DOI:
10.1039/C3RA43976C
(Paper)
RSC Adv., 2014,
4, 4782-4790
Halide ion induced tuning and self-organization of gold nanostars†
Received
31st July 2013
, Accepted 6th November 2013
First published on 7th November 2013
Abstract
The simple addition of different halide ions to the precursor polymer–solvent solution for the synthesis of gold nanostars drastically changes their optical properties owing to the blue/red shifting of the longitudinal surface plasmon resonance (LSPR) bands as well as the emergence of new plasmon bands, aesthetically illustrating the simultaneous size/shape tuning enhanced with intriguing self organization, as confirmed from the TEM measurements. Such a synchronized morphology tuning has been very well understood in terms of the distinct reaction kinetics imparted by the different adsorption affinities of inorganic halide ions towards metal ions/surfaces. The systematic results of which are discussed rigorously in the present work in conjunction with the uncommon/unusual assemblies of anisotropic/elongated gold nanostructures, the first of its kind to the best of our knowledge, that are envisioned as ‘hot spots’ for exotic applications in surface enhanced Raman scattering (SERS) as well as plasmonic waveguiding.
Introduction
Noble metal nanoparticles attract much attention in the scientific community because unlike bulk metals, their physico-chemical properties can be tuned extensively with respect to size/shape.1–4 The research activity in this field has dramatically increased over the last few years following the demonstration of exotic optical properties displayed by size/shape controlled anisotropic metal nanostructures such as nanostars, nanoflowers and nanodendrites along with a remarkable amount of literature dedicated to the resonant behavior of these metal nanoparticles.4–13 In such a nano-regime, the inherent localized surface plasmon resonances (LSPRs) due to the confinement of conduction electrons in a much smaller particle volume gives rise to many chemical, bio-sensing and photonic applications.14–20 For example, LSPRs enhance the electromagnetic fields around the surface of the nanoparticles, essentially leading to surface enhanced Raman spectroscopy (SERS), an extremely novel sensitive/selective technique for detecting minute quantities of the adsorbed surface species.10,20–24 Most of the signal enhancement in SERS compared to conventional Raman is attributed to the large increase in the local electric field generated. It has been well studied that anisotropic nanoparticles are better candidate for SERS.20–22 Furthermore, the fine tuning of the plasmon energy and line width through particle size and shape is critical to maximize the SERS signal.25
The assembly of anisotropic metal nanoparticles offers a further control of their optical properties by significantly aligning the orientation of the nanoparticles in a desired/designed manner, the co-operative SPR excitations of which facilitate a high localization of electromagnetic energy suitable for potential applications in sensing and waveguiding for functional photonic devices.26–32 The corresponding organized self assemblies that result from the near field optical plasmonic coupling among neighboring individual particles has made it possible to expand the exotic application potentials of these nanoparticles.26–36 Ordered arrays of metal nanostructures also display significant electric field enhancement and consequently an improved sensitivity is observed in both LSPR and surface enhanced Raman scattering (SERS) based assays.29–33,37
Many efforts have recently been devoted to the wet-chemical synthesis of anisotropically branched metal nanoparticles through both seed mediated as well as seedless growth procedures.4,38–40 Gold nanostars8–10,13 have been of great interest in recent times for their excellent applications in nanoantennas as well as zeptomol-sensitive SERS based sensing,10,41,42 as these 3D complex multi-branched nanoparticles with sharp tips display extremely interesting plasmonic properties but require extensive optimization. The intrinsic properties of Au nanostars result from hybridization of plasmons focalized at the core and the tips of the nanoparticles. The core acts as an antenna producing electromagnetic field enhancements of the tip plasmons.8
These monodisperse gold nanostars are produced with(out) seed mediation by different research groups worldwide utilizing dimethylformamide and poly(vinylpyrroliodone) (PVP).8,40 In the seed mediated approach, the optical properties of gold nanostars can only be extended up to 800 nm8,43while in the single step seedless process it can be routinely tuned to 920 nm, as reported previously.40 Gaining insights into the respective growth mechanisms can help us to determine the optimal synthesis conditions for obtaining and designing more complex architectures of nanoparticles with tunable plasmonic properties.
In our present case, it has been observed that the introduction of halide ions (chlorine/bromine) into the DMF–PVP complex leads to the tuning/self assembly of these gold nanostars, whereas a drastically different morphology evolves when an iodide ion is added. Till now, halide ions were utilized only for the careful etching of the surface of preformed Au nanoparticles in order to get different morphology related optical responses.44–46 Only very few reports investigate the essential role of halide ions in the actual synthesis of anisotropic gold nanostructures. Mirkin's group47a has discussed the role of halide ions in the seed mediated synthesis of gold nanoparticles using CTA–X (X = halide), where CTA–Au-X complexes play an important role in dictating the shape evolution of nanoparticles. A recent review by Catherine J. Murphy47b discussed that halide ion containing surfactants (CTAB/CTAC) are well employed for the anisotropic growth of metal nanocrystals. They demonstrate that halide ions diligently adjust the reduction potential of gold ion species, passivate the gold nanoparticle surface and control the extent of silver under-potential deposition (if present in the system), that dictates the reaction kinetics (knowing the exact parameter is lacking in the reports), leading to the growth of different faceted nanostructures. None of the previous reports explicitly studied/mentioned the effect of halide ions in the absence of surfactants for the nucleation/growth of anisotropic metallic nanostructures. Herein, we present a systematic investigation on the influence of different halide ions (in situ) on the size, morphology and monodispersity of anisotropic/elongated Au nanostructures obtained in concentrated solutions of poly(vinylpyrrolidone) in N,N-dimethylformamide for the first time to best of our knowledge. The present work elucidates the fact that the introduction of halide ions to the DMF–PVP system leads to not only the selective tuning governed by the sequential change in the morphology, but also induces the tip to tip organization of these anisotropic gold nanostructures depending upon the strength of the halide ion interaction. Precise kinetic control represents a powerful route for the facile nucleation and controlled nanocrystal growth at the early stages of the colloidal synthesis procedures, essentially delineating better ways for not only producing near monodisperse anisotropic metal nanostructures, but also exemplifying their random/self organization. The emphasis on the various synthetic parameters responsible for the same forms the major theme of our present work, clearly underlining the fact that simple yet unconventional ideas are vital for the development and design of new types of nanostructures with sophisticated application potentials.
