DOI:
10.1039/C3RA46173D
(Paper)
RSC Adv., 2014,
4, 9330-9338
Chemoenzymatic synthesis of “click” xylosides and xylobiosides from lignocellulosic biomass
Received
28th October 2013
, Accepted 23rd January 2014
First published on 23rd January 2014
Abstract
Synthesis of bio-based molecules from plant biomass with chemoenzymatic pathways represents a challenging task for the development of green chemistry. In this context, an efficient two-step chemoenzymatic sequence has been achieved for the preparation of triazole-linked xylosides and xylobiosides from biomass-derived xylans. The synthesis of propargyl xyloside and xylobioside catalysed by a xylanase in an aqueous medium was first studied and improved according to different reaction parameters. Cycloaddition reactions between the terminal alkyne moiety of these xylosides or xylobiosides and various aliphatic, aromatic or functionalized azides (“click chemistry”) afforded various triazole-linked O-xylosides and O-xylobiosides in high yields. These molecules are of interest for different biological applications.
Introduction
Lignocellulosic biomass is an abundant and low-cost source for renewable carbon. Sustainable refineries from lignocellulose represent an alternative for the petrochemical routes with the development of the production of bioethanol, biomaterials or bio-based added-value molecules.1–4 Hemicelluloses, notably xylans, are the second most important polysaccharide in the biomass after cellulose. They are mainly constituted of pentoses and especially D-xylose that corresponds to the backbone unit of xylans.5 Natural conversion of xylans is achieved by enzymatic hydrolysis with glycoside hydrolases which can cleave glycosidic bonds by acid–base catalysis. Xylanases (EC 3.2.1.8) as endo-enzymes convert xylans into oligoxylosides whereas β-xylosidases (EC 3.2.1.37), exo-enzymes, hydrolyze oligoxylosides into D-xylose units. Besides these enzymes can also catalyze the production of various xylosides through reverse hydrolysis or transglycosylation reactions of glycosyl residues to alcohols.6–8
Xylose is an unusual carbohydrate in mammalian cells, only present in the structure of proteoglycans. Proteoglycans are natural macromolecules composed of a core protein and glycosaminoglycan (GAG) side chains constituted of a repeating disaccharide of a hexosamine (GlcNac or GalNAc) and a hexuronic acid residue (GlcA or IdoA). This polysaccharide is covalently attached to the core protein through a specific tetrasaccharidic linkage, containing a β-D-xylopyranose directly bound to a serine residue.9 These GAG side chains, mainly sulfated, display many interactions with various proteins and thus play important roles in the regulation of numerous biological functions.10
Biosynthesis of GAG chains can also take place in cells via an exogenous pathway, independently of a core protein, by using simple xylosides as primers. Xylosides with hydrophobic aglycone groups have been shown to induce free GAG chains synthesis in different cellular systems by serving as acceptors for a galactosyl transferase in the first galactosylation step.11 A range of β-D-xylopyranosides have been synthesized and studied for many years and some of them have exhibited a broad spectrum of biological applications as antithrombotic agents (Odiparcil),12 antiproliferative agents13 or for skin anti-aging applications (Pro-Xylane™)14 (Fig. 1).
 |
| Fig. 1 Some representative reported xylosides with biological activities. | |
Recently, a wide library of triazole-linked N-xylosides containing a variety of hydrophobic groups, prepared by the “click chemistry” methodology from an azido xyloside, has been synthesized (Fig. 1). These N-xylosides have been shown to prime efficiently a diverse array of GAG chains and have exhibited potential anti-cancer activities15 or glycogen phosphorylase inhibition.16 Few examples of triazole-linked O-xylosides were described in the literature. These compounds have been obtained from a fully acetylated β-propargyl xyloside, synthesized in four chemical steps from D-xylose,17 and used for the synthesis of surfactants,18 water-soluble silicon-based dendrimers,19 or nucleotide analogues.20
Preparation of glycosides using an enzymatic pathway via reverse hydrolysis or transglycosylation reactions represents an interesting and promising approach due to the use of unprotected carbohydrates, the high selectivity of the anomeric linkage and the mild reaction conditions. Previously, we have reported the synthesis of aliphatic β-xylosides by transglycosylation reactions from activated xyloside donors using a β-xylosidase.21 More recently, the use of a xylanase has led to the preparation of mixtures of aliphatic xylosides and oligoxylosides from xylans or directly from destarched wheat bran.22 One recent example in the literature described glycosidase-catalyzed synthesis of propargyl β-D-glucoside or galactoside and their use as substrates for a “click chemistry” step.23 To our knowledge, no example of enzymatic synthesis of propargyl β-D-xyloside or xylobioside was previously described.
In this study, we developed a chemoenzymatic synthesis of a series of xylosides and xylobiosides featuring a triazole heterocycle using a xylanase-catalyzed synthesis of propargyl β-D-xyloside 1 and xylobioside 2 from xylans in a first step and the copper(I) catalyzed azide–alkyne cycloaddition (CuAAC) or click chemistry in a second step (Scheme 1). Both original xylosides 3 and xylobiosides 4 should exhibit potential biological activities for the priming of the biosynthesis of GAG chains, with different physico-chemical properties such as solubility according to the degree of polymerization (DP) of the xylose moiety.
 |
| Scheme 1 General strategy for the chemoenzymatic synthesis of triazole-linked O-xylosides. | |
Results and discussion
Enzymatic synthesis of propargyl xyloside and xylobioside from xylans
Transglycosylation reactions were performed with a commercial xylanase (NS 22083, Novozymes) using beechwood xylans and propargyl alcohol in water (pH = 6) at 50 °C. Propargyl xyloside and oligoxylosides were produced with DP from 1 to 6 according to mass spectra carried out on the crude reaction mixtures (Scheme 2).
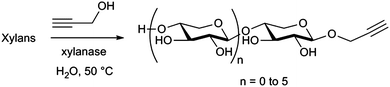 |
| Scheme 2 Enzymatic synthesis of propargyl xylosides using a xylanase. | |
In all reactions, propargyl xyloside 1 (n = 0, DP1) and propargyl xylobioside 2 (n = 1, DP2) were the most abundant compounds. Propargyl xylotrioside (n = 2, DP3) was also detected in the reaction mixture and purified in small amount but its purification was really tedious due to its polarity and to a mixture with xylose and oligoxylosides resulting from hydrolysis competing reaction. Propargyl xylosides with higher DP (DP 4 to DP 6) were produced in very small amounts and were only detected by mass spectrometry. Various experiments were focused on the different reaction parameters in order to optimize the enzymatic synthesis of propargyl xyloside and xylobioside. Enzymatic reactions were monitored by TLC (EtOAc/HOAc/H2O 7
:
2
:
2), quantification of propargyl xyloside 1 and xylobioside 2 was achieved by HPLC and both compounds were isolated by flash chromatography.
First experiments were carried out in a water solution with 10% v/v of propargyl alcohol at 50 °C and 50 IU mL−1 of xylanase with different xylan concentration (0.5 to 20% w/v, Fig. 2a and b).
 |
| Fig. 2 Influence of reaction parameters on propargyl xyloside and xylobioside synthesis. The reactions were performed at 50 °C, 1 h incubation time (a–d): 10% (v/v) of propargyl alcohol and 50 IU mL−1 of xylanase (a and b); 10% (w/v) xylans and 10% (v/v) of propargyl alcohol (c); 10% (w/v) xylans and 50 IU mL−1 of xylanase (d); 10% (v/v) of propargyl alcohol, 50 IU mL−1 of xylanase and 10% (w/v) xylans (e). | |
Results clearly show that the best yields in propargyl xylosides were reached with the lower xylan concentrations (0.5% and 1% w/v, Fig. 2a). In the above mentioned conditions, propargyl xyloside 1 and propargyl xylobioside 2 were produced in the same amounts (290 mg g−1 xylan for 0.5% xylan solution and 280 mg g−1 xylan for 1% xylan solution), with a 16% overall yield calculated on the basis of xylan exclusively constituted of D-xylose units as the starting material (6% for 1 and 10% for 2). These calculated yields are underestimated since commercially available beechwood xylan is constituted of about 60% of xylose units as determined by classical sugar analysis (see Experimental section).22a As our aim in this work was to obtain the largest amount of propargyl xylosides 1 and 2 in a single step, and not to optimize the conversion of xylans, yields were also expressed in mg of propargyl xylosides per mL of reaction (Fig. 2b). Higher xylan concentrations 10% w/v (19 mg mL−1, overall yield 11%) and 20% w/v (22 mg mL−1, overall yield 6%) led to the largest quantities of propargyl xylosides. The best compromise to maintain an acceptable yield and to insure the homogeneity of the reaction medium and an easy purification of the xylosides was to keep the xylan concentration at 10% w/v.
