DOI:
10.1039/C3RA46304D
(Review Article)
RSC Adv., 2014,
4, 12422-12440
Benzimidazole-biologically attractive scaffold for protein kinase inhibitors
Received
31st October 2013
, Accepted 12th December 2013
First published on 18th December 2013
Abstract
Great advances in elucidating molecular structures allow the precise determination of the interactions between a protein and a therapeutic agent. Enzyme inhibitors are used as a therapeutic agent with organic molecules, that interact with their targets through the weak linkages of hydrogen bonding and van der Waals interactions. These reduce the undesirable side effects and allow more non-specific interactions with non-target molecules. Benzimidazole acts as an enzyme inhibitor that may interact with different proteins and enzymes and has inspired chemists to carry out various structural variations of it. This review discusses the development of distinct benzimidazoles with an array of enzyme inhibitors viz., aurora kinase inhibitors, cyclin-dependent kinase inhibitors, mitogen activated protein kinase inhibitors, polo like kinase inhibitors, Tie kinase inhibitors, lymphocyte specific kinase inhibitors etc., also highlighting the molecular interaction with enzyme inhibitors. Various derivatives of benzimidazole, with different inhibitory activities, have been described on the basis of substitution around the central moiety, with an aim to help medicinal chemists to develop structure–activity relationships. The reviews in the literature till now are focused only on the biological activities of benzimidazole viz., antiviral, anticancer and antifungal, but the present review focuses on the latest work, describing the inhibitor aspects and the potential of the benzimidazole ring. This discussion will further help in the development of novel benzimidazole compounds.
Prinka Singla | Prinka Singla is a PhD student of Dr Kamaldeep Paul in the Thapar University Lab. She obtained both BSc (2008) and MSc (2010) degrees from Punjabi University, Patiala. She then joined Thapar University, Patiala for her PhD study. Her research interests include synthesis and characterization of biologically inspired heterocyclic moieties and their applications as anticancer drugs. |
Vijay Luxami | Vijay Luxami was born in Gurdaspur, India in 1983. She received her PhD from the Department of Chemistry, Guru Nanak Dev University Amritsar in 2010 and joined the School of Chemistry and Biochemistry, Thapar University, Patiala as an Assistant Professor. She has received DST Young Scientist, INSPIRE Faculty Fellow and DFG awards. Presently, she is a visiting scientist at the University of Bath, UK. Her area of research is supramolecular materials and their applications in molecular electronic materials. |
Kamaldeep Paul | Kamaldeep Paul is the Assistant Professor of synthetic organic and medicinal chemistry at the School of Chemistry and Biochemistry, Thapar University, Patiala, India. He received his MSc degree from the Department of Pharmaceutical Sciences, and a PhD in Synthetic Organic Chemistry from the Department of Chemistry, Guru Nanak Dev University, Amritsar in 2006. His area of research is synthetic organic and medicinal chemistry where his research is broadly focused on heterocycles-containing hybrid moieties and evaluation in vitro for multidrug resistance (MDR) modulators, kinase inhibitors (anticancer), antimalarial and antituberculosis activities. |
1 Scope of the review
Cancer is an uncontrolled growth of abnormal cells in the body which do not die, forming malignant tumors, and invade nearby parts of the body. The tumors can grow and interfere with the digestive, nervous, and circulatory systems and release hormones that alter body functions. The available anticancer drugs have a distinct mechanism of action, which may vary in the effects on different types of normal and cancer cells. The effectiveness of many anticancer drugs is limited by their toxicity to normal rapidly growing cells in intestinal and bone marrow areas. Cancer cells which are initially suppressed by a specific drug, may develop a resistance to that drug. In recent years, great advances in elucidating molecular structures have allowed the precise determination of the interaction between proteins and therapeutic agents. Identifying the exact nature of an interaction with a drug is the key to controlling its specificity and reducing unwanted side effects.1 Designed molecules bind to specific targets, and many of these efforts are aimed at inhibiting enzymes. Enzyme inhibitors have been used as therapeutic agents with organic molecules, as these agents interact with targets through the weak linkage of hydrogen bonding and van der Waals contact, that results in undesirable side effects, due to the shorter residency time of the drug at the target, allowing more non-specific interactions with non-target molecules. Protein phosphorylation, the most prevalent post-translational process in living cells, is catalyzed by protein kinases.2 Aberrant kinase activity is implicated in many diseases, and makes this target class attractive for the pharmaceutical industry. They require an essential divalent metal ion, usually Mg2+, to facilitate the phosphoryl transfer reaction and assist in ATP binding. The nucleotide pocket of a protein kinase utilizes both ATP and GTP as phosphate donors and displays a unique feature for being smaller than some unique bulky residues, hampering the entrance of large molecules, like staurosporine. The first protein kinase activity, described in 1954 by Burnett and Kennedy, was found in rat liver mitochondria, using casein as a substrate.3 The abnormal phosphorylation of proteins, mediated by a kinase, resulted in diseases, which include cancer, diabetes, rheumatoid arthritis and hypertension. The structural similarities of the benzimidazole nucleus to biological compounds, such as the purine base of DNA and the occurrence of this nucleus in vitamin B12 have been of great interest to the pharmaceutical industry.4 This heterocycle may represent a kind of privileged substructure, which may interact with different proteins and enzymes. Indeed, a number of important drugs used in different therapeutic areas contain the benzimidazole ring, as proton pump inhibitors (omeprazole), antihypertensives5 (candesartan, telmisartan), antihistaminics (astemizole), antihelmintics6 (albendazole, mebendazole), as well as several other kinds of still investigational therapeutic agents, including antitumorals7 and antivirals.8 Amongst these, it has been shown that benzimidazole possesses a high antitumor activity towards various human cells and the aim of this review is to highlight it’s use in potential anticancer agents, as having affinity to bind with various enzyme receptors.
2 Chemistry
Benzimidazole9 is conveniently synthesized by the reaction of the corresponding o-phenylenediamine with an acid/aldehyde (Scheme 1). This allows the production of a large family of derivatives, including substitution at the 1- or 2-position. Additionally, the benzimidazole ring can be substituted at the 4, 5, 6 or 7-positions, by nitro (1), amino (2) or halogen groups. This not only allows the introduction of other functional groups, which can be used for targeting biomolecules, but can have a major effect on the electronic properties, with a consequent influence on the chemical, photochemical and spectroscopic properties.
 |
| Scheme 1 Synthesis of substituted benzimidazole. | |
The literature precedence reveals that the substitution at the 1, 2, 5 and/or 6-positions of the benzimidazole moiety is also crucial from the point of view of medicinal chemistry, to exhibit a wide range of pharmacological activities10 by acting at different targets. Accordingly, the compounds may be mono-, di- or tri-substituted derivatives of the benzimidazole nucleus. Substitution of an amino group at the 2-position of the benzimidazole pharmacophore is capable of allowing an interaction with nucleotidic structures and enzymes, as well as with various enzyme inhibitor.11 The derivatization at the N–H position of benzimidazole by an electron donating group and substitution with a long chain of propyl, acetamido, thio, thiazole-amino, or tetramethyl piperidine, resulted in good biological activity.12 A 2-phenyl substituent13 and/or 5-nitro group14 improved the binding on proteins, through hydrogen bond formation, and/or its electron withdrawing properties, which resulted in π-interactions between the electron deficient ring of the drug and the electron rich ring of the aromatic amino acids. Terminal lipophilic groups are also important for the receptor binding affinity, with improved inhibition profiles, solubility and metabolic stability.15 Bis-benzimidazole derivatives, with an alkyl chain as a linker are also important for anticancer activity.16
3 Biological activities
Enzyme inhibition, as a mode of action for drugs has often been discovered almost as an afterthought. Detailed structural information can be employed to design molecules that bind to specific targets, and many of these efforts are aimed at enzymes. The substrate specificity of enzymes, often recognizing a single molecule only, offers the possibility of constructing a drug with a well-defined behavior. Since the blocking of an enzyme's activity can kill a pathogen or correct a metabolic imbalance, many drugs act as enzyme inhibitors. The binding of an inhibitor can stop a substrate from entering the enzyme's active site and/or hinder the enzyme from catalyzing its reaction, either reversibly or irreversibly. Benzimidazole is isosteric with the indole and purine nuclei,17 both of which bind reversibly and irreversibly to enzymes, present in a number of fundamental cellular components and bioactive compounds. Benzimidazole is usually attributed to their selective binding to parasite β-tubulin18 and has been shown to bind specifically to the DNA minor groove, serotonin receptors (5-HT), histamine (H4) receptors, dopamine (D4) receptors,19 VEGFR, G protein-coupled receptors (GPCRs) and act as inhibitor of anaplastic lymphoma kinase, c-Abl kinase, casein kinase 2, ATR kinase, tyrosine kinase, thymidylate kinase, ubiquitination inhibitors, TCS inhibitors (3), PARP-1 inhibitor (4),20 NK2 antagonist (5),21 inhibit a large array of DNA and RNA processing enzymes, such as topoisomerase 1 (6),22 DNA and RNA polymerases 1 (7)23 etc. (Fig. 1). Benzimidazoles also target histone deacetylase 2, the inhibition of phosphodiesterase IV, antagonism of angiotensin 1, neuropeptide Y binding, inhibition of proton pumps and DNA intercalating agents (8).24 They also have endowed activity against the Coxsackie virus B3, and the targeting of specific hepatitis C virus enzymes.25
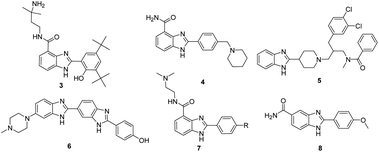 |
| Fig. 1 Substituted benzimidazole derivatives as receptors. | |
As shown in Scheme 2, compound 9 possesses a terminal lipophilic group, that is important for the Y5 receptor binding affinity and also exhibits Y5 antagonistic activity.26 The introduction of metabolically stable and rigid aromatic linkers, like the phenyl linker (10) in place of the –S–CH2– moiety endowed improved CYP450 inhibition profiles, good solubility and high metabolic stabilities while a meta-phenyl has a very high binding affinity compared to the para-phenyl, but the ortho-phenyl loses the Y5 receptor binding affinity.15
 |
| Scheme 2 Design of benzimidazole derivatives exhibiting Y5 antagonistic activity. | |
The search for small molecules capable of down regulating kinase activity was primarily addressed toward the nucleotide pocket of the kinase, exploiting structural differences in this region for molecular recognition. However, due to the high conservation of the ATP-binding site, the development of absolutely specific ATP-competitive inhibitors is hard to attain and the possibility to develop allosteric inhibitors is being explored. In this review, we classified protein kinase inhibitor on the basis of an enzyme inhibitor that represents the most promising drug targets for cancer chemotherapy and this article also reviews those lessons and their application in the development of other agents, targeting these and other kinases that have more recently entered the development pipeline.
