DOI:
10.1039/C4QO00205A
(Research Article)
Org. Chem. Front., 2014,
1, 1176-1179
Cu-mediated C–H cyanation of arenes using N,N-dimethylformamide (DMF) as the “CN” source†
Received
22nd July 2014
, Accepted 17th September 2014
First published on 18th September 2014
Abstract
This paper describes the direct C–H bond cyanation of 2-phenylpyridines employing N,N-dimethylformamide (DMF) as both the reagent and solvent. DMF provides both the C and N units in the generated “CN” group in this cyanation transformation. This reaction gives a novel protocol with high atom economy to extend the application of DMF and provides an alternative approach to aryl nitriles.
Developing new methodologies with high atom economy1 has been one of the most attractive goals in organic synthesis. In this context, scientists have recently developed lots of novel maneuvers to construct complex compounds from simple and readily available starting materials. Despite the fact that DMF has been widely used in organic synthesis as one of the popular polar solvents, the exploration of novel transformation of DMF, a versatile building block, has been significantly improved in the past few decades.2
Cyanation reaction is one of the important transformations due to the wide applications of the nitrile products in organic synthesis.3–5 Traditionally, the transition-metal catalyzed cyanation reactions6,7 often employ metal cyanides such as MCN (M = Cu, K, Na, Zn), TMSCN and K3Fe(CN)6 as the cyanating reagents, most of which are toxic and environmentally unfriendly. Very recently, a few of new organocyanating reagents were identified,6 such as nitromethane,6a tosyl cyanide,6e ethyl cyanoacetate,6f isonitrile,6g,o benzyl cyanide,6h acetonitrile,6i,k,n AIBN (2,2′-azobisisobutyronitrile)6j and NCTS (N-cyano-N-phenyl-p-toluenesulfonamide).6l,6m,6p
Significantly, elegant work has been reported by Chang and co-workers using DMF and ammonia or ammonium salts as the combined “CN” source to realize the cyanation of the carbon–hydrogen (heteroatom) bond (a, Scheme 1).8 Cheng et al. also realized the cyanation of aromatic halides by employing ammonium bicarbonate and DMF (or DMSO) as the combined “CN” source.9 In these reported cyanation reactions involving the combined “CN” source, DMF or DMSO provides the “C” unit of “CN”, while the “N” unit comes from ammonia or ammonium salts.8,9
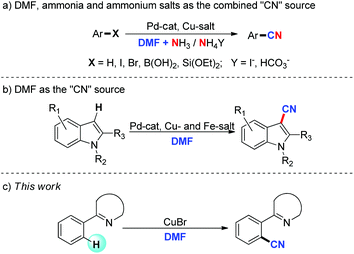 |
| Scheme 1 DMF participates in different cyanation reactions. | |
Our group recently demonstrated a cyanation reaction of indoles by the direct C–H functionalization process (b, Scheme 1).10 To the best of our knowledge, this is the first and only report that DMF provides both the “C” and “N” units of the “CN” moiety in this cyanation reaction. However, the reaction is limited to indole substrates. Moreover, the reaction conditions are too complex. Besides the palladium catalyst, four kinds of other additives including Cu- and Fe-salts are required for this transformation. Thus, development of more concise systems to realize direct C–H cyanation with different substrates is still desirable. Herein, we described a direct C–H bond cyanation of pyridyl arenes using DMF alone as the source of “CN” (c, Scheme 1).
We initiated our study with 2-phenylpyridine (1a)11 as a model substrate (Table 1). To our delight, when the reaction of 1a was conducted under O2, the desired ortho cyanation product 2a was obtained in 10% yield in the presence of CuBr2. The screening of copper salts showed that different copper species showed different efficiency in this cyanation reaction. Compared to CuBr2, CuI, Cu(OTf)2 and CuCN, the reaction with CuBr afforded the best result (entries 1–5, Table 1). Subsequently, the effects of different ligands were investigated (entries 6–8, Table 1). It was found that the reaction efficiency could be improved by adding 50 mol% of L1 (entry 6, Table 1). Attempts using other representive ligands which usually worked well with copper gave much lower yields (entries 7 and 8, Table 1). When a proton acid was added, such as acetic acid, the reaction was totally inhibited (entry 9, Table 1). Bases did not work either. The efficiency decreased drastically when TBD (1,3,4,6,7,8-hexahydro-2H-pyrimido[1,2-a]pyrimidine) or potassium bicarbonate was employed (entries 10 and 11, Table 1). When the equivalent of L1 declined to 25 mol%, the yield remained the same (entry 12, Table 1). Interestingly, when the reaction mixture was detected by GC-MS, we found that L1 decomposed to benzil (L4) under these conditions.12 Consequently, benzil which is much cheaper than L1 was employed as the optimal ligand (entry 13, Table 1). However, lower yield was obtained when the loading of L4 was reduced to 10 mol% (entry 14, Table 1). Further studies indicate that O2 is vital for the cyanation process due to the striking decrease in yields when the reactions were carried out under argon or air (entries 15 and 16, Table 1).
