DOI:
10.1039/C4QO00191E
(Research Article)
Org. Chem. Front., 2014,
1, 983-987
Synthesis and applications of 1-iodo-4-MgCl-1,3-dienes and 1-iodovinyl phenylmagnesium chlorides†
Received
8th July 2014
, Accepted 4th August 2014
First published on 8th August 2014
Abstract
Selective I/Mg exchange reactions of 1,4-diiodo-1,3-dienes and o-iodo-2-(2-iodovinyl)benzenes were achieved viaiPrMgCl·LiCl. Various 1-iodo-4-MgCl-1,3-dienes and 1-iodovinyl phenylmagnesium chlorides were thus efficiently generated and synthetically applied to afford new C–C bonds via reactions of the alkenyl or aryl C–MgCl bonds with different electrophiles. Useful conjugated compounds including polysubstituted benzenes, naphthalenes and phosphine compounds could be synthesized readily via further applications of the remaining alkenyl C–I bonds and subsequent cyclization reactions.
Introduction
Dihalo compounds such as 1a and 1b (Scheme 1) have become readily available.1 From such dibromides or diiodides, their corresponding organo-di-metallic compounds (reagents), including 1,4-dilithio-1,3-dienes,2 1,4-dicopper-1,3-dienes3 and 1,4-dimagnesia-1,3-dienes,4 can be easily generated (Model I, Scheme 1). The reaction chemistry study of these organo-di-metallic reagents has demonstrated their unique and useful synthetic applications because of the cooperative effect of the two C–M bonds in the same molecule.2–5 Conceptually, if one of the two C–X bonds could be selectively metalated, compounds containing one C–X bond and one C–M bond in the same molecule would be generated (Model II, Scheme 1). Useful synthetic applications of such compounds can thus be expected because the C–X bond and the C–M bond will react with totally different reagents. For example, the C–M bond will react with various electrophiles while the C–X bond can be applied to numerous cross-coupling reactions.
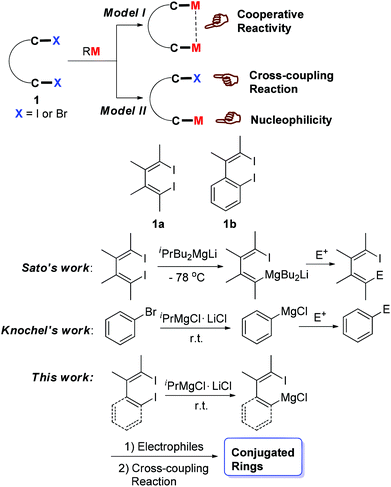 |
| Scheme 1 Halogen/metal exchange reactions of dihalo compounds. | |
The halogen/metal exchange reaction is a general and important strategy to prepare organometallic reagents.6 To realize the above-mentioned selective mono-metallation reaction, we first tried to obtain 1-lithio-4-iodo-1,3-dienes via mono-lithiation of 1,4-diiodo-1,3-dienes 1a with organolithium reagents. However, the selectivity of such I/Li exchange reaction was not satisfactory. In addition to the expected 1-lithio-4-iodo-1,3-dienes, 1,4-dilithio-1,3-dienes were always generated in non-negligible amounts, and a rather low reaction temperature was required in this reaction. We then considered trying other milder halogen/metal exchange reactions. In 2003, Sato et al. reported a site-selective I/Mg exchange reaction of 1,4-diiodo-1,3-dienes using an organomagnesium ate complex (Scheme 1).7 In 2004, Knochel et al. reported a LiCl-mediated Br/Mg exchange reaction to prepare functionalized aryl compounds from organic bromides under mild conditions.8 This method greatly promoted the accessibility of functionalized Grignard reagents in organic synthesis.9 Motivated by Sato and Knochel's pioneering work, herein we report a selective mono I/Mg exchange reaction of 1,4-diiodo-1,3-dienes (1a) and o-iodo-2-(2-iodovinyl)benzenes (1b) viaiPrMgCl·LiCl (Knochel reagent). Various 1-iodo-4-MgCl-1,3-dienes and 1-iodovinyl phenylmagnesium chlorides were thus efficiently generated and synthetically applied.