The effect of xylanase loading is depicted in Fig. 2c. During the reaction, the enzyme catalyzed transglycosylation (synthesis of propargyl xylosides) in competition with primary hydrolysis (hydrolysis of xylans into xylo-oligosaccharides) and secondary hydrolysis (hydrolysis of propargyl xylosides). Increasing the enzyme concentration from 2 to 100 IU mL−1 led to higher amounts of transglycosylation reaction products. A slight decrease in the yield of propargyl xylosides was observed at 100 IU mL−1 concentration, probably due to a higher secondary hydrolysis of both propargyl xyloside 1 and xylobioside 2. A more important effect on 2 was observed, leading to an increase of the ratio DP1/DP2.
The effect of concentration in propargyl alcohol in the reaction medium (1 to 20% v/v) was also studied (Fig. 2d). The best result was obtained with 20% v/v of propargyl alcohol (215 mg g−1 xylan, 12% overall yield) but a modulation in the DP1/DP2 ratio from 1
:
6 to 1
:
3 was observed compared to the reaction with 10% v/v of propargyl alcohol. The lower amount of compound 1 could be attributed to the limitation of the secondary hydrolysis of propargyl oligoxylosides leading to the formation of propargyl xyloside 1. This secondary hydrolysis cannot be quantified since the propargyl xyloside is produced both by tranglycosylation and secondary hydrolysis.
The effect of the duration of incubation was investigated (Fig. 2e) from short times (5 min) to longer reaction times (24 h). Maximal transglycosylation yields were obtained from 1 h to 4 h (180–190 mg g−1 xylan, overall yield 11%) at 50 °C. Interestingly, a modulation of the ratio 1/2 was observed according to the reaction time, from 1
:
6 (30 min) to 4.5
:
1 (24 h). In the latter case, the increase of the concentration in propargyl xyloside 1 is probably due a secondary hydrolysis of propargyl xylobioside 2. The decrease of the total amount of products for long reaction times was also observed and can be attributed to a secondary hydrolysis of propargyl xyloside 1.
Finally, an enzymatic reaction performed in the optimized reaction conditions: 10% w/v xylan concentration, 50 IU mL−1 of commercially available xylanase, 10% v/v propargyl alcohol, for 1 h led to the production of DP1 propargyl xyloside 1 (45 mg g−1 xylan) and DP2 propargyl xylobioside 2 (145 mg g−1 xylan). A putative and acceptable 18% overall yield, based on the sugar composition of the starting material (60% of D-xylose), could be attributed for the transglycosylation in these reaction conditions. A scale-up reaction (500 mL solution) was then carried out in the same reaction conditions. The amounts of compounds 1 and 2 produced were not significantly modified as determined by HPLC quantification. After centrifugation of the reaction mixture, filtration through reverse phase C18 silica gel and a final purification by flash chromatography, isolated compounds 1 (2.3 g) and 2 (7.1 g) were obtained, so about 10 g for a 500 mL reaction medium.
“Click” reactions of propargyl xylosides with azides
Preparation of 1,2,3-triazoles rings by the Huisgen's 1,3-dipolar cycloaddition24 catalyzed by Cu(I) species (CuAAC) has been applied to a wide variety of chemical research topics in the last decade25 since its introduction by Sharpless26 and Meldal.27 Introduction of 1,2,3-triazole moieties in organic molecules can bring interesting favorable physico-chemical properties for drug discovery such as a greater stability under physiological conditions, π–π interactions with aromatic rings, hydrogen-bond accepting ability, a strong dipole moment or coordination of the nitrogen atoms with metal ions.28
Click reactions were carried out in the previously described reaction conditions23 with both purified propargyl xyloside 1 and propargyl xylobioside 2 and various azides (Scheme 3).
 |
| Scheme 3 Synthesis of triazole-linked O-xylosides 3 and triazole-linked O-xylobiosides 4. | |
All the reactions afforded the corresponding triazole-linked O-xylosides 3 and triazole-linked O-xylobiosides 4 in good yields (58% to 93%, Table 1).
Table 1 Yields obtained for triazole-linked O-xylosides 3 and triazole-linked O-xylobiosides 4
Entry |
Compound |
R |
Yields (%) |
1 |
3a |
 |
75 |
4a |
93 |
|
2 |
3b |
 |
88 |
4b |
73 |
|
3 |
3c |
 |
70 |
4c |
70 |
|
4 |
3d |
 |
80 |
4d |
80 |
|
5 |
3e |
 |
87 |
4e |
74 |
|
6 |
3f |
 |
58 |
4f |
61 |
|
7 |
3g |
 |
91 |
4g |
90 |
Aliphatic (entry 1) and aromatic (entries 2–3) R groups were first introduced as substituents of the triazole ring to obtain xylosides with hydrophobic aglycone moieties 3a–c (75% to 88%) and 4a–c (70–93%), known as good candidates for an efficient priming of GAGs biosynthesis.
Introduction of functional groups such as hydroxyl group from azidoethanol (entry 4, 3d–4d) or an oligoethylene glycol (OEG) part (entry 5, 3e–4e) was achieved in order to modulate the water-solubility and the polarity of these molecules which seem to be important factors for the nature of the GAGs produced and the antiproliferative activity.13c Additionally, it is worth to note that N-xylosides with OEG linkers were recently prepared to study the effect of the length of the OEG linker on the GAG priming ability and antiproliferative activity.29 A N-protected serine-analogue was also used to give the corresponding triazole-linked xyloside 3f (58%) and xylobioside 4f (61%) attached to an aminoacid moiety (entry 6).30 Finally, click reaction of 1 and 2 with commercially available peracetylated β-D-xylopyranosyl azide afforded the original compounds 3g (91%) and 4g (90%). Similar disaccharidic compounds with a hydroxynaphtyl aromatic part were previously synthesized.31 More recently, a library of cluster xylosides with two or more xylose residues attached via triazole linkers was synthesized and some compounds showed an interesting priming of multiple GAG chains.32
Experimental section
Materials
Propargyl alcohol was purchased from Sigma-Aldrich Corp. (St. Louis, USA). Benzyl azide solution, 2-[2-(2-azidoethoxy)ethoxy]ethanol solution, Fmoc-β-azido-Ala-OH and 2,3,4-tri-O-acetyl-β-D-xylopyranosyl azide, were purchased from Sigma-Aldrich. Butyl azide,33 2-azidoethanol23 and azidobenzene34 were synthesized as previously described. Beechwood xylans were obtained from Carl Roth (Karlsruhe, Germany). The sugar composition of commercial beechwood xylan was determined after acid hydrolysis and chromatographic analysis.22a For the starting material used in our study, the total ratio in monosaccharides was determined at 65% with an overall composition of D-xylose units of about 60%. The purified thermophilic endo-xylanase was purchased from Novozymes (NS-22083). Specific activity of xylanase NS 22083 (batch VHN00002) on beechwood xylan was 38 IU mg−1.
Enzymatic synthesis of propargyl β-D-oligoxylosides
Enzymatic reactions (1 mL) were carried out in closed glass vessels with a magnetic stirring and were incubated in a thermostated oil bath for 1 h at 50 °C. The reactions were stopped by incubating the reaction mixtures during 10 min at 100 °C and centrifugating them for 10 min (2000g) in order to pellet the residual xylans. For the scale-up, the 500 mL reaction was incubated for 3 h at 50 °C in a reactor IKA®-WERKE (Eurostar).