Table 1 Copper-mediated cyanation of 2-phenylpyridinea
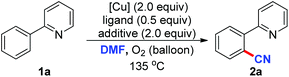
|
Entry |
[Cu] |
Ligand |
Additive |
Yieldb (%) |
Reaction conditions: 1a (0.4 mmol), DMF (3 mL), O2 (1 atm), 48 h.
Isolated yields.
25 mol% of L4 was used.
10 mol% of L4 was used.
The reaction was carried out under argon.
The reaction was carried out under air. TBD = 1,3,4,6,7,8-hexahydro-2H-pyrimido[1,2-a]pyrimidine.
|
1 |
CuBr2 |
— |
— |
10 |
2 |
CuI |
— |
— |
38 |
3 |
Cu(OTf)2 |
— |
— |
Trace |
4 |
CuCN |
— |
— |
Trace |
5 |
CuBr |
— |
— |
43 |
6 |
CuBr |
L1
|
— |
61 |
7 |
CuBr |
L2
|
— |
Trace |
8 |
CuBr |
L3
|
— |
Trace |
9 |
CuBr |
L1
|
AcOH |
0 |
10 |
CuBr |
L1
|
TBD |
33 |
11 |
CuBr |
L1
|
KHCO3 |
Trace |
12c |
CuBr |
L1
|
— |
61 |
13
|
CuBr
|
L4
|
—
|
61
|
14d |
CuBr |
L4
|
— |
47 |
15c,e |
CuBr |
L4
|
— |
0 |
16c,f |
CuBr |
L4
|
— |
Trace |
|
With the optimal conditions in hand, we next investigated the substrate scope of this cyanation reaction (Table 2). Although many conditions have been screened, the reactions did not execute completely for some substrates. The remaining substrates could be recovered after the reactions. Products (2b–2f, 2h–2k) with different substituent groups on the phenyl rings were obtained in moderate yields. However, using methyl 4-(pyridin-2-yl)benzoate (1g) gave the desired product in poor yield. The sites of substituent groups on the phenyl ring did not affect the efficiency of this transformation (2h–2j). Substituted pyridyl (at the 3-position or 5-position) groups were also tolerant in this direct cyanation reaction (2l–2m). In addition, the substrates were also compatible with the optimal conditions when the pyridyl groups were substituted by fused aromatic rings (1n and 1q). It is noteworthy that 2-(naphthalen-2-yl)pyridine (1q) gave the products 2q and 2q′ as a mixture in 2.7
:
1 molar ratio. Meanwhile, a dicyanated product 2r was obtained in 12% yield. Furthermore, 1-phenylisoquinoline (1o) and benzo[h]quinoline (1p) worked smoothly under the optimized conditions to afford the desired products 2o and 2p in 35% and 44% yield, respectively.
Table 2 Copper-mediated cyanation of pyridyl arenes with DMFa
Standard reaction conditions: 1 (0.4 mmol), CuBr (0.8 mmol), DMF (3 mL), 135 °C, O2 (1 atm), 48 h. Isolated yields. The number in the parentheses is the recovery of the substrates.
DMF (1 mL).
0.2 mmol L1 instead of L4 was used.
|
|
In order to probe the mechanism, some control experiments were investigated (Table 3). When the reaction was carried out in the presence of 2.0 equivalents of 2,2,6,6-tetramethyl-1-piperidinyloxy (TEMPO), the efficiency of this transformation did not decrease (entry 1, Table 3), which might rule out a single electron transfer (SET) process. When N,N-dimethylacetamide (DMA) was used as the solvent instead of DMF, no cyanation product 2a was obtained (entry 2, Table 3). It is noteworthy that when CuCN which could provide the “CN” source was employed instead of CuBr in DMF, no expected product 2a was detected (entry 3, Table 3). This result indicates that the tandem processes including in situ formation of “CN” from DMF and the subsequent C–H cyanation step are not involved in this novel transformation.