Results and discussion
Selective mono I/Mg exchange reactions of 1,4-diiodo-1,3-dienes (1a) and o-iodo-2-(2-iodovinyl)benzenes (1b)
We first chose the tetraethyl 1,4-diiodo-1,3-diene 1a1 to react with iPrMgCl·LiCl in THF at room temperature. Results are shown in Scheme 2. It was found that this mono I/Mg exchange reaction could selectively afford the desired 1-iodo-4-magnesia-1,3-diene Ia, which upon hydrolysis gave its corresponding mono iodide 2a1. The undesired dimagnesia-1,3-diene IIa was not generated in most cases. With 1 or 2 equivalents of the Knochel reagent, compound 2a1 could be obtained in moderate to good yields, but remarkable amounts of diiodide 1a1 remained unreacted, even after 6 h (entry 4). This observation indicated that this I/Mg exchange reaction was slow. With 4 equivalents of iPrMgCl·LiCl, the reaction completed after 6 h, affording the product 2a1 in 75% isolated yield after hydrolysis (entry 6).
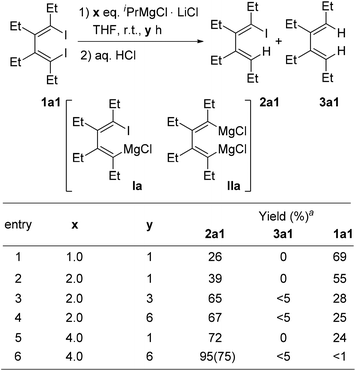 |
| Scheme 2 I/Mg exchange reaction conditions of 1,4-diiodo-1,3-diene 1a1. a GC yields. Isolated yields are given in parenthesis. | |
For the I/Mg exchange reaction of o-iodo-2-(2-iodovinyl)benzenes 1b with the Knochel reagent, the exchange reaction rate was found to be faster than that of 1,4-diiodo-1,3-dienes 1a. As shown in Scheme 3, the I/Mg exchange reaction of 1b1 with the Knochel reagent would generate Ib, Ib′ and IIb. However, no formation of the product 2b1′ was detected in all the cases, which indicated that the alkenyl C–I bond did not undergo the I/Mg exchange reaction. The aryl C–I bond was selectively magnesiated. This high selectivity is probably because the aryl C–I bond is more reactive than the alkenyl C–I bond in the I/Mg exchange reaction and the possible transition state is considered resembling the hypervalent halogen complex, for which an aryl ring could provide more stabilization to the transition state than an alkenyl via delocalization of the charge on halogen.9 With 2 equivalents of iPrMgCl·LiCl, the reaction of 1b1 completed after 3 h, affording the product 2b1 in 77% isolated yield after hydrolysis.
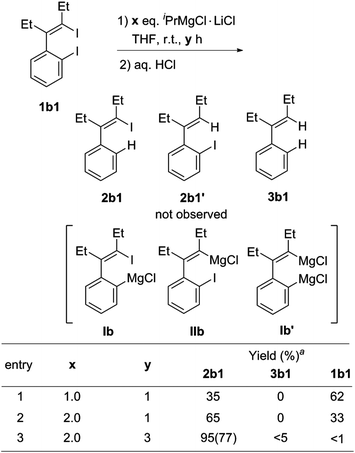 |
| Scheme 3 I/Mg exchange reaction conditions of o-iodo-2-(2-iodovinyl)benzene 1b1. a GC yields. Isolated yields are given in parenthesis. | |
Reactions of in situ generated 1-iodo-4-MgCl-1,3-dienes Ia and 1-iodovinyl phenylmagnesium chlorides Ib with electrophiles
New C–C bonds were highly efficiently generated when the in situ generated 1-iodo-4-MgCl-1,3-dienes Ia and 1-iodovinyl phenylmagnesium chlorides Ib were treated with electrophiles, such as allyl bromide and carbon dioxide (Schemes 4 and 5). In addition, the C–MgCl bond could be easily transformed to the C–B bond in high yields when treated with isopropoxyboronic acid pinacol ester.