3.1. Aurora kinase inhibitors
The serine–threonine kinases, aurora A and B, are regulatory proteins that play a key role in the mitotic events of cell division, centrosome maturation, separation and regulate spindle assembly and stability.27 Both aurora A and aurora B play roles in tumor cells in various organs including the colon, breast, pancreatic, gastric and prostate.28 VX-680 was the first inhibitor of aurora kinase that entered clinical trials.29 Therefore, aurora kinases have emerged as an attractive oncology target for small-molecule drug discovery.30 Benzimidazole derivatives have also been explored thoroughly for the development of aurora kinase inhibitors as anticancer agents.31
Structurally, SNS-314 (ref. 32) as a purine mimetic and biaryl urea residue, is an essential binding element in the DFG pocket with a low aqueous solubility, due to the presence of a biaryl urea moiety (Fig. 2).33 2-Aminobenzimidazole (11), as a bioisostere of the biaryl urea residue, acts as an aurora kinase inhibitor with an improved intrinsic solubility.
 |
| Fig. 2 Aurora kinase inhibitors. | |
Compound 12 (ref. 34) with a phenyl linker exhibited comparable potency to compound 11 (Fig. 3). Compound 13a showed drastically reduced activity in an aurora B assay, but an increase in the chain length (13b) demonstrated good potency in aurora A and B and also improved the aqueous solubility. The ‘NH’ moiety in the benzimidazole residue is important for the activity, as the N-methyl benzimidazole analogue displays a reduced potency against aurora B. The replacement of the benzimidazole residue with a substituted imidazole moiety significantly decreases the potency against aurora B. Thus, a benzimidazole analogue maintains a comparable potency, while having an improved solubility. The optimization of the pyrazole-benzimidazole series has been focused on maximizing the in vitro potency for both the aurora A and aurora B isoforms, in addition to routinely screening for a polyploid phenotype in HCT116 cells.35 A pyrazole-benzimidazole motif has excellent complementarity with the narrow region of the ATP pocket. The pyrazole-benzimidazole (14) has been identified during the course of a CDK program as a ligand efficient (LE = 0.59) fragment starting point for the discovery of aurora kinase inhibitors. The introduction of a fluorine atom at the para position of the phenyl ring (15b) of compound 15a stabilized the molecule in vivo by reducing its electron density.36 An initial strategy to improve the enzyme potency of a pyrazol-4-yl urea substituted benzimidazole analogue has been aimed at reinforcing the twisted conformation of the phenyl ring, by introducing an o-fluoro or 2,6-difluoro substituent e.g. 16a (aurora A IC50 = 2.8 nM) and 16b (aurora A IC50 = 1.5 nM) respectively, have been correlated with an improved activity in the HCT116 cellular assay (polyploidy observed as 0.1–0.3 and 0.03 μM, respectively). Thus, the benzimidazole and pyrazole series of compounds with aromatic substitution at the pyrazole groups play an important part for aurora kinase inhibitors and make it a most active moiety.
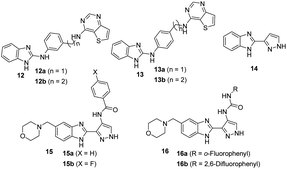 |
| Fig. 3 Benzimidazole analogs as aurora kinase inhibitors. | |
3.2. Cyclin-dependent kinase inhibitors
Cyclin-dependent kinase (CDKs), serine–threonine protein kinases, can generally be divided into two groups, based on their roles in cell-cycle progression and transcriptional regulation.37 A strong association between CDKs and cancer biology has promoted the development of pharmacological small-molecule CDK inhibitors that can be used for cancer therapy.38
Verma et al.39 have reported the activity pattern of a substituted benzimidazole at the C-2 and C-4 positions of pyrimidine.40 The presence of an aminobenzimidazole group at either the C-2 and/or C-4 positions of pyrimidine (17) is critical for CDK1 inhibitory activity (Fig. 4). Trifluoromethyl or bromo groups have been shown to be optimal at the C-5 position of the pyrimidine ring, while substitution with hydrogen or fluorine results in a 200- or 10-fold decrease in potency, respectively. Analogues with a substituted aniline at the C-2 position and benzimidazole at the C-4 position of the pyrimidine ring (18) have displayed good activity in the CDK1 biochemical assay. Substitution at the 3- or 4-position of the aniline ring is preferred, as a substituent at the 2-position results in a loss of potency. The greatest potency has been shown by analogs bearing aniline, containing a sulfonamide group (18b), which displayed a 100-fold improvement in potency compared to the lead compound (Fig. 5). A C-2 NH group comes into contact with the hinge region at Leu-83, through two hydrogen bonds, crucial for activity.41 The substitution of benzimidazole at the C-2 position has been more tolerated than at the C-4 position of pyrimidine (19). Also, compounds having an O-linker at C-4 (19d) were also tolerated and observed to be more potent than those having a S-linker (19e).
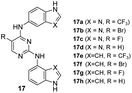 |
| Fig. 4 Aminobenzimidazole-pyrimidine based CDK1 inhibitors. | |
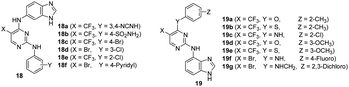 |
| Fig. 5 C-2 modified analogs. | |
Larger groups at C-4 disrupt the stabilizing hydrophobic interaction, thereby resulting in a reduced potency (20 or 21). Thus, the potency in the C-2 modified series is limited to those groups, which are capable of adopting a critical hydrogen bonding pattern to the hinge region, viz. aminobenzimidazoles and anilines. Substituted phenyl groups replacing alkyl groups (Fig. 6) within the O-linked series (22) have been well tolerated in the CDK1 assay and these analogs are generally tested below 100 nM.
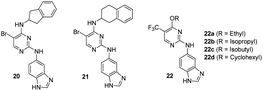 |
| Fig. 6 C-4 modified analogs. | |
Sondhi et al.42 have synthesized and evaluated in vitro an acridinyl-benzimidazole analogue for cyclin-dependent kinase (CDK-1 and CDK-5). The nature of the substituents on the benzimidazole ring of the acridinyl-benzimidazole derivatives has a major impact on the CDK inhibitory activity.43 Compounds 23a–b have shown moderate activity against CDK-5 and CDK-1. The structures of the active compounds indicate that substitution of Y = Cl for 23a and Y = NO2 for 23b have enhanced the potency, due to the presence of a hydrogen bonding/acceptor–donor in the benzimidazole (Fig. 7), that probably favors interaction with the active site of the CDK enzymes, whereas other analogues lost their potency.