Materials and methods
In a typical synthesis a freshly prepared solution of 10 mM PVP (Mw = 10
000, Aldrich) in DMF (Merck) is homogeneously mixed with an adequate amount of different halide ions (NaCl, NaBr, HBr, KI, CTAB, Br2) and after that tetracholoroauric acid (HAuCl4·3H2O, Aldrich) is added to the solution so as to make the molar ratio of PVP to metal (calculated in terms of polymer repeating unit or monomer chain length) ∼3333 and the whole solution is continuously stirred under normal room temperature conditions. The color of the solution starts changing from pale yellow to different colors, indicating the formation of gold nanostructures. Different halide ions at different concentrations are added in aliquots to the DMF–PVP complex and the rest of the reaction conditions are the same as mentioned above.
Optical absorption measurements were carried out in all our as-prepared nanoparticle solution samples in the wavelength range of 200–1100 nm using a Thermo Scientific absorption spectrophotometer. TEM samples were prepared by drying the fivefold centrifuged samples in ethanol at around 4500 rpm (to remove excess PVP) on carbon formvar coated copper grids and the images were acquired using an FE-Technai G2 system operated at an accelerating voltage of 300 kV. The hydrodynamic diameters of the as-prepared Au nanostructures were characterized using a particle sizing system NICOMP 380 ZLS (PSS, Santa Barbara, CA) at a measurement angle of 90º (Internal He-Ne laser, wavelength 633 nm) utilizing dynamic light scattering (DLS). All the measurements were done at room temperature unless otherwise specified.
Results and discussion
Two distinct surface plasmon bands appear in gold nanostars, a transverse plasmon band at lower wavelength (higher energy) arising due to the core, while the longitudinal band at higher wavelength (lower energy) occurs due to the surface tips/edges.8
Interestingly, the TEM images of the as-prepared, exceedingly reproducible and extremely stable gold nanostars reveal a complex multilayered core with a number of (non)planar single crystalline spikes radially branching out from the centre, resembling prominently a flower like structure, the size/shape of which has been normalized by the controlled addition of HCl without any external seed mediation. The evaluation of a plausible kinetics and growth mechanism has already been described extensively in our previous work.40 Higher volume/concentration of HCl essentially lead to a step-by-step dissociation of tips/edges from the star like Au nanostructures, gradually saturating towards the formation of spheroidal gold nanoparticles, strongly suggesting that an excess of HCl dramatically modifies the kinetics of the reaction steering towards a more conventional thermodynamic regime.
Convinced by the fact that the introduction of foreign agents in the precursor reaction medium substantially affects the size/shape of gold nanostars, we have carefully analyzed the effective role of each of the individual inorganic halide (chloride, bromide and iodide) ions upon addition to the DMF–PVP system in the following sections.
Effect of chloride ions
We have observed significant red shifting in the LSPR peaks with the sequential addition of mM quantities of NaCl to the precursor DMF–PVP system. The red shift in the LSPR peak increases drastically with an increasing NaCl concentration, finally stabilizing around 1000 nm, as shown in Fig. 1. A Gaussian multi-peak fitting (shown by dashed lines in Fig. 2) of the individual optical absorption spectra for different NaCl concentrations clearly reveals the broadening of the longitudinal plasmon peak (related to the tips) as well as the flattening of the transverse plasmon peak (corresponding to the central core), in tune with the corresponding TEM images (shown in Fig. 1(a–d)), illustrating visually the complex rearrangement of broadened tip/spike like structures with a continuum multi-layered core maintaining its overall structural stability through their cohesive single crystalline nature, albeit with different sizes for an increasing basicity of the medium. Whereas with an increasing acidity of the surrounding medium, i.e. for larger HCl additions in the precursor reaction mixture, the sequential dissociation of the tip/spike like structures from the complex 3D gold nanostars occurs, finally condensing towards the common space conserved spheroidal morphology, as it has already been discussed in our previous work.40
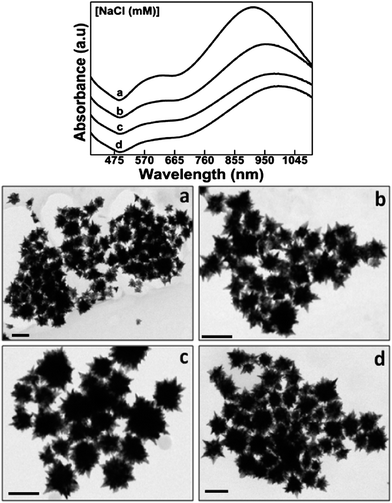 |
| Fig. 1 Additions of (a) 0.10, (b) 0.25, (c) 0.50 and (d) 1.00 mM NaCl concentrations to the precursor reaction mixture clearly influence the size distribution of the as-formed gold nanostars, as revealed by the optical spectra (a–d) and their corresponding TEM images (scale bar 200 nm). | |
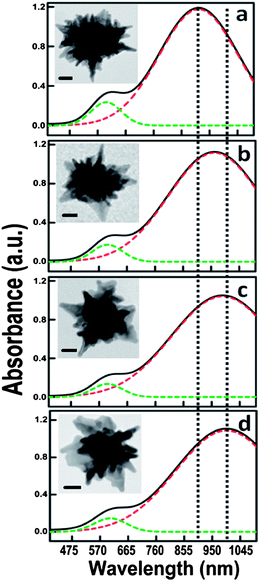 |
| Fig. 2 Multiple Gaussian peak fittings of the optical absorption spectra of (a) 0.10, (b) 0.25, (c) 0.50 and (d) 1.00 mM NaCl modified gold nanostars (the high resolution images of which are shown in the inset with a scale bar of 20 nm). Black (solid) curves are the experimental ones and dotted lines are their respective Gaussian fits. The band-width (as shown by the two vertical dotted lines) illustrates the gradual red-shifting of the longitudinal peak position from (a) to (d), analytically signifying the variation in the size/shape and arrangement of the tip/spike like structures in the as-formed gold nanostars with respect to increasing NaCl concentration. | |
Moreover, it is interesting to note that with NaCl addition, an uneven nucleation of seed particles takes place as clearly depicted by the bimodal distribution in the TEM images (see Fig. 1(a–d), average diameter 90 and 60 nm, respectively). Preferential solvation of the Na+ ions by DMF takes place and chloride ions maintain the equilibrium concentration of AuCl4− in the solution (Le Chatlier principle), thereby drastically decreasing the nucleation/growth rate. It is necessary to mention here that either with the HCl or NaCl addition the inter-particle distance between the as-formed Au nanostructures invariably decreases, signaling the fact that inter-particle coupling enabled plasmon resonances predominate in these gold nanostructures.