Table 3 Mechanistic studies with the control reactions of 1aa
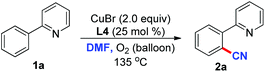
|
Entry |
Variation from the standard conditions |
Yieldb (%) |
For the standard conditions, see entry 13, Table 1.
Isolated yields.
1l was used instead of 1a. TEMPO = 2,2,6,6-tetramethyl-1-piperidinyloxy, DMA = N,N-dimethylacetamide.
|
1 |
Additional TEMPO (2.0 eq.) was added |
60 |
2 |
DMA instead of DMF |
0 |
3 |
CuCN (2.0 eq.) instead of CuBr |
0 |
4 |
Additional H2O (20.0 eq.) was added |
59 |
5c |
Additional NH4I (1.2 eq.) was added |
9 |
Moreover, when additional water (20.0 eq.) was employed under the standard conditions, the reaction produced 2a in 59% yield (entry 4, Table 3), which demonstrates that water did not influence the reaction. Notably, the negative effect was obtained with only 9% yield when NH4I (1.2 eq.), a crucial reagent in Chang's work,8b–d was added under the standard conditions (entry 5, Table 3), which suggests that the mechanism of the present chemistry is totally different from that of Chang's reactions. However, the reaction mechanism is still not clear yet.
In conclusion, we have developed a novel Cu-mediated C–H bond cyanation reaction of arenes using the pyridyl group as a directing group. DMF provides both the “C” and “N” units in the generated “CN” group in this cyanation transformation. This reaction gives a novel protocol with high atom economy to extend the application of DMF and provides an alternative approach to prepare aryl nitriles.
Financial support from the National Science Foundation of China (no. 21325206, 21172006), the National Young Top-notch Talent Support Program, and the PhD. Programs Foundation of the Ministry of Education of China (no. 20120001110013) is greatly appreciated.
Notes and references
-
(a) B. M. Trost, Angew. Chem., Int. Ed. Engl., 1995, 34, 259 CrossRef CAS;
(b) B. M. Trost, Science, 1991, 254, 1471 CAS.
-
(a) S. Ding and N. Jiao, Angew. Chem., Int. Ed., 2012, 51, 9226 CrossRef CAS PubMed;
(b) J. Muzart, Tetrahedron, 2009, 65, 8313 CrossRef CAS PubMed;
(c) S. Brahma and J. K. Ray, Tetrahedron, 2008, 64, 2883 CrossRef CAS PubMed;
(d) I. Pastoriza-Santos and L. M. Liz-Marzan, Adv. Funct. Mater., 2009, 19, 679 CrossRef CAS.
-
(a)
Fatiadi and A. J. Ln, Preparation and Synthetic Applications of Cyano Compounds, ed. S. Patai and Z. Rappaport, Wiley, New York, 1983 Search PubMed;
(b)
R. C. Larock, Comprehensive Organic Transformations, VCH, New York, 1989 Search PubMed;
(c)
A. Kleemann, J. Engel, B. Kutscher and D. Reichert, Pharmaceutical S J.ubstances: Synthesis, Patents, Applications, Thieme, Stuttgart, Germany, 4th edn, 2001 Search PubMed;
(d) J. S. Miller and J. L. Manson, Acc. Chem. Res., 2001, 34, 563 CrossRef CAS PubMed.
-
(a) T. Sandmeyer, Ber. Dtsch. Chem. Ges., 1884, 17, 2650 CrossRef;
(b) T. Sandmeyer, Ber. Dtsch. Chem. Ges., 1885, 18, 1492 CrossRef;
(c) H. H. Hodgson, Chem. Rev., 1947, 40, 251 CrossRef CAS;
(d) C. Galli, Chem. Rev., 1988, 88, 765 CrossRef CAS;
(e) K. W. Rosenmund and E. Struck, Ber. Dtsch. Chem. Ges., 1919, 52, 1749 CrossRef;
(f) J. von Braun and G. Manz, Justus Liebigs Ann. Chem., 1931, 111 CrossRef.