Purification, characterization and quantification of the transglycosylation products
The qualitative analysis was performed by TLC, using Kieselgel 60 F254 aluminium-backed sheets (E. Merck) and EtOAc/HOAc/water (7
:
2
:
2) as the mobile phase. Products were detected at 130 °C using 0.2% (v/v) orcinol in H2SO4 (20%, v/v). NMR spectra were recorded on Bruker spectrometer (500 MHz for 1H and 125 MHz for 13C or 600 MHz for 1H and 150 MHz for 13C). Chemical shifts are expressed in parts per million (ppm) using TMS as an internal standard. Coupling constants J are given in Hertz (Hz) and pattern abbreviations are as follows: s singlet, d doublet, t triplet, qu quintet, sex sextuplet, m multiplet. High resolution mass spectra (HRMS) were performed on Q-TOF Micro micromass positive ESI (CV = 30 V). After centrifugation to eliminate the residual xylan, the crude reaction was concentrated to half volume under reduced pressure. The purification of transglycosylation products DP1 and DP2 was achieved by silica gel chromatography (Polygoprep® 60–50 C18, Macherey-Nagel) with H2O as the mobile phase. Fractions containing DP1 and DP2 were lyophilized and purified by silica gel chromatography (9385 Merck Kieselgel 60, 230–400 mesh, 40–63 μm) with EtOAc
:
MeOH (90
:
10) as mobile phase. These compounds were identified by 1H and 13C-NMR, COSY, 1H–13C correlation experiments and ESI-HRMS.
The quantification of DP1 and DP2 was performed by HPLC using a RP-C18 column (Kinetex 5 μm C18 100A, 150 × 4.6 mm, Phenomenex). Standards propargyl xylosides were purified as described above. Products were eluted at 0.8 mL min−1 with a mobile phase composed of a methanol
:
water mixture (3
:
97). The detection of eluates was performed with a dynamic light scattering detector (ELSD-LTII, Shimadzu).
Propargyl β-D-xylopyranoside 1. White solid, m.p. 132 °C. [α]20D −85.9 (c 0.21 in H2O). δH (500 MHz; D2O; Me4Si) 2.92 (1H, br s, H alkyne), 3.28 (1H, dd, J2,1 7.8, J2,3 9.2, 2-H), 3.33 (1H, dd, J5,4 10.5, J5,5 11.6, 5-H), 3.45 (1H, t, J3,2 = J3,4 9.2, 3-H), 3.62 (1H, ddd, J4,5 5.4, J4,3 9.2, J4,5 10.5, 4-H), 3.97 (1H, dd, J5,4 5.4, J5,5 11.6, 5-H), 4.44 (2H, br s, OCH2), 4.58 (1H, d, J1,2 7.8, 1-H); δC (125 MHz; D2O; Me4Si) 56.7 (OCH2), 65.2 (C-5), 69.1 (C-4), 72.8 (C-2), 75.6 (C-3), 76.3 (C–H alkyne), 78.8 (C-alkyne), 101.5 (C-1); ESI-HRMS: m/z calcd for C8H12O5Na: [M + Na]+: 211.0582, found: 211.0585.
Propargyl β-D-xylobioside 2. White solid, m.p. 95 °C. [α]20D −83.3 (c 0.38 in H2O). δH (500 MHz; D2O; Me4Si) 2.83 (1H, br s, H alkyne), 3.18 (1H, dd, J2′,1′ 7.9, J2′,3′ 9.2, 2′-H), 3.22–3.24 (1H, m, 5′-H), 3.24 (1H, dd, J2,1 7.7, J2,3 8.2, 2-H), 3.33 (1H, dd, J5,4 9.2, J5,5 11.8, 5-H), 3.35 (1H, dd, J3′,4′ 5.9, J3′,2′ 9.2, 3′-H), 3.50 (1H, dd, J3,2 8.2, J3,4 9.8, 3-H), 3.54–3.56 (1H, m, 4′-H), 3.71 (1H, ddd, J4,5 5.2, J4,5 9.2, J4,3 9.8, 4-H), 3.89 (1H, dd, J5′,4′ 5.5, J5′,5′ 11.6, 5′-H), 4.03 (1H, dd, J5,4 5.2, J5,5 11.8, 5-H), 4.36 (2H, br s, OCH2), 4.38 (1H, d, J1′,2′ 7.9, 1′-H), 4.53 (1H, d, J1,2 7.7, 1-H); δC (125 MHz; D2O; Me4Si) 56.6 (OCH2), 62.8 (C-5), 65.1 (C-5′), 69.0 (C-4′), 72.5 (C-2), 72.6 (C-2′), 73.5 (C-3), 75.4 (C-3′), 76.1 (C-4), 76.2 (C–H alkyne), 78.5 (C-alkyne), 101.1 (C-1), 101.7 (C-1′); ESI-HRMS: m/z calcd for C13H20O9Na: [M + Na]+: 343.1005, found: 343.0996.
Propargyl β-D-xylotrioside. Amorphous solid. [α]20D −68.6 (c 0.38 in H2O). δH (500 MHz; D2O; Me4Si) 2.91 (1H, br s, H alkyne), 3.27 (1H, dd, J2′′,1′′ 7.9, J2′′,3′′ 9.2, 2′′-H), 3.29–3.37 (3H, m, 2-H and 2′-H and 5′′-H), 3.38–3.44 (2H, m, 5′-H and 5-H), 3.45 (1H, t, J3′′,2′′ = J3′′,4′′ 9.2, 3′′-H), 3.57 (1H, t, J3′,2′ = J3′,4′ 9.2, 3′-H), 3.60 (1H, t, J3,2 = J3,4 9.2, 3-H), 3.63 (1H, ddd, J4′′,5′′ 5.5, J4′′,3′′ 9.2, J4′′,5′′ 10.1, 4′′-H), 3.77–3.82 (2H, m, 4′-H and 4-H), 3.98 (1H, dd, J5′′,4′′ 5.5, J5′′,5′′ 11.6, 5′′-H), 4.09–4.13 (2H, m, 5′-H and 5-H), 4.44–4.45 (2H, m, OCH2), 4.47 (1H, d, J1′′,2′′ 7.9, 1′′-H), 4.49 (1H, d, J1′,2′ 7.8, 1′-H), 4.61 (d, J1,2 7.7, 1-H); δC (125 MHz; D2O; Me4Si) 62.8 (C-5), 62.9 (C-5′), 65.2 (C-5′′), 69.1 (C-4′′), 72.6 (C-2 and C-2′), 72.7 (C-2′′), 79.6 (C-3 and C-3′), 75. 6 (C-3′′), 76.2 (C-4′), 76.3 (C-4), 76.8 (C–H alkyne), 78.5 (C-alkyne), 101.3 (C-1′′), 101.6 (C-1′), 101.8 (C-1); ESI-HRMS: m/z calcd for C18H28O13Na: [M + Na]+: 475.1428, found: 475.1436.
General procedure for the click reactions
Propargyl xyloside 1 or propargyl xylobioside 2 (0.5 mmol), azide (0.6 mmol), copper(II) sulfate (9 mg, 0.05 mmol), and sodium ascorbate (20 mg, 0.1 mmol) were solubilized in a mixture tert-butyl alcohol
:
water (1
:
1; 4 mL) and stirred at room temperature overnight. The solution was directly subjected to flash column chromatography with EtOAc
:
MeOH (from 90
:
10 to 50
:
50) as the mobile phase to afford the corresponding triazole-linked O-xylosides 3 or xylobiosides 4.
Xyloside 3a. Yellow oil. [α]20D −57.9 (c 0.21 in MeOH). δH (600 MHz; CD3OD; Me4Si) 0.97 (3H, t, J 7.4, triazolyl-CH2-CH2-CH2-CH3), 1.34 (2H, sex, J 7.4, triazolyl-CH2-CH2-CH2), 1.89 (2H, qu, J 7.4, triazolyl-CH2-CH2), 3.20 (1H, dd, J2,1 7.5, J2,3 9.0, 2-H), 3.22 (1H, dd, J5,4 10.3, J5,5 11.5, 5-H), 3.32–3.35 (1H, m, 3-H), 3.49 (1H, ddd, J4,5 5.4, J4,3 9.0, J4,5 10.3, 4-H), 3.89 (1H, dd, J5,4 5.4, J5,5 11.5, 5-H), 4.33 (1H, d, J1,2 7.5, 1-H), 4.41 (2H, t, J 7.4, triazolyl-CH2), 4.73 (1H, d, J 12.4, 1H OCH2), 4.91 (1H, d, J 12.4, 1H OCH2), 7.90 (1H, s, H triazolyl); δC (150 MHz; CD3OD; Me4Si) 13.7 (triazolyl-CH2-CH2-CH2-CH3), 20.6 (triazolyl-CH2-CH2-CH2), 33.3 (triazolyl-CH2-CH2), 51.1 (triazolyl-CH2), 63.0 (OCH2), 67.0 (C-5), 71.2 (C-4), 74.9 (C-2), 77.7 (C-3), 104.3 (C-1), 125.1 (C–H triazolyl), 145.6 (C-triazolyl); ESI-HMRS: m/z calcd for C12H21N3O5: [MH]+: 310.1379, found: 310.1387.