Effect of iodine/iodide ions
It is interesting to note that with the addition of KI to the precursor reaction mixture, large numbers of flat triangular nanoparticles were formed along with spherical nanoparticles, as clearly seen from the TEM images (Fig. 3) which are well correlated with the optical spectrum having plasmon resonances extending towards the near IR region along with the other plasmon band centered at 550 nm. The formation of flat gold nanotriangles happens due to the introduction of iodide ions, which inherently inhibits the formation of complex 3-D gold nanostars by sequentially etching the as-formed nanoparticle surface resulting in flat elongated gold nanostructures.
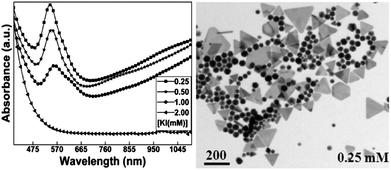 |
| Fig. 3 The effect of the addition of KI on the formation of anisotropic gold nanostructures is illustrated using optical spectra and their representative TEM image (scale bar is in nm). The increased absorbance (extended into the near IR region) strongly reveals the increased etching effect of iodide ion with increasing concentration. Note that for iodide ion concentrations higher than 1 mM no gold nanoparticles were obtained, as shown by the flat absorbance curve. | |
It is thus clear that halide ions, in particular I− ions, exert a major influence over the evolution of the shape in gold nanocrystals. Among other halide ions, iodide ions have a strong affinity to chemisorb on gold surfaces and thus have the ability to induce surface reconstruction on the adsorbed faces. Higher concentrations of iodide ions restrain the growth of Au nanoparticles as no surface plasmon resonance is observed even after several months after the addition of KI ([KI] = 2 mM) (see Fig. S1†).
Effect of bromide/bromine ions
Another surprising result was observed when ample amounts of NaBr or HBr (brominated halide ions) were added to the DMF–PVP complex, as enumerated by the formation of a new hump in the extinction spectra, indicating the development of random yet controlled assemblies of anisotropic gold nanoparticles (Fig. 4–7) depending on the strength of their electromagnetic coupling (controlled by the inter-particle spacing as well as the dielectric constant of the surrounding medium). It is found that the coupling strength decreases at higher bromine ion concentrations.
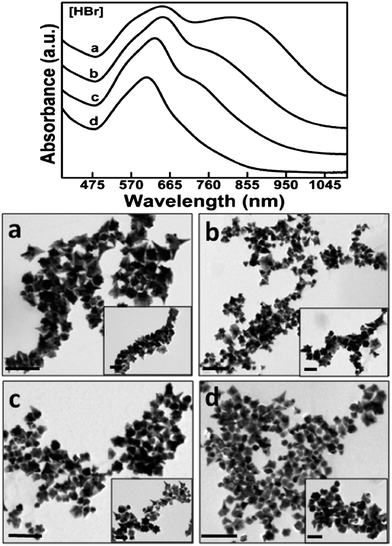 |
| Fig. 4 The increasing addition of (a) 0.20, (b) 0.40, (c) 0.60 and (d) 0.80 mM HBr to the precursor reaction mixture clearly influences the formation as well as the self-organization of anisotropic gold nanostructures, as prominently revealed by the optical spectra and their corresponding TEM images (scale bar 200 nm with inset scale bar 100 nm). | |
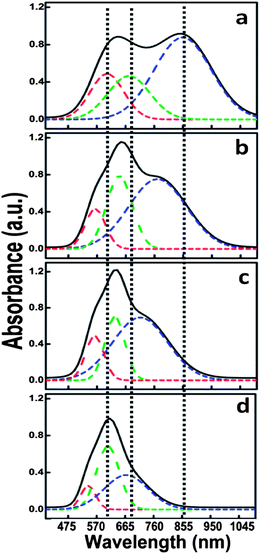 |
| Fig. 5 Multiple Gaussian peak fitted optical absorption spectra of the self-organized anisotropic gold nanostructures for (a) 0.20, (b) 0.40, (c) 0.60 and (d) 0.80 mM HBr concentrations. Black (solid) curves represent the experimental spectra and the dotted lines are their Gaussian fits. The anisotropic damping and formation of new plasmon peaks from (a) to (d) demonstrate clearly the convoluted change in morphology along with a sophisticated 2D self-organization with increasing HBr concentrations. | |
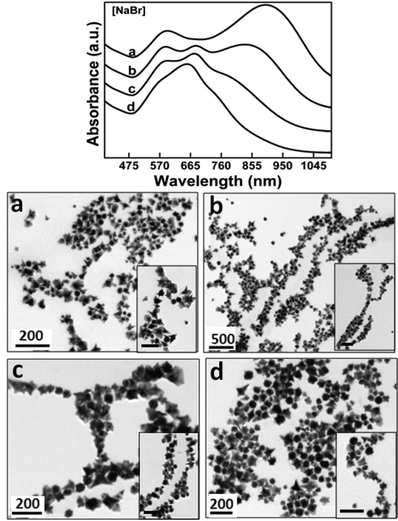 |
| Fig. 6 The increasing addition of (a) 0.20, (b) 0.40, (c) 0.60 and (d) 0.80 mM NaBr to the precursor reaction mixture clearly influences the formation as well as the self-organization of anisotropic gold nanostructures, as prominently revealed by the optical spectra and their corresponding TEM images (scale bar is in nm with inset scale bar 200 nm). | |
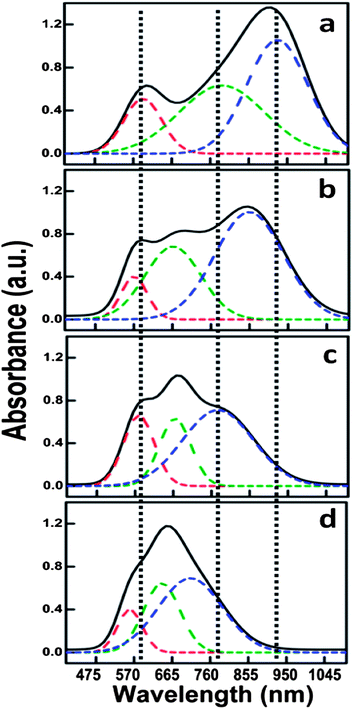 |
| Fig. 7 Multiple Gaussian peak fitted optical absorption spectra of the self-organized anisotropic gold nanostructures for (a) 0.20, (b) 0.40, (c) 0.60 and (d) 0.80 mM NaBr concentrations. Black (solid) curves represent the experimental spectra and the dotted lines are their Gaussian fits. The sequential damping and evolution of new plasmon peaks from (a) to (d) exhibit clearly the intricate change in morphology along with preferably refined 1D self-organization with increasing NaBr concentrations. | |