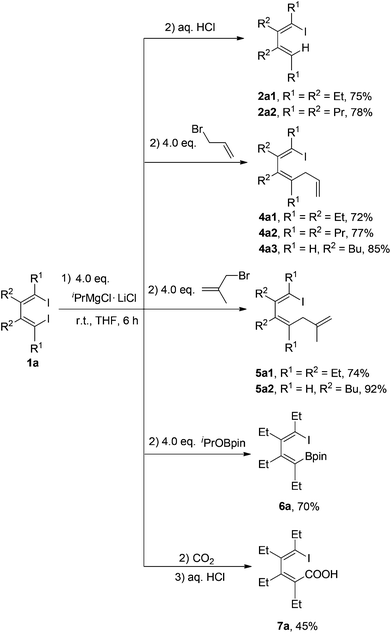 |
| Scheme 4
In situ generation of 1-iodo-4-magnesia-1,3-dienes Ia and their reactions with electrophiles. | |
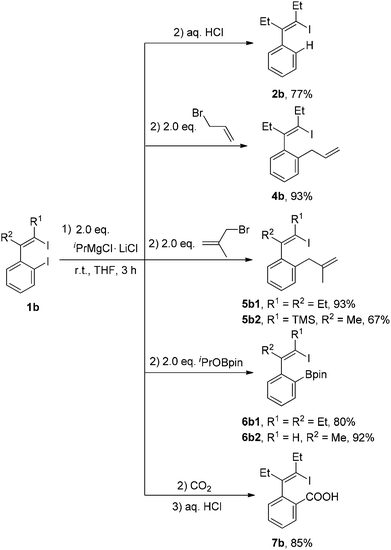 |
| Scheme 5
In situ generation of 1-iodovinyl phenylmagnesium chlorides Ib and their reactions with electrophiles. | |
Intramolecular Heck reaction of 1-iodo-1,3-diene analogues
As shown in Scheme 6, 1-iodo-1,3-butadiene analogues 4a and 5a were tested in the intramolecular Heck reaction.10 Pentasubstituted benzenes 8a were obtained in high yields via an intramolecular Heck reaction and a subsequent aromatization step. For 5a with an extra methyl group in the allyl unit, cycloheptatriene products 9a, other than the benzene-type products 8a, were generated in high yields. Cycloheptatrienes are useful and structurally interesting compounds.11 This synthetic method offered a new way to build such polysubstituted cycloheptatrienes.
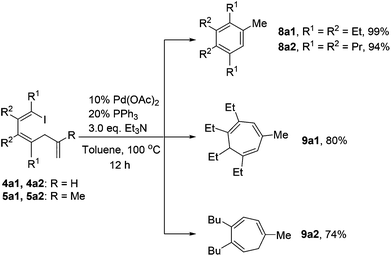 |
| Scheme 6 Intramolecular Heck reaction of allyl 1-iodo-1,3-diene derivatives. | |
As given in Scheme 7, compound 4b could also be converted into their corresponding polysubstituted naphthalenes 8b readily in high yields via a similar intramolecular Heck reaction and a subsequent aromatization step. It should be noted that these polysubstituted benzenes 8a and naphthalenes 8b are very difficult to synthesize by other methods.12 However, when we chose compound 5b1 with an extra methyl group to undergo this Heck reaction under the same conditions, a novel compound 9b1 containing a bicyclo[4.1.0] ring was obtained in a moderate yield (Scheme 7). Similarly, when compound 5b2 was used, the product 9b2 was obtained in 70% isolated yield.