-
(a) K. Ishihara, Y. Furuya and H. Yamamoto, Angew. Chem., Int. Ed., 2002, 41, 2983 CrossRef CAS;
(b) C.-W. Kuo, J.-L. Zhu, J.-D. Wu, C.-M. Chu, C.-F. Yao and K.-S. Shia, Chem. Commun., 2007, 301 RSC;
(c) E. Choi, C. Lee, Y. Na and S. Chang, Org. Lett., 2002, 4, 2369 CrossRef CAS PubMed;
(d) K. Yamaguchi, H. Fujiwara, Y. Ogasawara, M. Kotani and N. Mizuno, Angew. Chem., Int. Ed., 2007, 46, 3922 CrossRef CAS PubMed;
(e) T. Oishi, K. Yamaguchi and N. Mizuno, Angew. Chem., Int. Ed., 2009, 48, 6286 CrossRef CAS PubMed.
-
(a) X. Chen, X.-S. Hao, C. E. Goodhue and J.-Q. Yu, J. Am. Chem. Soc., 2006, 128, 6790 CrossRef CAS PubMed;
(b) W. Zhou, L. Zhang and N. Jiao, Angew. Chem., Int. Ed., 2009, 48, 709 Search PubMed;
(c) W. Zhou, J. Xu, L. Zhang and N. Jiao, Org. Lett., 2010, 12, 2888 CrossRef CAS PubMed;
(d) C. Qin and N. Jiao, J. Am. Chem. Soc., 2010, 132, 15893 CrossRef CAS PubMed;
(e) S. Kamijo, T. Hoshikawa and M. Inoue, Org. Lett., 2011, 13, 5928 CrossRef CAS PubMed;
(f) S. Zheng, C. Yu and Z. Shen, Org. Lett., 2012, 14, 3644 CrossRef CAS PubMed;
(g) S. Xu, X. Huang, X. Hong and B. Xu, Org. Lett., 2012, 14, 4614 CrossRef CAS PubMed;
(h) J. Jin, Q. Wen, P. Lu and Y. Wang, Chem. Commun., 2012, 48, 9933 RSC;
(i) R.-J. Song, J. C. Wu, Y. Liu, G.-B. Deng, C.-Y. Wu, W.-T. Wei and J.-H. Li, Synlett, 2012, 2491 CAS;
(j) H. Xu, P.-T. Liu, Y.-H. Li and F.-S. Han, Org. Lett., 2013, 15, 3354 CrossRef CAS PubMed;
(k) C. Pan, H. Jin, P. Xu, X. Liu, Y. Cheng and C. Zhu, J. Org. Chem., 2013, 78, 9494 CrossRef CAS PubMed;
(l) T.-J. Gong, B. Xiao, W.-M. Cheng, W. Su, J. Xu, Z.-J. Liu, L. Liu and Y. Fu, J. Am. Chem. Soc., 2013, 135, 10630 CrossRef CAS PubMed;
(m) M. Chaitanya, D. Yadagiri and P. Anbarasan, Org. Lett., 2013, 15, 4960 CrossRef CAS PubMed;
(n) X. Kou, M. Zhao, X. Qiao, Y. Zhu, X. Tong and Z. Shen, Chem. – Eur. J., 2013, 19, 16880 CrossRef CAS PubMed;
(o) X. Hong, H. Wang, G. Qian, Q. Tan and B. Xu, J. Org. Chem., 2014, 79, 3228 CrossRef CAS PubMed;
(p) Y. Yang and S. L. Buchwald, Angew. Chem., Int. Ed., 2014, 53, 8677 CrossRef CAS PubMed.