In terms of assimilating the extinction spectra for different HBr/NaBr concentrations, each representative spectra was deconvoluted utilizing Gaussian multi-peak fitting procedure, as shown in Fig. 5 and 7 with dashed lines. The main observation inferred is systematically explained as follows. Both the longitudinal LSPR mode (related to the tips) and transverse LSPR mode (corresponding to the central core) were blue-shifted along with a gradual decrease in the intensity of the longitudinal LSPR mode, signifying the effect of decreasing tip/spike length, their size and number, resulting in the overall change in morphology of the individual nanoparticles themselves. The anisotropic broadness of the individual plasmonic peaks essentially depicts their highly coupled nature, associated with the collective participation of individual gold nanoparticles in an ordered manner envisaged through in vitro PVP–bromine interaction in the surrounding medium.
Deconvolution of these individual asymmetrical plasmon peaks with different HBr/NaBr concentrations (shown in Fig. 5 and 7) qualitatively estimates the extent of the deviation from spherical symmetry of the as-formed gold nanostructures as well as their random self-organized configuration in an intriguing fashion is in complete collusion with the observed TEM images (Fig. 4 and 6). To our surprise, we saw that a 2D organization is preferred with HBr, while tip-to-tip or 1D assembly is preferred with NaBr addition, further accounting for the dynamic strength of the solvent DMF interaction with the cationic counterpart of the bromine ion in precisely engineering the unconventional anisotropic assembled configuration, even in the absence of other external energy resources.
The exclusive comparison of the optical absorption spectra of gold nanostructures formed with different NaBr concentrations (after 1 day and 8 months of preparation, see Fig. 8) illustrates conveniently the stability of the novel assembled gold nanostructures, which can be directly utilized for practical application purposes. The pH and refractive index stability of these as-formed assembled gold nanostructures were also conveniently demonstrated, as shown in Fig. S2 and S3,† establishing their robustness.
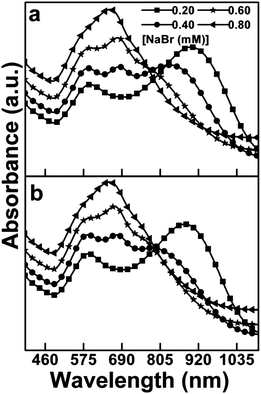 |
| Fig. 8 The inherent stability and the strength of self-organized anisotropic gold nanostructures formed in the presence of different NaBr concentrations, as clearly revealed by the reproducible absorption spectra (a) after 1 day and (b) after 8 months of formation. | |
Overall, the halide ions present in the solution form the gold–chloro–X (X = halide) complex, thereby strongly affecting its reduction potential (in the decreasing order Au–Cl > Au–Br > Au–I) and binding strength (increasing as Cl− < Br− < I−), subsequently inhibiting the nucleation as well as the growth of gold nanoparticles by way of halide ion adsorption on the metal surface. From our present experimental results, it can be easily inferred that along with the polymer–halide ion interaction, the gold chloro complex facilitates the formation of 3D anisotropic nanostructures and the gold bromo complex initiates the nanoparticle network formation, while the gold iodo complex extends the formation of planar Au nanostructures. Since self-organization predominates with the addition of bromine ions, as confirmed from the variation in hydrodynamic diameter obtained through the dynamic light scattering (DLS) measurements shown in Fig. 9, effectively matching our TEM images (Fig. 6), we can assert the simple fact that the organized nanoparticle chain length is maximum at the optimal concentration of 0.4 mM NaBr, which randomizes for lower/higher concentrations of NaBr under the present experimental conditions, thereby encouraging us to investigate the suggestive/irreversible role of bromine ions in the assembly of metal nanostructures.