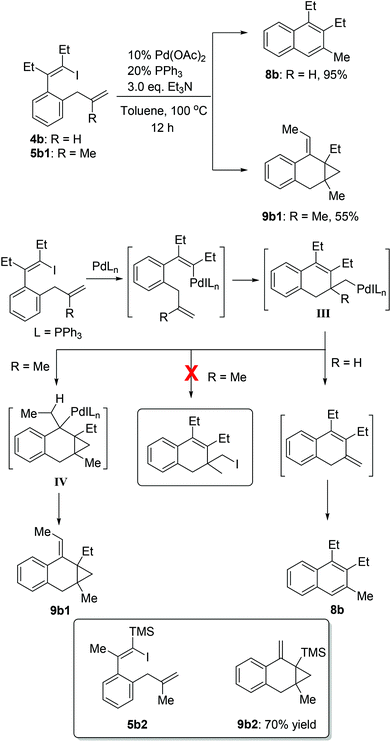 |
| Scheme 7 Intramolecular Heck reaction of allyl 2-iodovinylbenzene derivatives. | |
A proposed mechanism is shown in Scheme 7. After the oxidative addition of palladium and the subsequent intramolecular alkene insertion, the pivotal intermediate III was generated. When intermediate III had a β-hydrogen atom (R = H), the β-hydride elimination could occur to give the naphthalene product 8b after aromatization. When there was no β-hydrogen atom (R = Me) in intermediate III, no β-hydride elimination could occur. In this situation, the intermediate III would undergo the second intramolecular alkene insertion to offer the intermediate IV. Since the intermediate IV had syn-β hydrogens, the subsequent β-hydride elimination of IV would thus happen and lead to the final products 9b1. Recently, reductive elimination of alkyl halides from alkylpalladium halide species without β-hydrogens has attracted much attention.13,14 However, in our cases (R = Me), no corresponding C(sp3)–I reductive elimination product was observed, indicating that the intramolecular alkene insertion was more favored than C(sp3)–I reductive elimination.
Synthesis of the functionalized phosphine compound starting from 1-iodo-1,3-diene analogues
As further demonstration of the synthetic utility of the designed compounds containing one C–I bond and one C–M bond in the same molecule, we synthesized the phosphine compound 10 from 6b2 and Ph2PH in 73% isolated yield (Scheme 8). The structure of 10 was confirmed by single crystal X-ray structural analysis (Fig. 1).
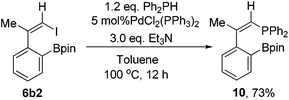 |
| Scheme 8 Synthesis of phosphine ligands. | |
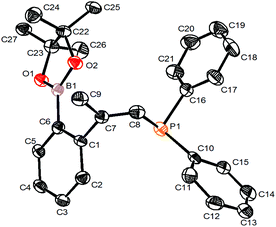 |
| Fig. 1 ORTEP drawings of 10 with 30% thermal ellipsoids. Hydrogen atoms are omitted for clarity. | |
Conclusions
In summary, we have reported herein the I/Mg exchange reactions of 1,4-diiodo-1,3-dienes 1a and o-iodo-2-(2-iodovinyl)benzenes 1b and their synthetic applications. The reactions efficiently afforded diverse 1-iodo-4-magnesia-1,3-dienes and 1-iodovinyl phenylmagnesium chlorides in situ. After they were quenched with different electrophiles, various functionalized derivatives were obtained. Most of the reactions were under mild conditions. By taking advantage of the rich reactivity of the derivatives, useful conjugated compounds including polysubstituted benzenes, naphthalenes and phosphine compounds could be synthesized readily via the subsequent cross-coupling reactions.
Acknowledgements
This work was supported by the 973 Program (2012CB821600) and the National Natural Science Foundation of China (NSFC).