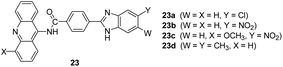 |
| Fig. 7 Acridinyl-benzimidazole analogs for cyclin-dependent kinase. | |
5,6-Dichloro-1-β-D-ribofuranosylbenzimidazole (DRB, 24) is an adenosine analogue that inhibits RNAP II transcription, both in vitro and in vivo, by competing with ATP44 and is the most selective CDK9 inhibitor. It inhibits CDK9 potently, with an IC50 value of 600 nM and shows excellent selectivity against other CDKs, but this selectivity is not associated with the level of cellular potency essential for anticancer agents in discovering pre-clinical and clinical candidates.45 The 5,6-dichloro group of the benzimidazole nucleus replaced with either a fluoride (25b) or methoxide group (25c) in the context of 1-β-D-ribofuranosylbenzimidazole, did not offer any superiority over DRB, in terms of the cytotoxic effects on the tumor cell lines.46 Similarly, the introduction of a methyl or ethyl group (Fig. 8) at the 2-position failed to enhance activity (25d).
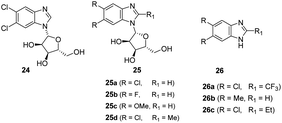 |
| Fig. 8 Structure of DRB and the designated derivatives. | |
Compound 26 showed a good antiproliferative activity in both HCT-116 and MCF-7 cells, with GI50 values of 7 μM and 6 μM, respectively47 and has been shown to have 3.5 and 6-fold increased potency than DRB. Analogues with different substitutions at the 2-position, including alkyl, in the context of 5,6-dichlorobenzimidazole, had little effect on the cell viability. The compound with an N-benzyl benzimidazole exhibited a more than threefold increase in the antiproliferative effect, compared with DRB, indicating the improved tolerance of a larger ring at the 1-position of the benzimidazole moiety.
Lin et al. have synthesized and evaluated the novel 3-benzimidazol-2-ylpyrazolo[3,4-b]pyridine analogue (27) as a potent CDK inhibitor and anti-proliferative agent.48 The structural modification of the 3-benzimidazoyl pyrazolo[3,4-b]pyridine series with the pyrazole, imidazole and pyridinyl or isoquinoyl groups has been critical for better CDK inhibitory activity49 with improved physicochemical, pharmacokinetic, and solubility properties. To the parent 3-benzimidazol-2-ylpyrazolo[3,4-b]pyridine (27) analogue, the 3-ethylamino methyl substituent (28c) confers a favorable CDK1 potency compared to the unsubstituted (28a), methoxy (28b) and other substituted compounds (Fig. 9).50 Thus, the benzimidazole-pyrimidine analogue with different substitution at the 4 or 5-positions of the pyrimidine moiety makes most active compounds for CDK inhibitors.
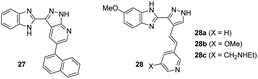 |
| Fig. 9 Pyrazolopyridine bearing benzimidazole analogs as CDK inhibitors. | |
3.3. Mitogen activated protein kinase inhibitors
Mitogen activated protein kinase (MEK) inhibitors,51 ATP utilizing serine–threonine kinases, are a vital mediator of a number of cellular activities, including growth, proliferation, regulation of inflammation, and apoptosis. MAPK has been proved to be a target for the development of therapeutics for immunologic diseases including squamous cell carcinoma, prostate and breast cancers.52
The presence of the 1-isopropyl group (29) has shown a better activity than a larger substituent at the enzyme CDK5.53 4-Mono-benzyl amino derivatives have displayed a better interaction than the 4-dibenzyl amino compounds, and a simple methoxy at the 6-position of benzimidazole (Fig. 10) has more potency than the alkoxy or benzyl derivatives, but hydrogen bond donor groups have been found essential for activity.54 Compounds 30a–d have been shown to be most selective for the inhibition of EGF induced ERK5 phosphorylation versus inhibiting pERK1/2 formation. Compound 30d has been selected for the role of selective inhibition of MEK5 versus MEK1/2, as this compound has the greatest decrease in MEK5 inhibition, relative to MEK1/2. Substitution at the 1-position of benzimidazole with the isopropyl (31–32), cyclopentyl (33–34) and benzyl (35) groups showed a significant hydrophobic interaction at a common kinase aromatic binding region, proximal to the H-bond donor–acceptor pair54 and this contribution has also been dependent on substitution at both the 4- and 6-positions of benzimidazole. Interestingly, compounds 32 and 34 possessing the hydroxybutyl ether at the 6-position (Fig. 11) have been found to be inactive in preventing EGF-induced ERK phosphorylation, but compound 35 has been shown selective to inhibit the growth of MCF-7 cells in a single dose survey.55
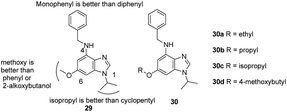 |
| Fig. 10 Inhibitors of EGF-induced ERK5 phosphorylation. | |
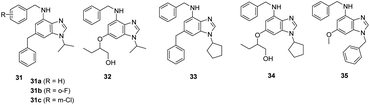 |
| Fig. 11 N-1, C-4 and C-6 modified analogs of benzimidazole as MAPK inhibitors. | |
Bamford and co-workers modified the 2-[3-amino-1,2,5-oxadiazol-5-yl] benzimidazole (36) (MSK-1 IC50 = 140 nM), to a highly potent (1H-imidazo[4,5-c]pyridin-2-yl)-1,2,5-oxadiazol-3-ylamine derivative (37) (MSK-1 IC50 = 3 nM).56 Compound 37 showed an improved potency with respect to compound 36, due to H-bonding interactions of the amino-oxadiazole with the hinge region protein backbone and the N5 of the imidazopyridine with an amino acid residue. Compound 38 had a low nanomolar activity in both ATP competitive enzyme binding and the inhibition of TNFα release in macrophages.57 Substitution at N1 with a lipophilic functionality, such as cyclohexyl or cyclopentyl, affords potent MSK-1 inhibitory compounds.58 The introduction of substituents containing a basic nitrogen, such as piperidin-4-yl and 4-butylamino derivatives, retains MSK-1 potency, an advantage from the straight alkyl chain analogue, although the shorter chain shows some improved selectivity over RSK-1. However, the more bulky 3-piperidinyl compound (39a) combined the improved MSK-1 potency and modest RSK-1 selectivity of the cyclohexyl derivative (39b) with the modest GSK-3 selectivity of the 4-piperidinyl analogue (Fig. 12).
 |
| Fig. 12 Effect of substitution on benzimidazole for p38a MAPK. | |
Mono- and dialkyl substitution with the C-2 amino group of pyrazole (not essential for enzyme activity) resulted in decreased potency (40). The removal of the sulfonyl group at N-1 of the benzimidazole (impact on cellular activity) also diminished activity; substitution with an alkyl group at the N-1 position and amino at the C-2 position (40b) are also well tolerated, but an aryl group is not.59 In this series, the benzimidazole and amino oxadiazole hybrids, with alkyl groups at the N1 position of benzimidazole, make these molecules the most active compounds.
3.4. Polo like kinase inhibitors
Polo-like kinase (PLK) is an attractive target for cancer treatment through interference60 with the cell cycle that includes PLK1, PLK2, PLK3 and PLK4. Compound 41 has been found to be a potent inhibitor of both PLK1 and PLK3 and moderately inhibits the growth of tumor cells and also has a desirable in vitro activity. Its aqueous solubility is poor for an intravenous formulation required for the target product profile, while compound 42 is more than 300-fold selective for the inhibition of PLK1 over PLK3, for evaluating the inhibition of polo-like kinases as an approach to cancer treatment.
In turn, while the aqueous solubility has dramatically improved in 42, with heterocyclic substitution at the 5- or 6-position of the benzimidazole, by maintaining potency for the inhibition of PLK1 and PLK3. However, 5-substituted benzimidazoles have exhibited a 2–3 fold increased cellular potency over their 6-substituted counterparts. Several examples have also displayed potent activity against PLK3, although this activity is reduced in the presence of a heteroatom at the ortho-position of the heteroaryl ring (Fig. 13).61 There is a difference in the enzyme potency between compounds with a 2-CF3 or 2-Cl substitution on the benzyl ether (43a–b); the 2-Cl benzyl ether has shown slightly more potency at the cellular level. Reducing the CYP450 inhibition of these heteroaryl-linked compounds is possible, by adding a polar functionality to the more metabolically stable pyrazole and amino pyridine heteroaryl rings (44a–c). The inhibition of PLK1 is not sensitive to the presence of a secondary or tertiary amine at the 2-position of the pyridine, or to the presence of a pendant non-basic amine. Similarly, the potency of the substituted pyrazoles is not sensitive to the length of the linker chain, or the type of basic amine attached.