Xyloside 4a. Yellow oil. [α]20D −63.9 (c 1.55 in MeOH). δH (500 MHz; CD3OD; Me4Si) 0.92 (3H, t, J 7.5, triazolyl-CH2-CH2-CH2-CH3), 1.30 (2H, sex, J 7.5, triazolyl-CH2-CH2-CH2), 1.85 (2H, qu, J 7.5, triazolyl-CH2-CH2), 3.16–3.23 (3H, m, 2-H and 2′-H and 5′-H), 3.26–3.30 (2H, m, 5-H and 3′-H), 3.41 (1H, t, J3,2 = J3,4 8.8, 3-H), 3.47 (1H, ddd, J4′,5′ 5.4, J4′,3′ 8.8, J4′,5′ 10.3, 4′-H), 3.62 (1H, ddd, J4,5 5.3, J4,3 8.8, J4,5 10, 4-H), 3.85 (1H, dd, J5′,4′ 5.4, J5′,5′ 11.5, 5′-H), 4.00 (1H, dd, J5,4 5.3, J5,5 11.7, 5-H), 4.29 (1H, d, J1′,2′ 7.7, 1′-H), 4.30 (d, J1,2 7.4, 1-H), 4.35 (2H, t, J 7, triazolyl-CH2), 4.69 (1H, d, J 12.4, 1H OCH2), 4.87 (1H, d, J 12.4, 1H OCH2), 7.94 (s, H triazolyl); δC (125 MHz; CD3OD; Me4Si) 13.7 (triazolyl-CH2-CH2-CH2-CH3), 20.6 (triazolyl-CH2-CH2-CH2), 33.3 (triazolyl-CH2-CH2), 51.1 (triazolyl-CH2), 63.0 (OCH2), 64.6 (C-5), 67.1 (C-5′), 71.1 (C-4′), 74.3 (C-2′), 74.6 (C-2), 75.8 (C-3), 77.6 (C-3′), 78.2 (C-4), 104.0 (C-1), 104.1 (C-1′), 124.1 (C-H triazolyl), 145.6 (C-triazolyl); ESI-HMRS: m/z calcd for C17H30N3O9Na: [MH]+: 420.1982, found: 420.1979.
Xyloside 3b. Pale yellow solid, m.p. 128 °C. [α]20D −52.9 (c 0.2 in H2O). δH (500 MHz; CD3OD; Me4Si) 3.23–3.24 (1H, m, 2-H), 3.24–3.26 (1H, m, 5-H), 3.34 (1H, t, J3,2 = J3,4 8.9, 3-H), 3.52 (1H, ddd, J4,5 5.3, J4,3 8.9, J4,5 10.2, 4-H), 3.91 (1H, dd, J5,4 5.3, J5,5 11.4, 5-H), 4.40 (1H, d, J1,2 7.3, 1-H), 4.82–4.84 (1H, m, 1H OCH2), 5.00 (1H, d, J 12.5, 1H OCH2), 7.50 and 7.58 (2H, t, J 7.8, Ph), 7.83 (1H, d, J 7.8, Ph), 8.53 (1H, s, H triazolyl); δC (125 MHz; CD3OD; Me4Si) 63.0 (OCH2), 67.0 (C-5), 71.2 (C-4), 74.9 (C-2), 77.7 (C-3), 104.4 (C-1), 121.6 (C-H Ph), 123.4 (C–H triazolyl), 130.1 and 131.0 (C-H Ph), 138.4 (C-Ph), 146.7 (C-triazolyl); ESI-HRMS: m/z calcd for C14H18N3O5: [MH]+: 308.1246, found: 308.1239.
Xyloside 4b. Pale yellow solid, m.p. 168 °C. [α]20D −49.0 (c 0.5 in H2O). δH (500 MHz; CD3OD; Me4Si) 3.23–3.24 (1H, m, 2′-H), 3.24–3.26 (1H, m, 5′-H), 3.31–3.33 (1H, m, 2-H), 3.37–3.39 (2H, m, 5-H and 3-H), 3.49–3.51 (1H, m, 3′-H), 3.52–3.54 (1H, m, 4′-H), 3.68–3.70 (1H, m, 4-H), 3.92 (1H, dd, J5′,4′ 5.3, J5′,5′ 11.7, 5′-H), 4.08 (1H, dd, J5,4 5.3, J5,5 11.7, 5-H), 4.36 (1H, d, J1′,2′ 7.5, 1′-H), 4.43 (1H, d, J1,2 7.5, 1-H), 4.86 (1H, d, J 12.5, 1H OCH2), 5.02 (1H, d, J 12.5, 1H OCH2), 7.52 and 7.61 (2H, t, J 7.8, Ph), 7.86 (1H, d, J 7.8, Ph), 8.56 (1H, s, H triazolyl); δC (125 MHz; CD3OD; Me4Si) 63.0 (OCH2), 64.6 (C-5), 67.1 (C-5′), 71.1 (C-4′), 74.3 (C-2′), 74.7 (C-2), 75.8 (C-3′), 77.6 (C-3), 78.2 (C-4), 104.0 (C-1′), 104.1 (C-1), 121.6 (C-H Ph), 123.4 (C-H triazolyl), 130.1 and 131.0 (C-H Ph), 138.4 (C-Ph), 146.6 (C-triazolyl); ESI-HRMS: m/z calcd for C19H26N3O9: [MH]+: 440.1669, found: 440.1681.
Xyloside 3c. White solid, m.p. 135 °C. [α]20D −33.9 (c 0.22 in H2O). δH (500 MHz; CD3OD; Me4Si) 3.16–3.23 (1H, dd, J2,1 7.5, J2,3 9.0, 2-H), 3.18–3.26 (1H, m, 5-H), 3.31 (1H, t, J3,2 = J3,4 7.5, 3-H), 3.50 (1H, ddd, J4,5 5.3, J4,3 9.0, J4,5 10.1, 4-H), 3.88 (1H, dd, J5,4 5.3, J5,5 11.4, 5-H), 4.33 (1H, d, J1,2 7.5, 1-H), 4.73 (1H, d, J 12.4, 1H OCH2), 4.91 (1H, d, J 12.4, 1H OCH2), 5.61 (2H, s, CH2-Ph), 7.31–7.43 (5H, m, Ph), 7.99 (1H, s, H triazolyl); δC (125 MHz; CD3OD; Me4Si) 53.5 (CH2-Ph), 61.6 (OCH2), 65.6 (C-5), 69.7 (C-4), 73.4 (C-2), 76.3 (C-3), 102.9 (C-1), 123.8 (C-H triazolyl), 127.7 and 128.2 and 128.6 (C-H Ph), 135.3 (C-Ph), 146.6 (C-triazolyl); ESI-HMRS: m/z calcd for C15H19N3O5Na: [M + Na]+: 344.1222, found: 344.1227.
Xyloside 4c. White solid, decomposition at T > 225 °C. [α]20D −61.8 (c 0.18 in DMSO). δH (500 MHz; DMSO-d6; Me4Si) 2.98–3.04 (2H, m, 2-H and 2′-H), 3.06–3.09 (1H, m, 5′-H), 3.09–3.13 (1H, m, 3′-H), 3.16–3.18 (1H, m, 5-H), 3.21–3.25 (1H, m, 3-H), 3.26–3.31 (1H, m, 4′-H), 3.50 (1H, dt, J4,5 5.5, J4,3 9.4, 4-H), 3.70 (1H, dd, J5′,4′ 5.5, J5′,5′ 11.5, 5′-H), 3.88 (1H, dd, J5,4 5.5, J5,5 11.5, 5-H), 4.23 (1H, d, J1′,2′ 7.5, 1′-H), 4.26 (1H, d, J1,2 7.5, 1-H), 4.59 (1H, d, J 12.1, 1H OCH2), 4.77 (1H, d, J 12.1, 1H OCH2), 5.00 (3 OH), 5.17 (2 OH), 5.59 (2H, s, CH2-Ph), 7.30–7.39 (10H, m, Ph), 8.15 (1H, s, H triazolyl); δC (125 MHz; DMSO-d6; Me4Si) 52.8 (CH2-Ph), 61.6 (OCH2), 63.2 (C-5), 65.8 (C-5′), 69.5 (C-4′), 72.6 (C-2′), 73.1 (C-2), 74.2 (C-3), 75.4 (C-4), 76.3 (C-3′), 102.0 (C-1′), 102.7 (C-1), 124.2 (C–H triazolyl), 127.9–128.7 (C-H Ph), 136.1 (C-Ph), 144.0 (C-triazolyl); ESI-HMRS: m/z calcd for C20H27N3O9Na: [M + Na]+: 476.1645, found: 476.1653.
