheses Based on Name Reactions, ed. A. Hassner and I. Namboothiri, Elsevier, Oxford, 3rd edn, 2011, p. 408 Search PubMed.
Footnote |
† Electronic supplementary information (ESI) available. See DOI: 10.1039/c3qo00059a |
|
This journal is © the Partner Organisations 2014 |
Click here to see how this site uses Cookies. View our privacy policy here.