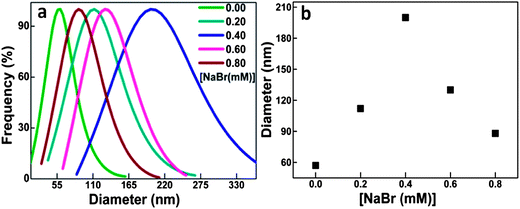 |
| Fig. 9 (a) Normalized hydrodynamic diameter of the as-formed Au nanostructures measured by dynamic light scattering (DLS). (b) The variation of the hydrodynamic diameter illustrates the coupling strength between adjacent Au nanoparticles resulting in stronger/longer chain length (the maximum of which appears for an optimal concentration of 0.4 mM NaBr), in direct confirmation with our TEM images given in Fig. 6. | |
Thus, control experiments were carried out by separately adding the well-known surfactant cetyltrimethylammonium bromide (CTAB), known for its long cationic chain suitably terminated with bromine ions, and molecular Br2 to the DMF–PVP complex, so as to test the individual roles of cations and halogen ions (as shown in Fig. S4 and S5†). As expected, assembled gold nanostructures obtained in both the cases emphasize the necessity of bromine ions in promoting the coupled organization of nanoparticles during the nucleation/growth stage itself. Instead, when we use bromine containing K2AuBr4 as the metal precursor we observe the persistence of the assembled gold nanostructures in a simple but efficient manner, as shown in Fig. S6† (more detailed work on the same is in progress), validating the certain formation of PVP–Au–bromo complexes (as schematically proposed in Scheme 1), which in conjunction with the DMF–PVP complex accentuates the organized chain-like formation of metal nanostructures. The effect of different molecular weights of PVP and gold concentrations on the optical spectra as well as on the assembly in the presence of NaBr is shown in Fig. S7 and S8.†
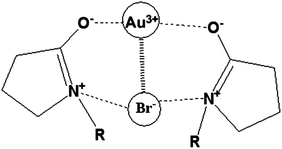 |
| Scheme 1 showing the plausible PVP–Au-bromo complex interaction, leading to the formation of organized nanostructured networks. | |
Till now, most of the available literature reports only the effect of halide ions on the post synthesized metal nanostructures. Recently, Liz-Marzán and his group48 have shown that by adding a small amount of CTAB to the gold nanostars colloidal solution reshaping takes place easily, as seen by the gradual conversion of 3D nanostar morphologies into quasi-spherical gold nanoparticles, which has been ascribed to the presence of both Br and the integrated chain length of CTAB, forcibly implying the idea that reshaping is not feasible in the presence of small inorganic molecules such as NaBr. In terms of verifying this nascent concept, we have added small amount of NaBr to the preformed colloidal Au nanostars and found that reshaping does indeed takes place (see Fig. S9†), but more slowly compared with that of CTAB, which prompted us to quantify the in situ effect of bromine entities in the growth/formation of metal nanostructures, in particular. Therefore, we examined the individual effects of three different bromine entities, namely NaBr, CTAB and Br2, in our synthesis protocol and their sharp contrasted results lead us to conclude that when brominated ions are used in situ, the self-organization (sharp tip-to-tip alignment) of metal nanostructures dominates (which correlates very well with our proposed Scheme 1 as well), and when used on the preformed nanoparticles aggressive reshaping takes place depending on their reactive strength. Further work of which is fast in progress and will be published elsewhere.
Furthermore, controlled inter-particle spacing in self-assembled metal nanostructures is achieved by encapsulating within organic shells of varying thicknesses, or by using stimuli-responsive polymer ligands, or by varying the type and location of ligands on the surface of the nanoparticles.49–51 This is also well correlated with the appearance of a new hump like feature in the near infra-red region (700–1100 nm) of the extinction spectra along with the existing transverse and longitudinal SPR peaks, indicating strong near-field plasmon coupling among the assembled anisotropic metal nanoparticles, that collectively behave as a continuous elongated quasi-1D nanostructure supporting multi-polar mode resonances in analogy with longer metal nanowire structures. Importantly, the perseverance of these spectral features emphasize the robustness of the plasmon coupling and its ability to tolerate defects within the sub-nm spaced assembly of nanoparticles, thereby providing a unique strategy for promoting a complex sub-wavelength optical phenomenon using bottom up design strategies.27,28,31,52
In our present work, we firmly believe that the surface chemisorbed PVP molecules not only dictate the individual size/shape of the as-formed anisotropic gold nanostructures but also effectively co-ordinate their in situ as well as intuitive programmed/directed organization in the presence of sufficient amount of NaBr/HBr, the mechanistic/topological aspects of which had not been verified or had been overlooked till now. It is further proposed that our as-prepared self-organized metal nanostructures can be directly utilized as plasmonic waveguides, particularly in view of the fact that the adjacent gold nanoparticles were mostly attached through their sharp tips, allowing them to transport electromagnetic energy over reasonably long distances far below the diffraction limit and hence minimizing the propagation loss to a large extent,28 highlighting the significance of our present bottom-up approach utilizing colloidal chemical synthesis procedures.
Even though PVP has already been extensively used both as reducing agent as well as steric stabilizer in the solution-phase synthesis of colloidal metal nanoparticles,53 its ability in giving rise to the non-equilibrium coupled integrative self-assembly to produce extended and multiple length scale nanostructured networks has been carefully identified and understood for the first time to the best of our knowledge. It is worth pursuing to ensure that the colossal breaking of the entangled nature of this universally soluble homogeneous polymer PVP in the presence of halide ions, especially the bromine ions, should essentially be the major factor in delineating the spatio-temporal organization of highly anisotropic metal nanostructures into long chain or any other patterned/templated shapes with ease even under normal conditions. Further work is in progress to tune this progressive self-assembly of these anisotropic gold nanostructures in synthetic patterns, as well as to understand the nature of the complex nucleation/growth mechanism.