- For review, see:
(a) G. P. Ellis and T. M. Romney-Alexander, Chem. Rev., 1987, 87, 779 CrossRef CAS; For some recent examples, see:
(b) C. Yang and J. M. Williams, Org. Lett., 2004, 6, 2837 CrossRef CAS PubMed;
(c) T. Schareina, A. Zapf and M. Beller, Chem. Commun., 2004, 1388 RSC;
(d) H.-J. Cristau, A. Ouali, J.-F. Spindler and M. Taillefer, Chem. – Eur. J., 2005, 11, 2483 CrossRef CAS PubMed;
(e) A. Littke, M. Soumeillant, R. F. Kaltenbach III, R. J. Cherney, C. M. Tarby and S. Kiau, Org. Lett., 2007, 9, 1711 CrossRef CAS PubMed;
(f) S.-I. Murahashi, T. Nakae, H. Terai and N. Komiya, J. Am. Chem. Soc., 2008, 130, 11005 CrossRef CAS PubMed;
(g) S. Velmathi and N. E. Leadbeater, Tetrahedron Lett., 2008, 49, 4693 CrossRef CAS PubMed;
(h) G. Chen, Z. Wang, J. Wu and K. Ding, Org. Lett., 2008, 10, 4573 CrossRef CAS PubMed;
(i) F. G. Buono, R. Chidambaram, R. H. Mueller and R. E. Waltermire, Org. Lett., 2008, 10, 5325 CrossRef CAS PubMed;
(j) Y. Ren, W. Wang, S. Zhao, X. Tian, J. Wang, W. Yin and L. Cheng, Tetrahedron Lett., 2009, 50, 4595 CrossRef CAS PubMed;
(k) S. Arai, T. Sato and A. Nishida, Adv. Synth. Catal., 2009, 351, 1897 CrossRef CAS;
(l) W. Han and A. R. Ofial, Chem. Commun., 2009, 5024 RSC;
(m) Y. Ju, F. Liu and C. Li, Org. Lett., 2009, 11, 3582 CrossRef CAS PubMed;
(n) X. Jia, D. Yang, S. Zhang and J. Cheng, Org. Lett., 2009, 11, 4716 CrossRef CAS PubMed;
(o) C. DeBlase and N. E. Leadbeater, Tetrahedron, 2010, 66, 1098 CrossRef CAS PubMed;
(p) S. Arai, Y. Koike and A. Nishida, Adv. Synth. Catal., 2010, 352, 893 CrossRef CAS;
(q) G. Yan, C. Kuang, Y. Zhang and J. Wang, Org. Lett., 2010, 12, 1052 CrossRef CAS PubMed;
(r) H.-Q. Do and O. Daugulis, Org. Lett., 2010, 12, 2517 CrossRef CAS PubMed;
(s) B. V. S. Reddy, Z. Begum, Y. J. Reddy and J. S. Yadav, Tetrahedron Lett., 2010, 51, 3334 CrossRef CAS PubMed;
(t) Z. Zhang, Z. Wang, R. Zhang and K. Ding, Angew. Chem., Int. Ed., 2010, 49, 6746 CrossRef CAS PubMed;
(u) P. Y. Yeung, C. M. So, C. P. Lau and F. Y. Kwong, Angew. Chem., Int. Ed., 2010, 49, 8918 CrossRef CAS PubMed;
(v) C. W. Liskey, X. Liao and J. F. Hartwig, J. Am. Chem. Soc., 2010, 132, 11389 CrossRef CAS PubMed;
(w) M. Shevlin, Tetrahedron Lett., 2010, 51, 4833 CrossRef CAS PubMed;
(x) Z. Zhao and Z. Li, Eur. J. Org. Chem., 2010, 5460 CrossRef CAS;
(y) G.-Y. Zhang, J.-T. Yu, M.-L. Hu and J. Cheng, J. Org. Chem., 2013, 78, 2710 CrossRef CAS PubMed.
-
(a) J. Kim and S. Chang, J. Am. Chem. Soc., 2010, 132, 10272 CrossRef CAS PubMed;
(b) J. Kim, H. Kim and S. Chang, Org. Lett., 2012, 14, 3924 CrossRef CAS PubMed;
(c) J. Kim, J. Choi, K. Shin and S. Chang, J. Am. Chem. Soc., 2012, 134, 2528 CrossRef CAS PubMed;
(d) Z. Wang and S. Chang, Org. Lett., 2013, 15, 1990 CrossRef CAS PubMed;
(e) A. B. Pawar and S. Chang, Chem. Commun., 2014, 50, 448 RSC; For reviews, see:
(f) J. Kim, H. J. Kim and S. Chang, Eur. J. Org. Chem., 2013, 3201 CrossRef CAS;
(g) J. Kim, H. J. Kim and S. Chang, Angew. Chem., Int. Ed., 2012, 51, 11948 CrossRef CAS PubMed.
-
(a) G. Zhang, X. Ren, J. Chen, M. Hu and J. Cheng, Org. Lett., 2011, 13, 5004 CrossRef CAS PubMed;
(b) X. Ren, J. Chen, F. Chen and J. Cheng, Chem. Commun., 2011, 47, 6725 RSC.