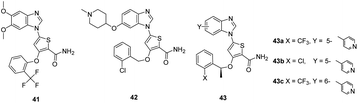 |
| Fig. 13 Inhibitors of PLK1 and PLK3. | |
Compound 45a is a potent inhibitor of PLK1 and PLK3 and moderately inhibits the growth of tumor cells in vitro. The substitution of the methyl group at the benzylic carbon (Fig. 14) in the R-configuration (45b) resulted in more than a 40-fold improvement in the ability to inhibit the growth of HCT116 cells (colorectal carcinoma) over the unsubstituted analogue (45a). The effect of benzylic substitution on the PLK3 potency is similar to that observed with PLK1 and the enantiomer is a less potent inhibitor of PLK1 at the enzymatic level; however, its potency in the cellular assay is similar to 45b. The 6-position of the benzimidazole might be a good location for appending such type of groups, as it is oriented towards the solvent. In addition, the incorporation of a diol (46b) and flexible amine chains (46c) has improved the solubility and reduced the protein binding, relative to 46a.62 Meanwhile, 46d is quite potent and the potency in the cellular assay has been further enhanced by the elimination of the methylene spacer (Fig. 15), between the oxygen atom and the piperidine ring (46e). 46e exhibited an excellent combination of potency, solubility and reduced protein binding and proved to be a potent inhibitor of cell growth in vitro across a wide range of cell lines, representing multiple tumor types. The stereochemistry also defined the activities of the molecules, as compound 45b having an R configuration, with thiophene at the N1 position of benzimidazole, proved to be the most active compound in this series, for polo-like kinase inhibitors.
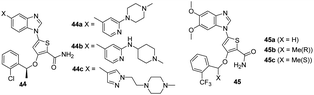 |
| Fig. 14 Heteroaryl-linked (2-thienyl)-1H-benzimidazoles. | |
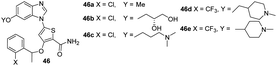 |
| Fig. 15 Effects of C-6 substitution on benzimidazole for polo like kinase inhibitors. | |
3.5. Insulin-like growth factor-1 receptor kinase inhibitors
The insulin-like growth factor-1 receptor (IGF-1R) is a transmembrane receptor tyrosine kinase, that plays a critical role in mitogenesis63 and survival in a variety of human tumor cells,64 such as colon, breast, prostate, and lung tumors. Compound 47, an inhibitor from the benzimidazole class, shows in vivo efficacy in IGF and has potential for drug–drug interactions, due to potent CYP3A4 inhibition (0.5 μM) and poor aqueous solubility (<1 μg mL−1). The unsubstituted piperazine (48) showed a threefold improvement in the enzymatic potency, while the cell-based activity is maintained, relative to morpholine. To improve the overall profile of 47, modification was done by changing the substitution at the C-5 position65 as well as the phenethylamine side chain (Fig. 16) by more polar N-substituted piperazines 49.
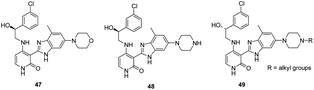 |
| Fig. 16 IGF-1R for imidazole replacements. | |
The installation of a small alkyl chain on the distal nitrogen of the piperazine resulted in an improvement of the enzyme potency, cell potency, and solubility, with a modest improvement in the CYP inhibition profile. The replacement of the morpholine of 47 with other bioisosteres, viz. 4-hydroxypiperidine, alkoxypiperidines and piperidin-4-one spiroketal, resulted in potent IGF-1R kinase inhibitors, but little improvement with respect to the CYP3A4 inhibition.66 4-Amino-piperidine inclusion reduced by 5- to 10-fold the CYP3A4 inhibition, compared to morphiline, but their oral exposure is extremely low. Replacing these basic amines with amides or carbamates resulted in compounds with a significantly increased oral exposure and improved cell potency, but stronger CYP3A4 inhibition.67
Substitution of the lipophilic (m-chlorophenyl) ethanol side chain of BMS-536929 (50) with various heterocyclic side chains, like the imidazolethanamine series (51) improved the IGF-1R potency. Substitution of the C-5 imidazolyl hydrogen in the 2-(1H-imidazol-4-yl)ethylamine series with chlorine (51a–b) increased by 5-fold the potency towards IGF-1R, but larger groups at C-5 were not tolerated (51c).68 The substitution of the C-3 position within this series showed an improved potency over such identically substituted C-5 regioisomers. The substitution of a halogen at the C-4 pyrazole position (52e) showed an excellent improvement in the potency and oral exposure. Substitution at the N-3 position of the pyrazole with a methyl group showed an improved potency over such an identically substituted C-5 (Fig. 17).
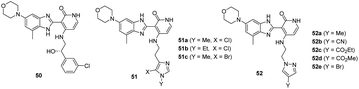 |
| Fig. 17 IGF-1R activities of the side chain appended at the 4 position of pyridone. | |
Compound 53, as a prototype of a new structural class of IGF-1R kinase inhibitors and the optimization of 53 by installing basic amine side chains at the C-4-position of the pyridone led to the identification of 54–56, with IC50 values of 390, 530, and 180 nM, respectively. The pendant imidazole moiety at the C-5 position of the benzimidazole has also been responsible for potent CYP inhibition. The replacement of the imidazole moiety with both the bromo and cyano groups (Fig. 18) has improved the potency (57). Compounds that lack an imidazole displayed a fourfold reduction in the IGF potency (57–58), relative to 56, 59 and 60. However, the N-methyl imidazoline 59 displayed an impressive 93 nM IGF-1R activity and even a reduction in cytochrome p450 inhibition.
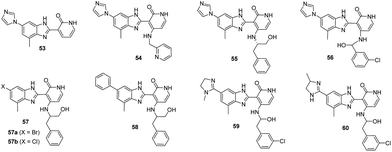 |
| Fig. 18 IGF-1R activities of phenylethylamine analogs. | |
The potency has been enhanced by exploiting the unfilled pocket that was accessed via the methyl group on the benzimidazole or the 4-position of the pyridone ring (61).69 The addition of secondary amines and anilines provided compounds that were devoid of IGF-1R inhibitory activity, but the addition of primary amines provided compounds with encouraging IGF-1R inhibitory activity.
When the methyl group at the C-5 position of the benzimidazole ring was substituted with a 2-imidazolylethylamine (Fig. 19), this showed a reduction in the IGF-1R inhibitory activity (62). Substitution at the C-5 position of the benzimidazole core, either with a methyl group or hydrogen, showed a better inhibitory activity with respect to the bromo or methoxy groups (63). The pyridyl methylamine analogue (64c) displayed a 390 nM IGF-1R inhibitory activity and a secondary amine nitrogen on the side chain with the thiophene (64b) and furan (64d) analogues were totally devoid of any IGF-1R inhibitory activity.
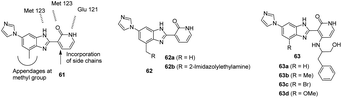 |
| Fig. 19 Representative benzimidazole as IGF-1R inhibitors. | |
Velaparthi et al. introduced electron-donating and electron-withdrawing meta-substituents, which indicated that halogen or hydroxyl substitution are preferred (65a–c).68a The potency of the hydroxyl analogue (65c), may be the result of a hydrogen-bond in the active site (Fig. 20). The modification at the pyridone with a phenethylamine group and the benzimidazole moieties with alkyl piperazine (49) showed an improvement in activity against the insulin-like growth factor-1 receptor, in the whole series of benzimidazole derivatives.
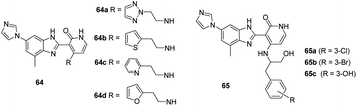 |
| Fig. 20 Effect of substituted pyridine on benzimidazole. | |
3.6. Interleukin-2 inducible T-cell kinase (ITK) inhibitors
Interleukin-2 inducible T-cell kinase (ITK) plays an important role in T-cell activation and proliferation70 and the initiation of regulatory and cytolytic effector functions. Compounds 66 and 67 have IC50 values in the range of 12 nM and exhibit good selectivity against a panel of protein kinase and are non-toxic.
Substitution with a 2-methoxyethyl group (68d) resulted in a 4-fold loss in potency, while the removal of the methyl group showed no effect. Large hydrophobic groups were preferred at this position; the only replacement that afforded a slight increase in potency was the introduction of a cyclopentylmethyl (68c) in the place of cyclohexyl (68a) and the replacement of N-methylcyclohexyl group with a morpholine (Fig. 21) resulted in a slight loss in potency, compared to 67.71
 |
| Fig. 21 Importance of N–H group on C-5 for ITK inhibitory activity. | |
Cook and co-workers72 have reported that the acylation of 2-aminobenzimidazoles resulted in good potency (IC50 = 180 nM) and moderate cellular activity (IC50 = 2.3 μM) and also tested for selectivity against insulin receptor tyrosine kinase, an enzyme with close structural homology to ITK. Small substituents at the 4-position of the benzimidazole increased both the potency and selectivity compared to larger groups. As a phenyl ring is not tolerated at the 4-position (69a),73 the potency of 69g suggested that substitution at the 3-position may avoid the unfavorable Phe435 interaction; the 3-substituted phenyl analogue showed a significant increase in potency. The 2-substituted thiophene analogue (70) was found to be a potent ITK inhibitor and served as a good replacement for the phenyl group, resulting in increased potency. Substitution at the 5-position of the thiophene ring has been found to increase the potency dramatically. A simple halogen substitution (70a–b)74 increased the molecular and cellular potency, cyano substitution at the 5-position also enhanced the potency, which increased by 250-fold selectivity for ITK (70c). Aryl substitution (70d–g) has been tolerated at the 5-position, and increased molecular and cellular potency. The introduction of the 2-pyridyl motif (70e) did not result in a substantial increase in potency, as compared to the phenyl analogue (70d). However, both the 3- and 4-pyridyl analogues, 70f and 70g, respectively, showed much improved molecular potency and these compounds have displayed very good selectivity against ITK.73 An oxazole analogue has shown good potency when assayed against ITK, with 5-oxazole being the most potent. Oxazoles at the 2 and 4-positions of thiophene have displayed reduced activity, similar to the 2-pyridyl analogue 70e, this loss of potency of the saturated compounds was attributed due to a lack of π-stacking interaction with Phe435, or an unfavorable electro-static repulsion between Lys391 and the protonated basic amine (or oxygen heteroatom) in the saturated ring system (Fig. 22).