Notes and references
- For a recent review of 1,4-diiodo-1,3-dienes, see: V. P. Ananikov, O. V. Hazipov and I. P. Beletskaya, Chem. – Asian. J., 2011, 6, 306 CrossRef CAS PubMed
.
- Z. Xi, Acc. Chem. Res., 2010, 43, 1342 CrossRef CAS PubMed
.
-
(a) J. Wei, Z. Wang, W.-X. Zhang and Z. Xi, Org. Lett., 2013, 15, 1222 CrossRef CAS PubMed
;
(b) S. Zhang, M. Zhan, Q. Wang, C. Wang, W.-X. Zhang and Z. Xi, Org. Chem. Front., 2014, 1, 130 RSC
;
(c) W. Geng, J. Wei, W.-X. Zhang and Z. Xi, J. Am. Chem. Soc., 2014, 136, 610 CrossRef CAS PubMed
.
-
(a) H. Fang, G. Li, G. Mao and Z. Xi, Chem. – Eur. J., 2004, 10, 3444 CrossRef CAS PubMed
;
(b) J. Wei, L. Liu, M. Zhan, L. Xu, W.-X. Zhang and Z. Xi, Angew. Chem., Int. Ed., 2014, 53, 5634 CrossRef CAS PubMed
.
-
(a) Z. Xi and Q. Song, J. Org. Chem., 2000, 65, 9157 CrossRef CAS PubMed
;
(b) Z. Xi, Q. Song, J. Chen, H. Guan and P. Li, Angew. Chem., Int. Ed., 2000, 40, 1913 CrossRef
.
- For a recent review of halogen/metal exchange reactions, see: D. Tilly, F. Chevallier, F. Mongin and P. C. Gros, Chem. Rev., 2014, 114, 1207 CrossRef CAS PubMed
.
-
(a) K. Kitagawa, A. Inoue, H. Shinokubo and K. Oshima, Angew. Chem., Int. Ed., 2000, 43, 2481 CrossRef
;
(b) K. Fukuhara, Y. Takayama and F. Sato, J. Am. Chem. Soc., 2003, 125, 6884 CrossRef CAS PubMed
.
- A. Krasovskiy and P. Knochel, Angew. Chem., Int. Ed., 2004, 43, 3333 CrossRef CAS PubMed
.
-
(a) A. H. Stoll, A. Krasovskiy and P. Knochel, Angew. Chem., Int. Ed., 2006, 45, 606 CrossRef CAS PubMed
;
(b) A. Krasovskiy, V. Krasovskaya and P. Knochel, Angew. Chem., Int. Ed., 2006, 45, 2958 CrossRef CAS PubMed
;
(c) A. Krasovskiy, B. F. Straub and P. Knochel, Angew. Chem., Int. Ed., 2006, 45, 159 CrossRef CAS PubMed
;
(d) L. Shi, Y. Chu, P. Knochel and H. Mayr, J. Org. Chem., 2009, 74, 2760 CrossRef CAS PubMed
;
(e) L. Shi, Y. Chu, P. Knochel and H. Mayr, Angew. Chem., Int. Ed., 2008, 47, 202 CrossRef CAS PubMed
;
(f) G. Manolikakes and P. Knochel, Angew. Chem., Int. Ed., 2009, 48, 205 CrossRef CAS PubMed
;
(g) L. Shi, Y. Chu, P. Knochel and H. Mayr, Org. Lett., 2012, 14, 2602 CrossRef CAS PubMed
.