- S. Ding and N. Jiao, J. Am. Chem. Soc., 2011, 133, 12374 CrossRef CAS PubMed.
- For some selected pyridine-group directed C–H bond functionalization reactions, see:
(a) K. L. Hull, E. L. Lanni and M. S. Sanford, J. Am. Chem. Soc., 2006, 128, 14047 CrossRef CAS PubMed;
(b) H. Li, Y. Li, X.-S. Zhang, K. Chen, X. Wang and Z.-J. Shi, J. Am. Chem. Soc., 2011, 133, 15244 CrossRef CAS PubMed;
(c) K. Morimoto, K. Hirano, T. Satoh and M. Miura, Org. Lett., 2010, 12, 2068 CrossRef CAS PubMed;
(d) Z.-H. Guan, Z.-H. Ren, S. M. Spinella, S. Yu, Y.-M. Liang and X. Zhang, J. Am. Chem. Soc., 2009, 131, 729 CrossRef CAS PubMed;
(e) T. Vogler and A. Studer, Org. Lett., 2008, 10, 129 CrossRef CAS PubMed;
(f) G. Deng, L. Zhao and C.-J. Li, Angew. Chem., Int. Ed., 2008, 47, 6278 CrossRef CAS PubMed;
(g) X. Jia, S. Zhang, W. Wang, F. Luo and J. Cheng, Org. Lett., 2009, 11, 3120 CrossRef CAS PubMed;
(h) N. Yoshikai, S. Asako, T. Yamakawa, L. Ilies and E. Nakamura, Chem. – Asian J., 2011, 6, 3059 CrossRef CAS PubMed;
(i) L. Yang, B. Qian and H. Huang, Chem. – Eur. J., 2012, 18, 9511 CrossRef CAS PubMed;
(j) A. S. Tsai, M. E. Tauchert, R. G. Bergman and J. A. Ellman, J. Am. Chem. Soc., 2011, 133, 1248 CrossRef CAS PubMed;
(k) W. Li, P. B. Arockiam, C. Fischmeister, C. Bruneau and P. H. Dixneuf, Green Chem., 2011, 13, 2315 RSC;
(l) Y.-G. Lim, Y. H. Kim and J.-B. Kang, J. Chem. Soc., Chem. Commun., 1994, 2267 RSC;
(m) K. Gao and N. Yoshikai, J. Am. Chem. Soc., 2011, 133, 400 CrossRef CAS PubMed;
(n) J. Y. Kim, S. H. Park, J. Ryu, S. H. Cho, S. H. Kim and S. Chang, J. Am. Chem. Soc., 2012, 134, 9110 CrossRef CAS PubMed;
(o) Q. Chen, L. Ilies, N. Yoshikai and E. Nakamura, Org. Lett., 2011, 13, 3232 CrossRef CAS PubMed;
(p) X. Peng, Y. Zhu, T. A. Ramirez, B. Zhao and Y. Shi, Org. Lett., 2011, 13, 5244 CrossRef CAS PubMed;
(q) L. Zhang, Z. Liu, H. Li, G. Fang, B.-D. Barry, T. A. Belay, X. Bi and Q. Liu, Org. Lett., 2011, 13, 6536 CrossRef CAS PubMed;
(r) C.-J. Li, Acc. Chem. Res., 2009, 42, 335 CrossRef CAS PubMed.
-
(a) L. Huang, K. Cheng, B. Yao, Y. Xie and Y. Zhang, J. Org. Chem., 2011, 76, 5732 CrossRef CAS PubMed;
(b) Y. Yuan and H. Zhu, Eur. J. Org. Chem., 2012, 329 CrossRef CAS;
(c) A. Stergiou, A. Bariotaki, D. Kalaitzakis and I. Smonou, J. Org. Chem., 2013, 78, 7268 CrossRef CAS PubMed;
(d) N. Tada, M. Shomura, H. Nakayama, T. Miura and A. Itoh, Synlett, 2010, 1979 CAS;
(e) C. Zhang, X. Wang and N. Jiao, Synlett, 2014, 1458 CAS.
Footnote |
† Electronic supplementary information (ESI) available. See DOI: 10.1039/c4qo00205a |
|
This journal is © the Partner Organisations 2014 |
Click here to see how this site uses Cookies. View our privacy policy here.