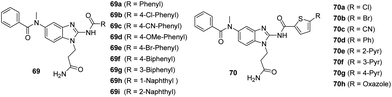 |
| Fig. 22 Aryl and 5-thienyl substitution on benzimidazole. | |
A pyrazole analogue has been shown to be the most potent compound, with an IC50 value of 2 nM, that was 25-fold more potent than the 5-oxazole analogue. Both the pyrazole and pyrazole derivative (71)75 have shown a greater than 1000-fold selectivity over ITK.
An unsubstituted amine led to an inactive compound, despite having a good binding potency. The introduction of one methyl group, as in compound 71d, was not sufficient for potency, but the N,N-dimethyl amine (71e) demonstrated moderate potency. Increasing the lipophilicity also increased the potency in both the cellular and functional assays. Aromatic substituents such as benzyl (71f) and aliphatic substituents are well tolerated. Polar substituents such as a hydroxy and methoxy group, or basic amines, such as dimethylamino substituents (Fig. 23), lead to a loss in potency.
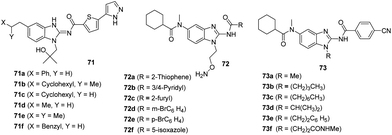 |
| Fig. 23 Effects of thiophene replacements and C-5 substituents on ITK inhibition. | |
Moriarty76 and co-workers have replaced the 2-thiophene with other heterocyclic moieties, viz. 2-furyl, 5-isoxazole, 3-pyridyl, 4-pyridyl or meta and para substituted phenyl, resulting in an improvement in the activity against ITK (72). The substitution of N-1 with linear alkyl groups led to a 17-fold improvement in potency, from a methyl (73a; IC50 = 0.27 μM) to butyl (73b; IC50 = 0.016 μM), but the extension of the chain to heptyl (73c; IC50 = 4.5 μM) resulted in a 17-fold loss in activity, indicating the optimum chain length required for the activity. The replacement of the N-alkyl group with a phenethyl group, (73e; IC50 = 0.006 μM), led to an additional improvement in potency, but the substitution of the phenyl ring was not tolerated and led to a loss in activity. Shortening the spacer length between the N-1 of the benzimidazole and the side chain primary amide resulted in an 18-fold loss in potency, while mono and di-substitution of the amide group resulted in a small loss in potency (2- to 4-fold). The replacement of a methyl group (74a; IC50 = 0.004 μM) with an iso-propyl group (74c; IC50 = 0.153 μM) resulted in a 38-fold loss in activity, but an ethyl group (74b; IC50 = 0.007 μM) did not negatively impact on the enzymatic activity. By replacing the cyclohexyl ring with methyl (75a; IC50 = 0.059 μM), ethyl (75b; IC50 = 0.32 μM), iso-propyl (75c; IC50 = 0.22 μM) or cyclopropyl (75d; IC50 = 0.046 μM) groups, this resulted in a loss in potency, but a cyclobutyl ring (75e; IC50 = 0.008 μM) gave potency comparable to 75a. Substituting the cyclohexyl ring with a phenyl group (75f; IC50 = 0.020 μM) resulted in 5-fold less activity, while removal of the carboxy cyclohexyl group (75g; IC50 = 0.076 μM) led to a 19-fold decrease in potency, relative to compound 74a. Interestingly, the replacement of the amide N with a C–H group (75h; IC50 = 0.003 μM) did not attenuate the activity. Phenyl (76b) could take the place of thiophene (76a; ITK IC50 (μM) = 0.37) with only a slight loss in potency. Electron withdrawing groups at C3 or C4 of the phenyl ring have been well tolerated; the 4-nitro analogue showing a fourfold improvement, while electron donating substituents, such as a methoxy group, decreased the activity (Fig. 24). An amide substitution at the 5-position of the benzimidazole has the requirement of a tertiary amide, with one cyclic substituent and one small alkyl substituent. The methyl can be replaced with small alkyl groups, but not with more bulky groups such as a second cyclohexyl ring, that reduced its potency.76
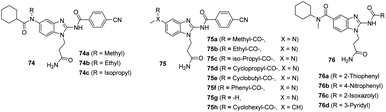 |
| Fig. 24 Variation of N-1 and C-5 substitution. | |
The cyclohexyl ring could be replaced with a phenyl ring, but the potency was lost on replacing it with smaller alkyl groups. While the unsubstituted benzimidazole is only weakly active, polar substituents, either as the amide or alcohol are preferred, as in 77a and 77b, showing the best potency. The substitution of the amide maintains the binding, but diminishes the cellular activity. Replacing the hydroxyl with an amine (Fig. 25) resulted in a 10-fold decrease in the enzyme activity, and the loss of cellular activity. Among this series of compounds, 70g or 70h, with pyridyl and oxazole groups are the most active.
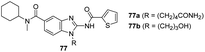 |
| Fig. 25 Effect of N-1 substituents on ITK inhibition. | |
3.7. Tie kinase inhibitors
The precise role of the Tie-2 receptor in pathological angiogenesis77 has been difficult to ascertain, due to the existence of numerous endogenous ligands, including angiopoeitin-1 (Ang-1) and angiopoeitin-2 (Ang-2).78 Structural modification of an existing kinase inhibitor series provided a pyridyl-pyrimidine derivative that is a potent, nonselective inhibitor of Tie-2.79
The monomethyl-substituted analogue 78a has an IC50 value of 23 nM, with a 10-fold higher IC50 value of 250 nM for inhibition. The desmethyl analogue (78b), monomethyl (78c), and dimethyl analogue (78d),80 all exhibited 10- to 20-fold reduced potency for Tie-2 inhibition. Naphthalene is an ideal central ring (79), exhibiting an IC50 value of 1 nM for the Tie-2 enzyme in cells, in this series of compounds.81 Lipophilic substituents (Me, CF3) in 79b–c at the 5 and 6-positions of benzimidazole (Fig. 26) reduced the Tie-2 potency.
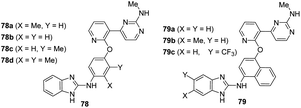 |
| Fig. 26 Selective Tie-2 inhibitor | |
3.8. Glycogen synthase kinase inhibitors
Glycogen synthase kinase 3 (GSK3), multi-functional serine–threonine kinases, are associated with many diseases, such as diabetes mellitus, cancer, bipolar disorder, Alzheimer's disease, and schizophrenia82 and participate in a multitude of cellular processes, including cell membrane-to-nucleus signaling, gene transcription, translation, cytoskeletal structuring and cell cycle progression.83 The introduction of relatively small substituents to the amide group (80) provides low inhibitory activities and the introduction of bulky aromatic moieties improved the inhibitory potency. Ro and co-workers84 have reported the substitution at the 4-position of benzimidazole. A docking study85 revealed that the oxygen atom of a phenethyl sulfonamide moiety, that is linked to the amide at the 4-position of the benzimidazole, would enhance the inhibitory activity by a hydrophilic interaction with Arg141. The introduction of relatively small substituents at the C-2 position (81) provided low inhibitory activities,86 while bulky aromatic moieties improved the inhibitory potency (Fig. 27). Thus, the combination of both a p-fluorophenyl at the 2-position and a phenethyl sulfonamide at the 4-position of benzimidazole (81c) makes the molecule with most activity. Compound 82a has been evaluated at a 5 μM concentration with 86% inhibition. As the size of the substituent on the amide increases, the potency of the inhibitor also improved (82b–d).87
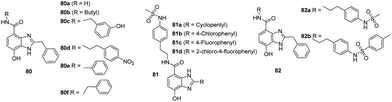 |
| Fig. 27 7-Hydroxy benzimidazole analogs against GSK3b. | |
3.9. Lymphocyte specific kinase inhibitors
Lymphocyte-specific kinase (Lck) is an attractive drug target, because of restricted expression in T cells and natural killer (NK) cells.88 Sabat89 and co-workers have reported the substitution of a pyrimidine moiety of 1-benzimidazole, with a methyl ether (83b) and amide (83d), displaying greatly attenuated activity. Pyrimidine compounds that did not contain the 4-methyl group on the C-2 anilino substituent were devoid of any activity. The presence of both a 4-methyl and a phenolic hydroxyl on the C-2 anilino substituent is crucial for good activity, compared to the lead compound 83a. The introduction of an ethylmorpholino group at the C6 with an O (84b) or NH (84c) linker resulted in better potency in the enzyme assay as inhibitors. The N-methyl piperazine analogue of benzimidazole displayed better potency, but possessed no aqueous solubility. The substitution of the N-methyl piperazine unit for morpholine gave the first analogue with good aqueous solubility, with a slight decrease in activity (84a; Lck IC50 = 65 nM). The insertion of a methylene spacer produced an analogue with better enzyme potency than the hydrazino analogue, but an additional ethylene spacer in combination with a piperidine substituent reduced the activity (Fig. 28).