Conclusions
With respect to the influence of halide ions, the modified individual SPR behavior extends over the entire visible and near IR electromagnetic spectrum, providing a convenient strategy for surface ligand induced electric dipolar transitions, which are macroscopically thermodynamic in nature but highly sensitive to microscopic kinetic aspects. The addition of HCl/NaCl offers tuning in SPR while the introduction of HBr/NaBr leads to assembled nanostructures. These confinements of light energy of the individual nanoparticles facilitate an enhancement in the Raman signal, while their assembly can be employed for plasmonic waveguiding.
Acknowledgements
The author AK thanks CSIR for the NET-JRF fellowship. We sincerely thank the R&D Scheme, MTech (Nano) as well as the USIC, University of Delhi, for materials characterization. We are grateful to Mr Rahul Bhardwaj for TEM imaging.
References
- H. Zhang, M. Jin and Y. Xia, Noble-Metal Nanocrystals with Concave Surfaces: Synthesis and Applications, Angew. Chem., Int. Ed., 2012, 51, 2 CrossRef.
- H. M. Chen and R. S. Liu, Architecture of Metallic Nanostructures: Synthesis Strategy and Specific Applications, J. Phys. Chem. C, 2011, 115, 3513 CAS.
- K. S. Lee and M. A. El-Sayed, Gold and Silver Nanoparticles in Sensing and Imaging: Sensitivity of Plasmon Response to Size, Shape, And Metal Composition, J. Phys. Chem. B, 2006, 110, 19220 CrossRef CAS PubMed.
- B. Lim and Y. Xia, Metal Nanocrystals with Highly Branched Morphologies, Angew. Chem., Int. Ed., 2011, 50, 76 CrossRef CAS PubMed.
- F. Hao, C. L. Nehl, J. H. Hafner and P. Nordlander, Plasmon Resonances of a Gold Nanostar, Nano Lett., 2007, 7, 729 CrossRef CAS PubMed.
- S. K. Dondapati, T. K. Sau, C. Hrelescu, T. A. Klar, F. D. Stefani and J. Feldmann, Label-Free Biosensing Based On Single Gold Nanostars As Plasmonic Transducers, ACS Nano, 2010, 4, 6318 CrossRef CAS PubMed.
- J. L. Burt, J. L. Elechiguerra, J. Reyes-Gasga, J. M. Montejano-Carrizales and M. Jose-Yacaman, Beyond Archimedean Solids: Star Polyhedral Gold Nanocrystals, J. Cryst. Growth, 2005, 285, 681 CrossRef CAS PubMed.
- P. S. Kumar, I. P. Santos, B. R. Gonzalez, F. J. G. Abajo and L. M. Liz-Marzan, High Yield Synthesis and Optical Response Of Gold Nanostars, Nanotechnology, 2008, 19, 015606 CrossRef PubMed.
- P. R. Sajanlal and T. Pradeep, Mesoflowers: A New Class of Highly Efficient Surface-Enhanced Raman Active and Infrared-Absorbing Materials, Nano Res., 2009, 2, 306 CrossRef CAS.
- J. Xie, Q. Zhang, J. Y. Lee and D. I. C. Wang, The Synthesis Of SERS-Active Gold Nanoflower Tags For In Vivo Applications, ACS Nano, 2008, 2, 2473 CrossRef CAS PubMed.
- A. Mohanty, N. Garg and R. Jin, A Universal Approach To The Synthesis of Noble Metal Nanodendrites And Their Catalytic Properties, Angew. Chem., Int. Ed., 2010, 49, 4962 CrossRef CAS PubMed.
- J. J. Feng, A. Q. Li, Z. Lei and A. J. Wang, Low-Potential Synthesis of “Clean” Au Nanodendrites And Their High Performance Toward Ethanol Oxidation, ACS Appl. Mater. Interfaces, 2012, 4, 2570 CAS.
-
(a) A. Guerrero-Martínez, S. Barbosa, I. Pastoriza-Santos and L. M. Liz-Marzán, Nanostars Shine Bright For You. Colloidal Synthesis, Properties and Applications of Branched Metallic Nanoparticles, Curr. Opin. Colloid Interface Sci., 2011, 16, 118 CrossRef PubMed;
(b) P. Das, A. Kedia, P. S. Kumar, N. Large and T. K. Chini, Local electron beam excitation and substrate effect on the plasmonic response of single gold nanostars, Nanotechnology, 2013, 24, 405704 CrossRef PubMed.
- V. Amendola, Synthesis of Gold and Silver Nanoparticles For Photonics Application, PhD thesis, University of Padova, 2000.
- E. Hutter and J. H. Fendler, Exploitation of Localized Surface Plasmon Resonance, Adv. Mater., 2004, 16, 1685 CrossRef CAS.
- S. Y. Lee, H. C. Jeon and S. M. Yang, Unconventional Methods for Fabricating Nanostructures Toward High-Fidelity Sensors, J. Mater. Chem., 2012, 22, 5900 RSC.
- E. M. Larsson, J. Alegret, M. Kall and D. S. Sutherland, Sensing Characteristics of NIR Localized Surface Plasmon Resonances In Gold Nanorings For Application As Ultrasensitive Biosensors, Nano Lett., 2007, 7, 1256 CrossRef CAS PubMed.
- T. Chung, S. Y. Lee, E. Y. Song, H. Chun and B. Lee, Plasmonic Nanostructures For Nano-Scale Bio-Sensing, Sensors, 2011, 11, 10907 CrossRef CAS PubMed.