Xyloside 3d. Pale yellow solid, decomposition at T > 188 °C. [α]20D −47.1 (c 0.28 in H2O). δH (500 MHz; D2O; Me4Si) 3.30 (1H, dd, J2,1 7.8, J2,3 9.2, 2-H), 3.36 (1H, dd, J5,4 10.5, J5,5 11.7, 5-H), 3.43 (1H, t, J3,2 = J3,4 9.2, 3-H), 3.62 (1H, ddd, J4,5 5.5, J4,3 9.2, J4,5 10.5, 4-H), 3.97 (1H, dd, J5,4 5.5, J5,5 11.7, 5-H), 4.01 (2H, t, J 5.0, triazolyl-CH2-CH2-OH), 4.51 (1H, d, J1,2 7.8, 1-H), 4.57 (2H, t, J 5.0, triazolyl-CH2-CH2-OH), 4.87 (1H, d, J 12.6, 1H OCH2), 4.97 (1H, d, J 12.6, 1H OCH2), 8.10 (1H, s, H-triazolyl); δC (125 MHz; D2O; Me4Si) 52.5 (triazolyl-CH2-CH2-OH), 60.1 (triazolyl-CH2-CH2-OH), 62.0 (OCH2), 65.1 (C-5), 69.1 (C-4), 72.9 (C-2), 75.6 (C-3), 102.1 (C-1), 125.7 (C-H triazolyl), 143.4 (C-triazolyl); ESI-HMRS: m/z calcd for C10H17N3O6Na: [M + Na]+: 298.1015, found 298.1021.
Xyloside 4d. Yellow solid, decomposition at T > 225 °C. [α]20D −65.7 (c 0.28 in H2O). δH (500 MHz; D2O; Me4Si) 3.28 (1H, dd, J2,1 7.8, J2,3 9.1, 2-H), 3.31 (1H, dd, J2′,1′ 7.9, J2′,3′ 9.2, 2′-H), 3.32 (1H, dd, J5′,4′ 10.6, J5′,5′ 11.6, 5′-H), 3.41 (1H, dd, J5,4 10.3, J5,5 11.8, 5-H), 3.44 (1H, t, J3′,2′ = J3′,4′ 9.2, 3′-H), 3.56 (1H, t, J3,2 = J3,4 9.1, 3-H), 3.63 (1H, ddd, J4′,5′ 5.5, J4′,3′ 9.2, J4′,5′ 10.6, 4′-H), 3.78 (1H, ddd, J4,5 5.3, J4,3 9.1, J4,5 10.3, 4-H), 3.98 (1H, dd, J5′,4′ 5.5, J5′,5′ 11.6, 5′-H), 4.02 (2H, t, J 4.2, triazolyl-CH2-CH2-OH), 4.11 (1H, dd, J5,4 5.3, J5,5 11.8, 5-H), 4.48 (1H, d, J1′,2′ 7.9, 1′-H), 4.54 (1H, d, J1,2 7.8, 1-H), 4.58 (2H, t, J 5.1, triazolyl-CH2-CH2-OH), 4.88 (1H, d, J 12.7, 1H OCH2), 4.97 (1H, d, J 12.7, 1H OCH2), 8.10 (1H, s, H triazolyl); δC (125 MHz; D2O; Me4Si) 52.5 (triazolyl-CH2-CH2-OH), 60.1 (triazolyl-CH2-CH2-OH), 62.0 (OCH2), 62.9 (C-5), 65.2 (C-5′), 69.1 (C-4′), 72.7 (C-2′), 72.8 (C-2), 73.7 (C-3), 75.7 (C-3′), 76.3 (C-4), 101.8 (C-1′), 102.0 (C-1), 125.7 (C-H triazolyl), 143.4 (C-triazolyl); ESI-HMRS: m/z calcd for C15H25N3O10Na: [M + Na]+: 430.1438, found 430.1434.
Xyloside 3e. Yellow oil. [α]20D −32.1 (c 0.12 in H2O). δH (600 MHz; D2O; Me4Si) 3.27 (1H, dd, J2,1 7.8, J2,3 10, 2-H), 3.31–3.35 (1H, m, 5-H), 3.42 (1H, t, J3,2 = J3,4 10, 3-H), 3.54–3.56 (2H, m, e-CH2), 3.59–3.61 (1H, m, 4-H), 3.61–3.63 (2H, m, c-CH2), 3.62–3.64 (2H, m, d-CH2), 3.64–3.69 (2H, m, f-CH2), 3.96 (1H, t, J5,4 = J5,5 10.0, 5-H), 3.99 (2H, t, J 5, b-CH2), 4.50 (1H, d, J1,2 7.8, 1-H), 4.64 (2H, t, J 5, a-CH2), 4.85 (1H, d, J 12.5, 1H OCH2), 4.95 (1H, d, J 12.5, 1H OCH2), 8.11 (1H, s, H triazolyl); δC (150 MHz; D2O; Me4Si) 50.0 (C-a), 60.3 (C-f), 62.0 (OCH2), 65.1 (C-5), 68.7 (C-b), 69.1 (C-4), 69.3 (C-c), 69.6 (C-d), 71.8 (C-e), 72.9 (C-2), 75.6 (C-3), 102.2 (C-1), 125.7 (C-H triazolyl), 143.5 (C-triazolyl); ESI-HMRS: m/z calcd for C14H25N3O8Na: [M + Na]+: 386.1539, found: 386.1537.
Xyloside 4e. Yellow oil. [α]20D −19.1 (c 0.49 in H2O). δH (500 MHz; D2O; Me4Si) 3.27 (1H, dd, J2,1 7.8, J2,3 9.0, 2-H), 3.29–3.33 (2H, m, 2′-H and 5′-H), 3.41 (1H, dd, J5,4 10.5, J5,5 12, 5-H), 3.44 (1H, t, J3′,2′ = J3′,4′ 9.3, 3′-H), 3.54–3.64 (3H, m, 3-H and e-CH2), 3.64–3.66 (1H, m, 4′-H), 3.65–3.69 (6H, m, c-CH2 and d-CH2 and f-CH2), 3.79 (1H, ddd, J4,5 5.3, J4,3 9.0, J4,5 10.5, 4-H), 3.96–3.99 (1H, m, 5′-H), 3.99–4.01 (2H, m, b-CH2), 4.11 (1H, dd, J5,4 5.3, J5,5 12, 5-H), 4.47 (1H, d, J1,2 7.8, 1-H), 4.53 (d, J1′,2′ 7.7, 1′-H), 4.65 (2H, t, J 4.5, a-CH2), 4.87 (1H, d, J 12.5, 1H OCH2), 4.96 (1H, d, J 12.5, 1H OCH2), 8.11 (1H, s, H triazolyl); δC (125 MHz; D2O; Me4Si) 50.0 (C-a), 60.3 (C-f), 62.0 (OCH2), 62.9 (C-5), 65.2 (C-5′), 68.7 (C-b), 69.2 (C-4′), 69.3 (C-c), 69.7 (C-d), 71.6 (C-e), 72.7 (C-2′), 72.8 (C-2), 73.7 (C-3), 75.6 (C-3′), 76.3 (C-4), 101.8 (C-1′), 102.0 (C-1), 125.7 (C-H triazolyl), 143.5 (C-triazolyl); ESI-HMRS: m/z calcd for C19H33N3O12Na: [M + Na]+: 518.1962, found: 518.1968.