 |
| Fig. 28 Effect of substituted pyrimidine on benzimidazole. | |
In the dihydropyrimido[4,5-d]pyrimidinone scaffold,90 the pyrimidine N1 of compound 85 does not form any hydrogen bonds and can be replaced with a carbon, without the loss of protein kinase inhibitory activity. So, in compound 86, where the central ring is replaced with a pseudo-ring constrained by an intramolecular hydrogen bond between the pyrimidine N and the benzimidazole NH group (Fig. 29), that allows the retention of the bidentate hinge hydrogen bonds and is also expected to position the phenyl ring 2 of compound 86 into the kinase specificity pocket,91 as in 85.
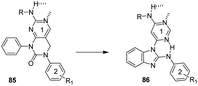 |
| Fig. 29 Rational design of pyrimidine-benzimidazoles. | |
Compounds 88 and 90a–b have reduced potency, as compared with compound 87b, because they all lack the hydrogen-bond-donating NH2 group required for strong Lck hinge binding and compound 88 is less potent than compound 89a. The electron withdrawing effect of the chloro group makes its adjacent pyrimidine nitrogen less basic and weakens the hydrogen bonding of that nitrogen with the Lck hinge region.92 The amino group in 89c made the N1 more basic than that in 87b and is expected to form stronger hydrogen bonds and therefore have a better inhibitory activity on Lck. However, compound 89c has shown much weaker potency, compared to compound 89b. From the binding mode, the substitution on the amino group of the pyrimidine has been exposed to solvent and is well-tolerated; a relatively larger group introduced a steric clash and also explained why compound 89b had even weaker inhibition, compared to compound 89a.93 Compound 89d is about twice as potent as compound 89e, suggesting that the solubility-enhancing morpholine group is better positioned (meta relative to the NH group). Pyrimidine benzimidazole compounds have shown strong inhibition of Lck in the biochemical and cellular assays. Large hydrophobic substituents, for example, t-butyl (90b), have been well tolerated in the ‘DFG out’ binding pocket (Fig. 30).
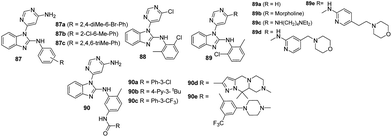 |
| Fig. 30 C-2 and N-4 substituted pyrimidine-benzimidazole analogs. | |
The Lck inhibition potency improved with an increase in the size of the substituted amides 90a, 90d–e and compound 90c has shown very potent Lck inhibition, in both the biochemical and cellular assays and also displayed a 40-fold selectivity against Src and a 4-fold selectivity against Hck in the biochemical (Lance) assay. Compounds 91a–b showed inactivity, or had very weak Lck inhibition, because they lack the hydrogen bond-donating NH group at the 6-position of the pyrimidine, which is important for binding to the Lck hinge region. Hydrophobic substitutions are preferred for inhibitory activity (92), but hydrophilic substituents lose Lck inhibitory activity, significantly. Compounds with an X
NHCH2CH2CN showed very weak Lck inhibition, while 93 showed improved Lck inhibitory activity, suggesting that the basic amine is very important for Lck inhibition (Fig. 31).
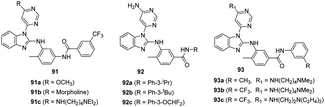 |
| Fig. 31 Effect of C-2 substitution on Lck activity of pyrimidines containing analogs. | |
Analogue 94 was highly potent against Lck in vitro and moderately active in the cellular assay. The optimization of the benzimidazole substructure of compound 95 resulted in the C5-substituted inhibitor 96, which showed potent inhibition of Lck activity.94 The diminished activities of the enantiomeric compound 98b, as well as that of the des-methyl analogue 98c underscores the importance of the benzylic methyl substitution. As expected, the addition of the methyl group (99b), resulted in a marked increase in activity. The substitution of the Cbz group with methylation or benzylation, resulted in la oss of activity.95 The phenyl carbamate (100) has shown fourfold less potency compared to compound 99, while the phenylacetamide lost much of its potency (Fig. 32).
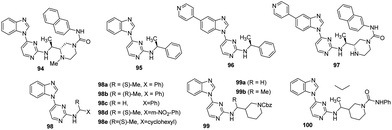 |
| Fig. 32 Effect of N-1 substitution on Lck activity of pyrimidines containing analogs. | |
The piperazine analogue (101b) has shown a tenfold increase in potency compared to the corresponding des-methyl piperidine naphthyl urea (101c).96 The introduction of the potency-enhancing methyl group into compound 101b provided compound 102a, which showed higher activity. The replacement of the methyl group in compound 103a with an ethyl group, 103b, did not significantly change the potency, but larger alkyl groups, such as iso-butyl or hexyl, led to significantly weaker inhibitors of IL2 release (Fig. 33). Other changes to this group, such as with a pyridyl, ethyl ester, and acetyl substituents was also detrimental to the inhibitor potency and cellular activity, although the piperazine (103a) was the most potent inhibitor of Lck activity in this series (IC50 = 0.06 nM). When these benzimidazole C5 substituents were incorporated into the piperazine naphthyl urea series (104), the resulting compounds were highly potent inhibitors of Lck activity. Thus, two pyrimidine rings on the benzimidazole moiety (103), showed more interactions for lymphocyte specific inhibitory properties.
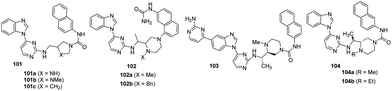 |
| Fig. 33 N-1 substituted pyrimidines containing analogs. | |
3.10. RAF kinase inhibitors
The many effects of rapidly growing fibrosarcoma (RAF) kinases on cancer cell growth and survival, together with the high prevalence of mutation in melanoma, for which there is no good treatment, make RAF a very attractive target for anticancer therapy.97
A comparison of derivatives 105a–e, bearing different residues at the 2-position of the benzimidazole moiety, revealed that an acetyl amino group provided the best activity. This residue is twice as potent than the methyl amide residue, and almost five times more potent than the methyl carbamate. The potency of the compounds was significantly improved by the introduction of additional substituents in other positions, such as a chloro or methoxy group on the phenylurea at the 6-position of the benzimidazole (Fig. 34). A CF3 group is better than a chloro, but in combination with a methoxy group, potency could be regained; the lower activity of all these derivatives confirmed the need for electron withdrawing residues or an aromatic ring.98
 |
| Fig. 34 Benzimidazole derivatives as c-RAF inhibitory activities. | |
Ramurthy and co-workers have introduced a t-butylphenyl group on the C-2 position of the benzimidazole (106), that make it the most active compound for RAF kinase inhibitors. A 3-tert-butyl group is important for in vitro potency and inhibition of the phosphorylation of ERK.99
3.11. IKK kinase inhibitors
Benzimidazole has been identified as a moderately potent inhibitor of IKK (inhibitor of nuclear factor Kappa kinase) and has potential to interfere with several stages of mitosis and therefore presents an intervention opportunity in the oncology arena.100
In compounds 108a–b and 109a–b,101 the replacement of the amide moiety of compound 107 with a nitrile group is detrimental to the PLK1 activity, but is beneficial to the inhibitory effect upon IKK-b. The dimethoxy substituents at the 5 and 6 positions of the benzimidazole ring point towards the solvent at the edge of the ATP site. The benzimidazole also makes hydrophobic contacts with Ala31 and Leu134. The o-Cl atom of compound 110 makes hydrophobic contacts with Val18 and Gly11 on the N-terminal lobe, but the opposite edge of the phenyl ring is in the region of the backbone carbonyl of Gln131, in the C-terminal lobe, and makes no specific interactions. The primary carboxamide substituent on the thiophene ring points toward the back pocket of the active site, where it makes a direct hydrogen bond with Glu51, via an NH2. However, the carbonyl of the carboxamide lacks any hydrogen-bonding partner (Fig. 35) but carboxylic acids can be tolerated in the position of the amide in PLK1 (111), because the ionizable histidine provides a counterion to the charge.