- For a review on intramolecular Heck reaction, see:
(a)
S. Bräse and A. de Meijere in Handbook of Organopalladium Chemistry for Organic Synthesis, ed. E. Negishi, John Wiley & Sons, New York, 2002, vol. 1, pp. 1223–1254 Search PubMed
For a recent review on the roles of bases in transition-metal catalyzed reactions, see:
(b) K. Ouyang and Z. Xi, Acta Chim. Sin., 2013, 71, 13 CrossRef CAS
For recent papers on intramolecular Heck reaction, see:
(c) W. Cong, L. Zhao, X. Wu, J. Xu and H. Yao, Tetrahedron, 2014, 70, 312 CrossRef CAS PubMed
;
(d) W. Wang, J.-T. Lu, H.-L. Zhang, Z.-F. Shi, J. Wen and X.-P. Cao, J. Org. Chem., 2014, 79, 122 CrossRef CAS PubMed
;
(e) M. Lei, W. Tian, W. Li, P. Lu and Y. Wang, Tetrahedron, 2014, 70, 3665 CrossRef CAS PubMed
;
(f) G. Symkenberg and M. Kalesse, Angew. Chem., Int. Ed., 2014, 53, 1795 CrossRef CAS PubMed
.
- For selected papers of cycloheptatrienes, see:
(a) H. E. Winberg, J. Org. Chem., 1959, 24, 264 CrossRef CAS
;
(b) H.-M. Steuhl, C. Bornemann and M. Klessinger, Chem. – Eur. J., 1999, 5, 2404 CrossRef CAS
;
(c) M. L. H. Green, Chem. Rev., 1995, 95, 439 CrossRef CAS
;
(d) R. V. Williams, W. D. Edwards, P. Zhang, D. J. Berg and R. H. Mitchell, J. Am. Chem. Soc., 2012, 134, 16742 CrossRef CAS PubMed
;
(e) M. Xie, X. Liu, X. Wu, Y. Cai, L. Lin and X. Feng, Angew. Chem., Int. Ed., 2013, 52, 5604 CrossRef CAS PubMed
.
-
(a) V. Gevorgyan, K. Tando, N. Uchiyama and Y. Yamamoto, J. Org. Chem., 1998, 63, 7022 CrossRef CAS PubMed
;
(b) T. Takahashi, F.-Y. Tsai, Y. Li, K. Nakajima and M. Kotora, J. Am. Chem. Soc., 1999, 121, 11093 CrossRef CAS
.
- For a recent review on reductive elimination of alkyl halides from alkyl palladium(II) halides, see:
(a) C. Chen and X. Tong, Org. Chem. Front., 2014, 1, 439 RSC
;
(b) S. G. Newman and M. Lautens, J. Am. Chem. Soc., 2011, 133, 1778 CrossRef CAS PubMed
;
(c) H. Liu, C. Chen, L. Wang and X. Tong, Org. Lett., 2011, 13, 5072 CrossRef CAS PubMed
;
(d) H. Liu, C. Li, D. Qiu and X. Tong, J. Am. Chem. Soc., 2011, 133, 6187 CrossRef CAS PubMed
;
(e) S. G. Newman, J. K. Howell, N. Nicolaus and M. Lautens, J. Am. Chem. Soc., 2011, 133, 14916 CrossRef CAS PubMed
;
(f) D. A. Petrone, H. A. Malik, A. Clemenceau and M. Lautens, Org. Lett., 2012, 14, 4806 CrossRef CAS PubMed
;
(g) X. Jia, D. A. Petrone and M. Lautens, Angew. Chem., Int. Ed., 2012, 51, 9870 CrossRef CAS PubMed
;
(h) D. A. Petrone, M. Lischka and M. Lautens, Angew. Chem., Int. Ed., 2013, 52, 10635 CrossRef CAS PubMed
.
- For computational study of reductive elimination, see:
Y. Lan, P. Liu, S. G. Newman, M. Lautens and K. N. Houk, Chem. Sci, 2012, 3, 1987 Search PubMed.
Footnote |
† Electronic supplementary information (ESI) available: General information, experimental procedures, characterization data, copies of 1H NMR and 13C NMR spectra, and crystal data of compound 10. CCDC 1011067. For ESI and crystallographic data in CIF or other electronic format see DOI: 10.1039/c4qo00191e |
|
This journal is © the Partner Organisations 2014 |
Click here to see how this site uses Cookies. View our privacy policy here.