Xyloside 3f. (mixture of rotamers). White solid, decomposition at T > 215 °C. [α]20D −91.8 (c 0.39 in H2O). δH (500 MHz; D2O; Me4Si) 3.0 (1H, t, J2,1 = J2,3 8.5, 2-H), 3.11–3.17 (1H, m, 5-H), 3.36 (1H, t, J3,2 = J3,4 8.5, 3-H), 3.41–3.45 (1H, m, 4-H), 3.80 (1H, dd, J5,4 5.3 Hz, J5,5 11.6, 5-H), 4.08 (1H, m, NHCOOCH2CH), 4.16–4.19 (1H, m, 1H NHCOOCH2), 4.30 (1H, d, J1,2 8.5, 1-H), 4.33–4.41 (2H, m, 1H CH2 NHCOOCH2 and CH2CHCOOH), 4.54–4.60 (2H, m, 1H CH2CHCOOH and 1H OCH2), 4.69 (1H, d, J 11.9, 1H OCH2), 4.81–4.83 (1H, m, 1H CH2CHCOOH); δC (125 MHz; D2O; Me4Si) 46.8 (NHCOOCH2CH), 51.9 (CH2CHCOOH), 56.0 (CHCOOH), 61.7 (OCH2), 65.1 (C-5), 66.5 (NHCOOCH2), 69.1 (C-4), 72.8 (C-2), 75.5 (C-3), 102.2 (C-1), 120.1 (C-H triazolyl), 125.1 (CH-Ph), 125.4 (CH-Ph), 127.4 (CH-Ph), 127.9 (CH-Ph), 140.9 (CH-Ph), 143.7 (C-Ph), 143.2 (C-triazolyl), 157.0 (OCONH), 174.4 (COOH); ESI-HMRS: m/z calcd for C26H28N4O9Na: [M + Na]+: 563.1754, found: 563.1747.
Xyloside 4f. (mixture of rotamers). White solid, decomposition at T > 190 °C. [α]20D −42.1 (c 0.19 in H2O). δH (600 MHz; D2O; Me4Si) 3.01–3.02 (1H, t, J2,1 = J2,3 9.0, 2-H), 3.16–3.22 (2H, m, 2′-H and 5-H), 3.27–3.31 (1H, m, 5′-H), 3.37–3.42 (3H, m, 3-H and 3′-H and 4-H), 3.61 (1H, ddd, J4′,5′ 5.4, J4′,3′ 9.5, J4′,5′ 10.5, 4′-H), 3.80 (1H, dd, J5,4 5.0, J5,5 12.6, 5-H), 3.96 (dd, J5′,4′ 5.4, J5′,5′ 11.3, 5′-H), 4.11–4.14 (2H, m, NHCOOCH2CH and 1H NHCOOCH2), 4.27 (1H, d, J1′,2′ 7.8, 1′-H), 4.29 (1H, d, J1,2 8.0, 1-H), 4.45–4.47 (1H, m, CHCOOH), 4.49–4.51 (1H, m, 1H NHCOOCH2), 4.55–4.58 (2H, m, 1H CH2CHCOOH and 1H OCH2), 4.67 (1H, d, J 12.4, 1H OCH2), 4.84–4.87 (1H, m, 1H CH2CHCOOH); δC (150 MHz; D2O; Me4Si) 46.8 (NHCOOCH2CH), 51.9 (CH2CHCOOH), 56,0 (CHCOOH), 61.8 (OCH2), 62.7 (C-5), 65.1 (C-5′), 66.5 (NHCOOCH2), 69.1 (C-4′), 72.5 (C-2), 72.7 (C-2′), 73.4 (C-3), 75.5 (C-3′), 76.1 (C-4), 101.7 (C-1′), 102.0 (C-1), 120.1 (C-H triazolyl), 125.1 (CH-Ph), 125.2 (CH-Ph), 125.4 (CH-Ph), 125.5 (CH-Ph), 125.4 (CH-Ph), 127.4 (CH-Ph), 127.5 (CH-Ph), 128.0 (CH-Ph), 128.1 (CH-Ph), 140.9 (C-triazolyl), 143.2 (C-Ph), 143.6 (C-Ph), 143.9 (C-Ph), 157.1 OCONH, 174.4 (COOH); ESI-HMRS: m/z calcd for C31H36N4O13Na: [M + Na]+: 695.2177 found: 695.2183.
Xyloside 3g. Pale yellow solid, decomposition at T > 180 °C. [α]20D −76.5 (c 0.40 in DMSO). δH (500 MHz; DMSO; Me4Si) 1.77 (3H, s, COCH3), 1.96 (3H, s, COCH3), 1.98 (3H, s, COCH3), 2.97 (1H, dd, J2,1 7.6, J2,3 9.0, 2-H), 3.07–3.08 (1H, m, 5-H), 3.10 (1H, t, J3,2 = J3,4 9.0, 3-H), 3.28 (1H, ddd, J4,5 5.4, J4,3 9.0, J4,5 10.1, 4-H), 3.71 (1H, dd, J5,4 5.4, J5,5 11.4, 5-H), 3.77 (1H, t, J5a,4a = J5a,5a 10.0, 5a-H), 4.08 (1H, dd, J5a,4a 5.6, J5a,5a 10.0, 5a-H), 4.13 (1H, d, J1,2 7.6, 1-H), 4.61 (1H, d, J 12.6, 1H OCH2), 4.74 (1H, d, J 12.6, 1H OCH2), 5.09 (1H, td, J4a,3a = J4a,5a 10.0, J4a,5a 5.6, 4a-H), 5.42 (1H, t, J3a,2a = J3a,4a 10.0, 3a-H), 5.55 (1H, t, J2a,1a = J2a,3a 10.0, 2a-H), 6.13 (d, J1a,2a 10.0, 1a-H), 8.32 (1H, s, H-triazolyl); δC (125 MHz; DMSO; Me4Si) 20.4 (COCH3), 20.8 (COCH3), 20.9 (COCH3), 61.5 (OCH2), 64.8 (C-5a), 66.1 (C-5), 68.5 (C-4a), 69.9 (C-4), 70.7 (C-2a), 72.6 (C-3a), 73.5 (C-2), 76.7 (C-3), 85.2 (C-1a), 102.8 (C-1), 124.0 (C-H triazolyl), 144.8 (C-triazolyl), 169.5 (COCH3), 170.5 (COCH3), 170.6 (COCH3); ESI-HMRS: m/z calcd for C19H23N3O12: [MH]+: 490.1673, found: 490.1659.
Xyloside 4g. Pale yellow solid, m.p. 67 °C. [α]20D −91.8 (c 0.39 in MeOH). δH (500 MHz; CD3OD; Me4Si) 1.87 (3H, s, COCH3), 2.04 (3H, s, COCH3), 2.07 (3H, s, COCH3), 3.19–3.29 (3H, m, 2-H and 2′-H and 5-H), 3.33–3.36 (1H, m, 3-H), 3.35–3.38 (1H, m, 5′-H), 3.47 (1H, t, J3′,2′ = J3′,4′ 9.0, 3′-H), 3.52 (1H, ddd, J4,5 5.3, J4,3 8.7, J4,5 10.3, 4-H), 3.68 (1H, ddd, J4′,5′ 5.1, J4′,3′ 9.0, J4′,5′ 10.1, 4′-H), 3.79 (1H, dd, J5a,4a 10.5, J5a,5a 11.5, 5a-H), 3.91 (1H, dd, J5,4 5.4, J5,5 11.5, 5-H), 4.06 (dd, J5′,4′ 5.3, J5′,5′ 11.5, 5′-H), 4.27 (1H, dd, J5a,4a 5.6, J5a,5a 11.5, 5a-H), 4.31 (1H, d, J1′,2′ 7.5, 1′-H), 4.35 (1H, d, J1,2 7.5, 1-H), 4.78 (1H, d, J 12.7, 1H OCH2), 4.91 (1H, d, J 12.7, 1H OCH2), 5.22 (1H, ddd, J4a,5a 5.6, J4a,3a 9.4, J4a,5a 10.5, 4a-H), 5.52 (1H, t, J3a,2a = J3a,4a 9.4, 3a-H), 5.58 (1H, t, J2a,1a = J2a,3a 9.4, 2a-H), 6.05 (1H, d, J1a,2a 9.4, 1a-H), 8.28 (1H, s, H triazolyl); δC (125 MHz; CD3OD; Me4Si) 20.2 (COCH3), 20.4 (COCH3), 20.5 (COCH3), 62.5 (OCH2), 64.6 (C-5), 66.3 (C-5a), 67.1 (C-5′), 69.8 (C-4a), 71.1 (C-4′), 72.1 (C-2a), 73.7 (C-3a), 74.3 (C-2), 74.6 (C-2′), 75.7 (C-3), 77.6 (C-3′), 78.2 (C-4), 87.3 (C-1a), 103.6 (C-1′), 104.0 (C-1), 124.4 (C-H triazolyl), 146.0 (C-triazolyl), 170.5 (COCH3), 171.4 (COCH3), 171.5 (COCH3); ESI-HMRS: m/z calcd for C24H35N3O16Na: [M + Na]+: 644.1915, found: 644.1921.