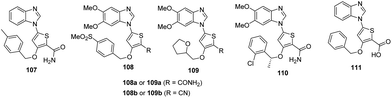 |
| Fig. 35 Benzimidazole bearing N1 thienyl analogs. | |
3.12. Rho kinase inhibitors
Substituted benzimidazoles act as ATP-competitive Rho kinase (ROCK) inhibitors that have excellent potency in both the biochemical (IC50 < 10 nM) and cell-based (IC50 < 100 nM) assays. Their high potency is largely due to three key interactions within the ROCK binding pocket: (i) H-bonding between the pyridyl nitrogen and hinge region, (ii) water-mediated H-bonding of an imidazole nitrogen and L121, and (iii) hydrophobic interactions between the aryl moiety and the P-loop (112). The addition of a polar side chain improved selectivity for ROCK, that can be attributed to the interaction of the polar side chain with the ROCK binding pocket.102 The incorporation of a substituent at the 6- or 7-position of the benzimidazole reduced the affinity for ROCK. The lack of tolerance for even small groups on these positions indicated that this portion of the inhibitor makes close contact with the enzyme.103 Larger alkoxy groups might improve the selectivity (Fig. 36), but a methoxy group (113d) was well tolerated, relative to 113b.104 Overall, no improvement in the desired selectivity was found by adding substituents on any of the three benzimidazole carbon atoms. A methyl group at N-1 was poorly tolerated, while the N-3 methyl derivative was well tolerated, exhibiting only a small decrease in the affinity for ROCK. The tolerance of fairly large groups indicated that groups on the N-3 were likely projected into the vacant, solvent exposed portion of the binding pocket in ROCK.
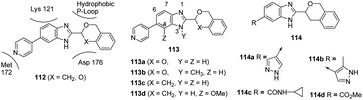 |
| Fig. 36 Effects of pyridine and imidazole substitution at benzimidazole analogs. | |
The 5-position appears to be solvent exposed and showed a greater tolerance toward substitution. Increasing the size of the alkoxy substituent led only to a large decrease in the ROCK potency. The addition of a substituted side chain on the benzimidazole core might lead to an improvement in the selectivity for ROCK. A pyrazole at the 5-position of the benzimidazole (114a)105 gives high ROCK affinity (IC50 = 27 nM) and cell based potency (IC50 = 86 nM), but with a reduced selectivity for PKA (IC50 = 168 nM). The addition of a methyl group on the 3-position of the pyrazole modestly improved the ROCK-II potency. The addition of a methoxy or an amide group improved both the potency and PKA selectivity, because these groups are helpful in enhancing the affinity for ROCK II. Cyclopropylamide (114c)106 or methyl ester groups (114d) were well tolerated, with an increase in the activity for ROCK-II. The primary amide (115a)105 was similar in potency and selectivity to the acetylchromene (115b), but with lower potency than the secondary and tertiary amides. Larger cycloalkyl amides (115c) possessed excellent ROCK potency, but the PKA potency was greatly increased compared to the smaller cyclopropyl group (115d). Benzimidazole with a fluorine atom at the 7-position (116) showed excellent affinity for ROCK-II (Fig. 37) and high potency in the cell-based assay (IC50 < 6 nM). A methoxychromene at the 2-position of benzimidazole (116) precisely fits into the hydrophobic pocket, for the interaction with kinase inhibitor.
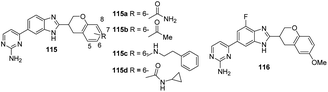 |
| Fig. 37 Evaluation of benzimidazole bearing 6-substituted chromans. | |
3.13. Other tyrosine kinase inhibitors
Tyrosine kinase controls many cellular processes through the phosphorylation and dephosphorylation of intracellular proteins and its signaling transduction pathways107 that are associated with diabetes, rheumatoid arthritis, psoriasis and cancer.108 A 2 to15-fold increase in kinase activity was seen on the incorporation of electron withdrawing substituents in the 6-position of the benzimidazole, with the 6-cyano group (117) exhibiting an IC50 value of 7 nM in the kinase assay. Halogenated substituents (118) also provided compounds with good kinase inhibitory activity. Difluorophenyl provided an increase in potency over the dichlorophenyl analogue, with the 2,6-difluorobenzyl analogue (Fig. 38) displaying an IC50 value of 6 nM (119a–b).109
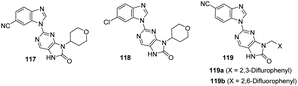 |
| Fig. 38 Purinone-benzimidazole based tyrosine inhibitors. | |
Fluorination at the 5-, 6- and 8-positions of the chroman showed a slight increase in inhibitory activity, whereas the 7-fluorochroman analogue showed an approximately threefold weaker activity. As with the substituted benzylic analogue, chlorine substitution was found to be inferior to both a fluorine and hydrogen (120). A significant increase in potency was observed for the chloro, fluoro and cyano-substituted benzimidazoles. The 6-fluorobenzimidazole analogue demonstrated good JAK3 kinase activity and a nine-fold selectivity over JAK,2 in the kinase assay.
Hamaguchi and co-workers have reported that substitution at the 5-position of benzimidazole with a 2-sulfonyl group is required for an inhibitory effect. With a change from the 4-methyl-thiopyridyl moiety (121a) to a 4-methylpyridyl (121b) or pyridyl moiety (121c), the inhibitory effects were decreased (Fig. 39), which makes this moiety one of the most active kinase inhibitors in this series of compounds.110
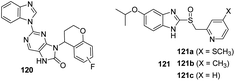 |
| Fig. 39 Chroman-benzimidazole based tyrosine inhibitors. | |
3.14. Casein kinase-2 and analogue inhibitors
Inhibitors of casein kinase-2 (CK2) trigger apoptosis and increase the susceptibility of cancer cells to chemotherapeutic agents111 and its activity is aberrantly elevated in various tumor types,112 like breast carcinoma, adenocarcinoma of the lung, prostate carcinoma and lymphomas. The CK2 inhibitor, 4,5,6,7-tetrabromo-1H-benzimidazole (122) has been used to elucidate the role of CK2 phosphorylation.113 More potent inhibitors of CK2 are obtained by a modification of 122 and selectivity is provided by the hydrophobic pocket adjacent to the ATP/GTP binding site, which is smaller in CK2 than in other protein kinases.114 Polyhalogenated benzimidazoles have been found to represent a valuable scaffold to effectively compete with the ATP binding site (123).115 The four atoms of bromine in the benzene ring seem to be an essential requirement for the biological activity.116 Four chlorine atoms, methyl groups or a change of the length of the alkyl substituents led to a significantly lower inhibitory effect on the CK2 activity. The introduction of an S-carboxymethyl, S-carboxyethyl or S-carboxypropyl residue (Fig. 40) at position 2 of 124 resulted in an increase in the inhibitory activity against CK2, from 8-fold to 11-fold.117 Wesley and co-workers have reduced the ketone group of mebendazole (125) to a hydroxyl group (126), but this leads to a loss of inhibition towards the parasite CDPK1 enzymes.118 The removal of the carbamate moiety (127) or the addition of a urea (128) or amides (129) at the 2-position of the benzimidazole retains the inhibitory potency, indicating that the carbonyl function of the carbamate is not critical for inhibition (Fig. 41).
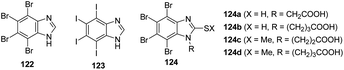 |
| Fig. 40 Structures of polyhalogenated benzimidazoles. | |
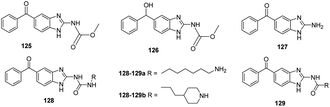 |
| Fig. 41 C-2 modified analogs of benzimidazole as casein kinase inhibitors. | |
Aromatic substitution at the N-1 position of the benzimidazole showed a strong relationship to the binding affinity. For example, ortho-aromatic substituents have approximately 20-fold more potency than the meta or para-substituted systems. Directly attached cycloalkyl groups, like the N-cyclobutyl (130d), N-cyclopentyl (130c) and N-cyclohexyl (130b) compounds exhibited <20 nM inhibition. More polar substituents generally showed a significantly weaker binding affinity.119 Small polar substituents at the 5-position of the benzimidazole fared the best, with the hydroxyl (131b) and amino (131c) groups being below the limit of detection (Fig. 42). 6-Phenyl-3-benzimidazole (132) has been found to be especially active against PKC-ζ (IC50 = 22 nM) and CDK-2 (IC50 = 102 nM). The addition of the 3-OMe (133a) moiety to either the 4-OH (133b) or the 4-NH2 (133c), resulted in a 4-fold loss of potency against PKC.