- E. A. Coronado, E. R. Encinaa and F. D. Stefani, Optical Properties of Metallic Nanoparticles: Manipulating Light, Heat and Forces At The Nanoscale, Nanoscale, 2011, 3, 4042 RSC.
- A. K. Willets and R. P. V. Duyne, Localized Surface Plasmon Resonance Spectroscopy and Sensing, Annu. Rev. Phys. Chem., 2007, 58, 267 CrossRef PubMed.
- B. Sharma, R. R. Frontiera, A. I. Henry, E. Ringe and R. P. V. Duyne, SERS: Materials, Applications, And The Future, Mater. Today, 2012, 15, 17 CrossRef.
- L. Rodríguez-Lorenzo, R. A. Álvarez-Puebla, F. J. G. Abajo and L. M. Liz-Marzán, Surface Enhanced Raman Scattering Using Star-Shaped Gold Colloidal Nanoparticles, J. Phys. Chem. C, 2010, 114, 7336 Search PubMed.
- E. N. Esenturk and A. R. Hight-Walker, Surface-Enhanced Raman Scattering Spectroscopy via Gold Nanostars, J. Raman Spectrosc., 2009, 40, 86 CrossRef CAS.
- L. S. Kleinman, E. Ringe, N. Valley, K. L. Wustholz, E. Phillips, K. A. Scheidt, G. C. Schatz and R. P. V. Duyne, Single-Molecule Surface-Enhanced Raman Spectroscopy Of Crystal Violet Isotopologues: Theory And Experiment, J. Am. Chem. Soc., 2011, 133, 4115 CrossRef PubMed.
- E. Ringe, M. R. Langille, K. Sohn, J. Zhang, J. Huang, C. A. Mirkin, R. P. V. Duyne and L. D. Marks, Plasmon Length: A Universal Parameter To Describe Size Effects In Gold Nanoparticles, J. Phys. Chem. Lett., 2012, 3, 1479 CrossRef CAS.
-
(a) S. A. Maier and H. A. Atwater, Plasmonics: Localization and Guiding Of Electromagnetic Energy in Metal/Dielectric Structures, J. Appl. Phys., 2005, 98, 011101 CrossRef PubMed;
(b) S. A. Maier, P. G. Kik, H. A. Atwater, S. Meltzer, E. Harel, B. E. Koel and A. A. G. Requicha, Local Detection Of Electromagnetic Energy Local Detection Of Electromagnetic Energy Transport Below The Diffraction Limit In Metal Nanoparticle Plasmon Waveguides, Nat. Mater., 2003, 2, 229 CrossRef CAS PubMed.
- H. Staleva, S. E. Skrabalak, C. R. Carey, T. Kosel, Y. Xia and G. V. Hartland Coupling, To Light, And Transport And Dissipation Of Energy In Silver Nanowires, Phys. Chem. Chem. Phys., 2009, 11, 5889 RSC.
- W. K. Jung and K. M. Byun, Fabrication of Nanoscale Plasmonic Structures and Their Applications to Photonic Devices and Biosensors, Biomedical Engineering Letters, 2011, 1, 153 CrossRef PubMed.
- B. Yan, A. Thubagere, W. R. Premasiri, L. D. Ziegler, L. D. Negro and B. M. Reinhard, Multiscale Signal Enhancement: Nanoparticle Cluster Arrays, ACS Nano, 2009, 3, 1190 CrossRef CAS PubMed.
- W. Li, P. H. C. Camargo, X. Lu and Y. Xia, Dimers Of Silver Nanospheres: Facile Synthesis And Their Use As Hot Spots For Surface-Enhanced Raman Scattering, Nano Lett., 2009, 9, 485 CrossRef CAS PubMed.
-
(a) P. K. Jain, Plasmons In Assembled Metal Nanostructures: Radiative And Nonradiative Properties, Near-Field Coupling And Its Universal Scaling Behavior, PhD thesis, Georgia Institute of Technology, 2008;
(b) F. Zaato, Processes for Forming Plasmonic Waveguides from Self-assembled Gold Nanoparticle Thin Films, PhD thesis, Virginia Polytechnic Institute and State University, 2006.
- Z. Nie, A. Petukhova and E. Kumacheva, Properties And Emerging Applications Of Self-Assembled Structures Made From Inorganic Nanoparticles, Nat. Nanotechnol., 2010, 5, 515 CrossRef PubMed.
- F. Le, D. W. Brandl, Y. A. Urzhumov, H. Wang, J. Kundu, N. J. Halas, J. Aizpurua and P. Nordlander, Metallic Nanoparticle Arrays: A Common Substrate For Both Surface-Enhanced Raman Scattering And Surface-Enhanced Infrared Absorption, ACS Nano, 2008, 2, 707 CrossRef CAS PubMed.
-
(a) S. Lin, M. Li, E. Dujardin, C. Girard and S. Mann, One-Dimensional Plasmon Coupling By Facile Self-Assembly Of Gold Nanoparticles Into Branched Chain Networks, Adv. Mater., 2005, 17, 2553 CrossRef CAS;
(b) M. Li, S. Johnson, H. Guo, E. Dujardin and S. A. Mann, Generalized Mechanism For Ligand-Induced Dipolar Assembly Of Plasmonic Gold Nanoparticle Chain Networks, Adv. Funct. Mater., 2011, 21, 851 CrossRef CAS.
-
(a) J. A. Fan, C. Wu, K. Bao, J. Bao, R. Bardhan, N. J. Halas, V. N. Manoharan, P. Nordlander, G. Shvets and F. Capasso, Self-Assembled Plasmonic Nanoparticle Clusters, Science, 2010, 328, 1135 CrossRef CAS PubMed;
(b) S. J. Barrow, A. Funston, D. E. Gomez, T. J. Davis and P. Mulvaney, Surface Plasmon Resonances in Strongly Coupled Gold Nanosphere Chains from Monomer to Hexamer, Nano Lett., 2011, 11, 4180 CrossRef CAS PubMed.