Conclusions
In conclusion, a range of original triazole-linked O-xylosides and O-xylobiosides was prepared in a straightforward chemoenzymatic two-step sequence from xylans. The synthesis of O-xylobiosides by this methodology is of particular interest since these compounds are not accessible by means of conventional carbohydrate synthesis. Preparation of propargyl xyloside and xylobioside was first investigated via an enzymatic transglycosylation reaction from xylans and propargyl alcohol using a xylanase. Different reaction parameters were studied (xylan concentration, alcohol concentration, enzyme loading, reaction time) to optimize the production of xylosides. In the second step, click reactions in an aqueous solution were carried out with various azides to give the corresponding triazoles with good yields (53% to 93%). Aliphatic, aromatic, hydroxyl, xylose-derived or aminoacid-derived groups were introduced as the substituent of the triazole ring. Biological evaluation of these new triazole-linked xylosides will be undertaken.
Acknowledgements
This work was supported by the “Région Champagne-Ardenne” (Xylocos Emergence program). The authors are grateful to the “Région Champagne-Ardenne” and FEDER for financial support and for doctoral fellowships (M. O. and C. B.). NS-22083 xylanase was kindly provided by Novozymes (France).
Notes and references
- For reviews, see:
(a) A. Corma, S. Iborra and A. Velty, Chem. Rev., 2007, 107, 2411–2502 CrossRef CAS PubMed;
(b) J. N. Chheda, G. W. Huber and J. A. Dumesic, Angew. Chem. Int. Ed., 2007, 46, 7164–7183 CrossRef CAS PubMed.
- S. J. Bennett and P. J. G. Pearson, Chem. Eng. Res. Des., 2009, 87, 1120–1139 CrossRef CAS PubMed.
- J. H. Clark, V. Budarin, F. E. I. Deswarte, J. J. E. Hardy, F. M. Kerton, A. J. Hunt, R. Luque, D. J. Macquarrie, K. Milkowski, A. Rodriguez, O. Samuel, S. J. Tavener, R. J. White and A. J. Wilson, Green Chem., 2006, 8, 853–860 RSC.
- M. FitzPatrick, P. Champagne, M. F. Cunningham and R. A. Whitney, Bioresour. Technol., 2010, 101, 8915–8922 CrossRef CAS PubMed.
- A. Ebringerova and T. Heinze, Macromol. Rapid Commun., 2000, 21, 542–556 CrossRef CAS.
- For a general review on the glycosidase-catalysed synthesis of glycosides, see: F. van Rantwijk, M. W. V. Oosterom and R. A. Sheldon, J. Mol. Catal. B: Enzym., 1999, 6, 511–532 CrossRef CAS.
- For the synthesis of xylosides using a xylosidase, see:
(a) H. Shinoyama, Y. Kamiyama and T. Yasui, Agric. Biol. Chem., 1988, 52, 2197–2202 CrossRef CAS;
(b) H. Shinoyama, Y. Gama, H. Nakahara, Y. Ishigami and T. Yasui, Bull. Chem. Soc. Jpn., 1991, 64, 291–292 CrossRef CAS;
(c) M. Gargouri, I. Smaali, T. Maugard, M. D. Legoy and N. Marzouki, J. Mol. Catal. B: Enzym., 2004, 29, 89–94 CrossRef CAS PubMed.
- For xylanase-catalysed transglycosylation reactions, see:
(a) S. Matsumura, Y. Kinta, K. Sakiyama and K. Toshima, Biotechnol. Lett., 1996, 18, 1335–1340 CrossRef CAS;
(b) S. Matsumura, K. Sakiyama and K. Toshima, Biotechnol. Lett., 1997, 19, 1249–1253 CrossRef CAS;
(c) S. Matsumura, K. Sakiyama and K. Toshima, Biotechnol. Lett., 1999, 21, 17–22 CrossRef CAS;
(d) N. Kadi, L. Belloy, P. Chalier and J. C. Crouzet, J. Agric. Food Chem., 2002, 50, 5552–5557 CrossRef CAS PubMed;
(e) Z. Jiang, Y. Zhu, L. Li, X. Yu, I. Kusakabe, M. Kitaoka and K. Hayashi, J. Biotechnol., 2004, 114, 125–134 CrossRef CAS PubMed;
(f) A. Tramice, E. Pagnotta, I. Romano, A. Gambacorta and A. Trincone, J. Mol. Catal. B: Enzym., 2007, 47, 21–27 CrossRef CAS PubMed;
(g) N. Kadi and J. Crouzet, Food Chem., 2008, 106, 466–474 CrossRef CAS PubMed;
(h) K. Chiku, H. Dohi, A. Saito, H. Ebise, Y. Kousai, H. Shinoyama, Y. Nishida and A. Ando, Biosci., Biotechnol., Biochem., 2009, 73, 1123–1128 CrossRef CAS;
(i) G. Mamo, S. Kasture, R. Faryar, S. Hashim and R. Hatti-Kaul, Process Biochem., 2010, 45, 700–705 CrossRef CAS PubMed.
- N. B. Schwartz, Trends Glycosci. Glycotechnol., 1995, 7, 429–445 CrossRef CAS.
-
(a) S. F. Penc, B. Pomahac, T. Winkler, R. A. Dorschner, E. Eriksson, M. Herndon and R. L. Gallo, J. Biol. Chem., 1998, 273, 28116–28121 CrossRef CAS PubMed;
(b) M. Bernfield, M. Götte, P. W. Park, O. Reizes, M. L. Fitzgerald, J. Lincecum and M. Zako, Annu. Rev. Biochem., 1999, 68, 729–777 CrossRef CAS PubMed;
(c) M. Delehedde, F. Allain, S. J. Payne, R. Borgo, C. Vampouille, D. G. Fernig and E. Deudon, Curr. Med. Chem., 2002, 1, 89–102 CAS;
(d) R. Sasisekharan, Z. Shriver, G. Venkataraman and U. Narayanasami, Nat. Rev. Cancer, 2002, 2, 521–528 CrossRef CAS PubMed;
(e) L. Horonchik, S. Tzaban, O. Ben-Zaken, Y. Yedidia, A. Rouvinski, D. Papy-Garcia, D. Barritault, I. Vlodavsky and A. Taraboulos, J. Biol. Chem., 2005, 280, 17062–17067 CrossRef CAS PubMed;
(f) M. M. Fuster and J. D. Esko, Nat. Rev. Cancer, 2005, 5, 526–542 CrossRef CAS PubMed.
-
(a) M. Okayama, K. Kimata and S. Suzuki, Biochem. J., 1973, 74, 1069–1073 CAS;
(b) N. B. Schwartz, L. Galligani, P.-L. Ho and A. Dorfman, Proc. Natl. Acad. Sci. U.S.A., 1974, 71, 4047–4051 CrossRef CAS;
(c) N. B. Schwartz, J. Biol. Chem., 1977, 252, 6316–6321 CAS.
-
(a) F. Bellamy, V. Barberousse, N. Martin, P. Masson, J. Millet, S. Samreth, C. Sepulchre, J. Theveniaux and D. Horton, Eur. J. Med. Chem., 1995, 30, 101s–115s CAS;
(b) J. R. Toomey, M. A. Abboud, R. E. Valocik, P. F. Koster, C. L. Burns-Curtis, K. Pillarisetti, T. M. Danoff and J. A. Erhardt, J. Thromb. Haemostasis, 2006, 4, 1989–1996 CrossRef CAS PubMed;
(c) A. L. Myers, V. V. Upreti, M. Khurana and N. D. Eddington, J. Clin. Pharmacol., 2008, 48, 1158–1170 CrossRef CAS PubMed.