- R. Jin, Nanoparticle Clusters Light Up In SERS, Angew. Chem., Int. Ed., 2010, 49, 2826 CrossRef CAS PubMed.
-
(a) A. Tao, P. Sinsermsuksakul and P. Yang, Tunable Plasmonic Lattices of Silver Nanocrystals, Nat. Nanotechnol., 2007, 2, 435 CrossRef CAS PubMed;
(b) T. Atay, J. H. Song and A. V. Nurmikko, Strongly Interacting Plasmon Nanoparticle Pairs: From Dipole–Dipole Interaction To Conductively Coupled Regime, Nano Lett., 2004, 4, 1627 CrossRef CAS.
- H. L. Wu, C. H. Chen and M. H. Huang, Seed-Mediated Synthesis of Branched Gold Nanocrystals Derived From The Side Growth of Pentagonal Bipyramids And The Formation of Gold Nanostars, Chem. Mater., 2009, 21, 110 CrossRef CAS.
- E. Hao, R. C. Bailey, G. C. Schatz, J. T. Hupp and S. Li, Synthesis and Optical Properties of “Branched” Gold Nanocrystals, Nano Lett., 2004, 4, 327 CrossRef CAS.
- A. Kedia and P. S. Kumar, Precursor Driven Nucleation and Growth Kinetics Of Gold Nanostars, J. Phys. Chem. C, 2012, 116, 1679 CAS.
- L. Rodríguez-Lorenzo, R. A. Alvarez-Puebla, I. Pastoriza-Santos, S. Mazzucco, O. Stephan, M. Kociak, L. M. Liz-Marzan and F. J. G. Abajo, Zeptomol Detection Through Controlled Ultrasensitive Surface-Enhanced Raman Scattering, J. Am. Chem. Soc., 2009, 131, 4616 CrossRef PubMed.
- L. Rodrıguez-Lorenzo, R. A. Alvarez-Puebla, F. J. G. Abajo and L. M. Liz-Marzan, Surface Enhanced Raman Scattering Using Star-Shaped Gold Colloidal Nanoparticles, J. Phys. Chem. C, 2010, 114, 7336 Search PubMed.
- S. Barbosa, A. Agrawal, I. Pastoriza-Santos, L. Rodríguez-Lorenzo, R. A. Alvarez-Puebla, A. Kornowski, H. Weller and L. M. Liz-Marzán, Tuning Size And Sensing Properties In Colloidal Gold Nanostars, Langmuir, 2010, 26, 14943 CrossRef CAS PubMed.
- S. Singh, R. Pasricha, U. M. Bhatta, P. V. Satyam, M. Sastry and B. L. V. Prasad, Effect Of Halogen Addition To Monolayer Protected Gold Nanoparticles, J. Mater. Chem., 2007, 17, 1614 RSC.
- B. Tang, S. Xu, J. An, B. Zhao, W. Xu and J. R. Lombardi, Kinetic Effects Of Halide Ions On The Morphological Evolution Of Silver Nanoplates, Phys. Chem. Chem. Phys., 2009, 11, 10286 RSC.
- A. Rai, A. Singh, A. Ahmad and M. Sastry, Role Of Halide Ions And Temperature On The Morphology Of Biologically Synthesized Gold Nanotriangles, Langmuir, 2006, 22, 736 CrossRef CAS PubMed.
-
(a) M. R. Langille, M. L. Personick, J. Zhang and C. A. Mirkin, Defining Rules For The Shape Evolution Of Gold Nanoparticles, J. Am. Chem. Soc., 2012, 134, 14542 CrossRef CAS PubMed;
(b) S. E. Lohse, N. D. Burrows, L. Scarabelli, L. M. Liz-Marzan and C. J. Murphy, Chem. Mater., 2013 DOI:10.1021/cm402384j.
- L. Rodríguez-Lorenzo, J. M. Romo-Herrera, J. Pérez-Juste, R. A. Alvarez-Puebla and L. M. Liz-Marzán, Reshaping and LSPR Tuning of Au Nanostars in the Presence of CTAB, J. Mater. Chem., 2011, 21, 11544 RSC.
- A. Wei, Calixarene-Encapsulated Nanoparticles: Self-Assembly Into Functional Nanomaterials, Chem. Commun., 2006, 1581 RSC.
- K. K. Caswell, J. N. Wilson, U. H. F. Bunz and C. J. Murphy, Preferential
End-to-End Assembly of Gold Nanorods by Biotin-Streptavidin Connectors, J. Am. Chem. Soc., 2003, 125, 13914 CrossRef CAS PubMed.
- Y. Wang, Y. F. Li, J. Wang, Y. Sang and C. Z. Huang, End-To-End Assembly Of Gold Nanorods By Means of Oligonucleotide-Mercury(II) Molecular Recognition, Chem. Commun., 2010, 46, 1332 RSC.
- S. A. Maier, P. G. Kik and H. A. Atwater, Optical Pulse Propagation in Metal Nanoparticle Chain Waveguides, Phys. Rev. B: Condens. Matter, 2003, 67, 205402 CrossRef.
- A. Kedia and P. S. Kumar, Solvent-Adaptable Poly(Vinylpyrrolidone) Binding Induced Anisotropic Shape Control Of Gold Nanostructures, J. Phys. Chem. C, 2012, 116, 23721 CAS.
Footnote |
† Electronic supplementary information (ESI) available: UV-vis spectra and TEM images are illustrated in Fig. S1–S9. See DOI: 10.1039/c3ra43976c |
|
This journal is © The Royal Society of Chemistry 2014 |
Click here to see how this site uses Cookies. View our privacy policy here.