-
(a) K. Mani, B. Havsmark, S. Persson, Y. Kaneda, H. Yamamoto, K. Sakurai, S. Ashikari, H. Habuchi, S. Suzuki, K. Kimata, A. Malmstrom, G. Westergren-Thorsson and L.-A. Fransson, Cancer Res., 1998, 58, 1099–1104 CAS;
(b) K. Mani, M. Belting, U. Ellervik, N. Falk, G. Svensson, S. Sandgren, F. Cheng and L.-A. Fransson, Glycobiology, 2004, 14, 387–397 CrossRef CAS PubMed;
(c) M. Jacobsson, U. Ellervik, M. Belting and K. Mani, J. Med. Chem., 2006, 49, 1932–1938 CrossRef CAS PubMed;
(d) U. Nilsson, R. Johnsson, L.-A. Fransson, U. Ellervik and K. Mani, Cancer Res., 2010, 70, 3771–3779 CrossRef CAS PubMed.
-
(a) N. Pineau, F. Bernerd, A. Cavezza, M. Dalko-Csiba and L. Breton, Eur. J. Dermatol., 2008, 18, 36–41 CAS;
(b) J. Sok, N. Pineau, M. Dalko-Csiba, L. Breton and F. Bernerd, Eur. J. Dermatol., 2008, 18, 297–302 CAS;
(c) A. Cavezza, C. Boulle, A. Guéguiniat, P. Pichaud, S. Trouille, L. Ricard and M. Dalko-Csiba, Bioorg. Med.
Chem. Lett., 2009, 19, 845–849 CAS;
(d) E. Vassal-Stermann, A. Duranton, A. F. Black, G. Azadiguian, J. Demaude, H. Lortat-Jacob, L. Breton and R. R. Vivès, PLoS One, 2012, 7, e47933 CAS.
-
(a) B. Kuberan, M. Ethirajan, X. V. Victor, V. Tran, K. Nguyen and A. Do, ChemBioChem, 2008, 9, 198–200 CrossRef CAS PubMed;
(b) X. V. Victor, T. K. N. Nguyen, M. Ethirajan, V. M. Tran, K. V. Nguyen and B. Kuberan, J. Biol. Chem., 2009, 284, 25842–25853 CrossRef CAS PubMed;
(c) K. Raman and B. Kuberan, Mol. BioSyst., 2010, 6, 1800–1802 RSC;
(d) Y. Tsuzuki, T. K. N. Nguyen, D. R. Garud, B. Kuberan and M. Koketsu, Bioorg. Med. Chem. Lett., 2010, 20, 7269–7273 CrossRef CAS PubMed.
- D. Goyard, M. Baron, P. V. Skourti, A. S. Chajistamatiou, T. Docsa, P. Gergely, E. D. Chrysina, J.-P. Praly and S. Vidal, Carbohydr. Res., 2012, 364, 28–40 CrossRef CAS PubMed.
- H. Ding, R. Yang, Y. Song, Q. Xiao and J. Wu, Nucleosides, Nucleotides Nucleic Acids, 2008, 27, 368–375 CAS.
- E. Davis Oldham, S. Seelam, C. Lema, R. J. Aguilera, J. Fiegel, S. E. Rankin, B. L. Knutson and H.-J. Lehmler, Carbohydr. Res., 2013, 379, 68–77 CrossRef PubMed.
- S. Gatard, L. Liang, L. Salmon, J. Ruiz, D. Astruc and S. Bouquillon, Tetrahedron Lett., 2011, 52, 1842–1846 CrossRef CAS PubMed.
- A. S. Rowan, N. I. Nicely, N. Cochrane, W. A. Wlassoff, A. Claiborne and C. J. Hamilton, Org. Biomol. Chem., 2009, 7, 4029–4036 CAS.
- M. Muzard, N. Aubry, R. Plantier-Royon, M. O′Donohue and C. Rémond, J. Mol. Catal. B: Enzym., 2009, 58, 1–5 CrossRef CAS PubMed.
-
(a) M. Ochs, M. Muzard, R. Plantier-Royon, B. Estrine and C. Rémond, Green Chem., 2011, 13, 2380–2388 RSC;
(b) C. Rémond, M. Ochs, M. Muzard, R. Plantier-Royon and B. Estrine, Fr. Pat., 2 967 164–A1, 2012.
- W.-Y. Lu, X.-W. Sun, C. Zhu, J.-H. Xu and G.-Q. Lin, Tetrahedron, 2010, 66, 750–757 CrossRef CAS PubMed.
-
(a) R. Huisgen, Angew. Chem. Int. Ed., 1963, 2, 565–598 CrossRef;
(b) R. Huisgen, Angew. Chem. Int. Ed., 1963, 2, 633–696 CrossRef.
- For some recent reviews on click chemistry, see:
(a) H. C. Kolb and K. B. Sharpless, Drug Discovery Today, 2003, 8, 1128–1137 CrossRef CAS;
(b) A. Dondoni, Chem. – Asian J., 2007, 2, 700–708 CrossRef CAS PubMed;
(c) M. Meldal and C. W. Tornoe, Chem. Rev., 2008, 108, 2952–3015 CrossRef CAS PubMed;
(d) V. Arago-Leoneti, V. L. Campo, A. S. Gomes, R. A. Field and L. Carvalho, Tetrahedron, 2010, 66, 9475–9492 CrossRef PubMed;
(e) S. D. Roughley and A. M. Jordan, J. Med. Chem., 2011, 54, 3451–3479 CrossRef CAS PubMed;
(f) D. Kushwaha, P. Dwivedi, S. K. Kuanar and V. K. Tiwari, Curr. Org. Synth., 2013, 10, 90–135 CAS.
-
(a) H. C. Kolb, M. G. Finn and K. B. Sharpless, Angew. Chem. Int. Ed., 2001, 40, 2004–2021 CrossRef CAS;
(b) V. V. Rostovtsev, L. G. Green, V. V. Fokin and K. B. Sharpless, Angew. Chem. Int. Ed., 2002, 41, 2596–2599 CrossRef CAS.
- C. W. Tornoe, C. Christensen and M. Meldal, J. Org. Chem., 2002, 67, 3057–3064 CrossRef CAS PubMed.
- J. Hou, X. Li, J. Shen, G. Zhao and P. G. Wang, Expert Opin. Drug Discovery, 2012, 7, 489–501 CrossRef CAS PubMed.
- K. Holmqvist, A. Persson, R. Johnsson, J. Löfgren, K. Mani and U. Ellervik, Bioorg. Med. Chem., 2013, 21, 3310–3317 CrossRef CAS PubMed.
- C.-O. Abrahamsson, U. Ellervik, J. Eriksson-Bajtner, M. Jacobsson and K. Mani, Carbohydr. Res., 2008, 343, 1473–1477 CrossRef CAS PubMed.
- R. Johnsson, K. Mani and U. Ellervik, Bioorg. Med. Chem., 2007, 15, 2868–2877 CrossRef CAS PubMed.
-
(a) T. K. N. Nguyen, V. M. Tran, V. Sorna, I. Eriksson, A. Kojima, M. Koketsu, D. Loganathan, L. Kjellén, R. I. Dorsky, C.-B. Chien and B. Kuberan, ACS Chem. Biol., 2013, 8, 939–948 CrossRef CAS PubMed;
(b) V. M. Tran, T. K. N. Nguyen, V. Sorna, D. Loganathan and B. Kuberan, ACS Chem. Biol., 2013, 8, 949–957 CrossRef CAS PubMed.
- D. M. Nguyen and D. H. Miles, Synth. Commun., 2011, 41, 1759–1771 CrossRef CAS.
- K. Barral, A. D. Moorhouse and J. E. Moses, Org. Lett., 2007, 9, 1809–1811 CrossRef CAS PubMed.
Footnote |
† Present address: LISBP, INSA de Toulouse, 135 Avenue de Rangueil, 31077 Toulouse Cedex 04, France. |
|
This journal is © The Royal Society of Chemistry 2014 |
Click here to see how this site uses Cookies. View our privacy policy here.