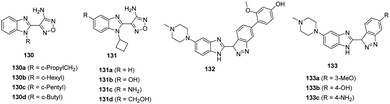 |
| Fig. 42 Activity of N-substituted oxadiazolamine and indazole analogs. | |
4 Conclusion
This review has given an overview of benzimidazole derivatives that have been developed to date as protein kinase enzyme inhibitors for anticancer agents. As can be seen from this review, the field is thriving and more examples are being published every year, with many more being developed by both academic institutions and industry. The benzimidazole structure has a bright future in the field of small molecules, targeting therapeutics; its ease of synthesis and the ability to adapt and modify the basic structure is the key to its success. The progress of the benzimidazole derivatives has resulted in the appearance of new potent kinase inhibitors. The accumulative evidence has suggested that the kinase pathway is widely involved in the pathogenesis of a variety of diseases and that benzimidazole derivatives are useful for the kinase treatment of those diseases. However, few benzimidazole kinase inhibitors are available for clinical use, possibly because of kinase's wide distribution and various substrates, which may cause more untoward effects. Many reports also show that benzimidazole could be a potential target for the treatment of some diseases, such as anticancer, antimicrobial, antiulcer, antimalarial etc. Further studies should aim to develop selective inhibitors to reduce any adverse effects. Moreover, the crystal and the co-crystal structures of various kinases bound with inhibitors have been solved, paving a way for the development of inhibitors by virtual screening, another attractive strategy for developing new drugs with a higher efficiency over traditional ways. The activity profile of the benzimidazole scaffold relies upon the substitution on the parent skeleton and n indicated pharmacophore can be synthesized, based on the SAR analysis for further exploration of the biological efficacy.
In summary, given that kinases distribute in many tissues and also have multiple functions, more specific benzimidazole based kinase inhibitors need to be developed. We hope that novel and better kinase inhibitor(s), by generating suitable hybrids of benzimidazole and heterocyclic nuclei will be developed for diseases in the short future.
Acknowledgements
KP thanks the SERC Fast Track Research Grant, Department of Science and Technology, New Delhi (SR/FT/CS-40/2010). PS is grateful for a DST/Inspired Fellowship (Fellow Code-IF110542).
References and notes
- A. Y. Louie and T. J. Meade, Chem. Rev., 1999, 99, 2711 CrossRef CAS PubMed.
- A. G. Gorp, K. M. Pomeranz, K. U. Birkenkamp, R. C. Hui, E. W. Lam and P. J. Coffer, Cancer Res., 2006, 66, 10760 CrossRef PubMed.
- G. Burnett and E. P. Kennedy, J. Biol. Chem., 1954, 211, 969 CAS.
- M. J. O'Neil, M. Smith and P. E. Heckelman, The Merck Index, Merck & Co Inc, 13th edn, 2001, 10074, p. 1785 Search PubMed.
- J. R. Kumar, J. L. Jawahar and D. P. Pathak, Eur. J. Chem., 2006, 3, 278 CAS.
-
(a) K. C. Nicolaou, A. J. Roecker, R. Hughes, R. van Summeren, J. A. Pfefferkorn and N. Winssinger, Bioorg. Med. Chem., 2003, 11, 465 CrossRef CAS;
(b) A. T. Mavrova, K. K. Anichina, D. I. Vuchev, J. A. Tsenov, M. S. Kondeva and M. K. Micheva, Bioorg. Med. Chem., 2005, 13, 5550 CrossRef CAS PubMed.
-
(a) M. M. Ramla, M. A. Omar, A. M. M. El-Khamry and H. I. El Diwani, Bioorg. Med. Chem., 2006, 14, 7324 CrossRef CAS PubMed;
(b) S. Demirayak, U. Abu Mohsen and A. C. Karaburun, Eur. J. Med. Chem., 2002, 37, 255 CrossRef CAS;
(c) A. W. White, N. J. Curtin, B. W. Eastman, B. T. Golding, Z. Hostomsky, S. Kyle, J. Li, K. Maegley, A. D. J. Skalitzky, S. E. Webber, X. H. Yu and R. J. Griffin, Bioorg. Med. Chem. Lett., 2004, 14, 2433 CrossRef CAS PubMed;
(d) E. Lukevics, P. Arsenyan, I. Shestakova, I. Domracheva, A. Nesterova and O. Pudova, Eur. J. Med. Chem., 2001, 36, 507 CrossRef CAS;
(e) A. Kamal, P. P. Kumar, K. Sreekanth, B. N. Seshadri and P. Ramulu, Bioorg. Med. Chem. Lett., 2008, 18, 2594 CrossRef CAS PubMed;
(f) S. T. Huang, I. J. Hsei and C. Chen, Bioorg. Med. Chem., 2006, 14, 6106 CrossRef CAS PubMed;
(g) B. Narasimhan, D. Sharma and P. Kumar, Med. Chem. Res., 2012, 21, 269 CrossRef CAS;
(h) A. W. White, R. Almassy, A. H. Calvert, N. J. Curtin, R. J. Griffin, Z. Hostomsky, K. Maegly, D. R. Newell, S. Srivinivasan and B. T. Golding, J. Med. Chem., 2000, 43, 4084 CrossRef CAS PubMed.
-
(a) H. Al-Muhaimeed, J. Int. Med. Res., 1997, 25, 175 CAS;
(b) P. Madsen, L. B. Knudsen, F. C. Wiberg and R. D. Carr, J. Med. Chem., 1998, 41, 5150 CrossRef CAS PubMed;
(c) B. D. Palmer, A. J. Kraker, B. G. Hartl, A. D. Panopoulos, R. L. Panek, B. L. Batley, G. H. Lu, S. Trumpp-Kallmeyer, H. D. Hollis Showalter and W. A. Denny, J. Med. Chem., 1999, 42, 2373 CrossRef CAS PubMed;
(d) M. Subhashish, K. Vijayendra, A. K. Prasad, R. Hanumanth, G. Raj, M. E. Bracke, E. O. Carl, C. J. Subash and S. P. Virender, Bioorg. Med. Chem., 2001, 9, 337 CrossRef;
(e) J. J. Vanden Eynde, F. Delfosse, P. Lor and Y. van Haverbeke, Tetrahedron, 1995, 51, 5813 CrossRef CAS;
(f) K. J. Lee and K. D. Janda, Can. J. Chem., 2001, 79, 1556 CrossRef CAS;
(g) E. A. Rashedy and H. Y. Aboul-Enein, Mini–Rev. Med. Chem., 2013, 13, 399 Search PubMed.
-
(a) Y. S. Chhonker, B. Veenu, S. R. Hasim, N. Kaushik, D. Kumar and P. Kumar, Eur. J. Chem., 2009, 6, 342 Search PubMed;
(b) R. Chebolu, D. N. Kommi, D. Kumar, N. Bollineni and A. K. Chakraborti, J. Org. Chem., 2012, 77, 10158 CrossRef CAS PubMed;
(c) R. Vinodkumar, S. D. Vaidya, B. V. S. Kumar, U. N. Bhise, S. B. Bhirud and U. C. Mashelkar, ARKIVOC, 2008(xiv), 37 Search PubMed;
(d) P. Bandyopadhyay, M. Sathe, S. Ponmariappan, A. Sharma, P. Sharma, A. K. Srivastava and M. P. Kaushik, Bioorg. Med. Chem. Lett., 2011, 21, 7306 CrossRef CAS PubMed;
(e) K. Bahrami, M. M. Khodaei and F. Naali, J. Org. Chem., 2008, 73, 6835 CrossRef CAS PubMed;
(f) Y. Kim, M. R. Kumar, N. Park, Y. Heo and S. Lee, J. Org. Chem., 2011, 76, 9577 CrossRef CAS PubMed;
(g) L. H. Du and Y. G. Wang, Synthesis, 2007, 5, 675–678 Search PubMed;
(h) M. Breza and V. Milata, ARKIVOC, 2005(ix), 80 Search PubMed;
(i) Z. G. Le, Z. C. Chen, Y. Hu and Q. G. Zheng, Synthesis, 2004, 2, 208 Search PubMed.
- R. S. Joshi, P. G. Mandhane, S. K. Dabhade and C. H. Gill, J. Chin. Chem. Soc., 2010, 57, 1227 CAS.
- H. Yun, J. Yang, B. Wu, D. Robinson, K. Sprankle, P. P. Kung, K. Lowery, V. Mohan, S. Hofstadler, E. E. Swayze and R. Griffey, Bioorg. Med. Chem. Lett., 2004, 14, 695 CrossRef PubMed.
-
(a) A. Grassi, J. Ippen, M. Bruno, G. Thomas and P. Bay, Eur. J. Pharmacol., 1991, 195, 251 CrossRef CAS;
(b) Y. Ozkay, Y. Tunali, H. Karaca and I. Isikdag, Eur. J. Med. Chem., 2010, 45, 3293 CrossRef PubMed.
- M. Tonelli, M. Simone, B. Tasso, F. Novelli, V. Boido, F. Sparatore, G. Paglietti, S. Pricl, G. Giliberti, S. Blois, C. Ibba, G. Sanna, R. Loddo and P. La Colla, Bioorg. Med. Chem., 2010, 18, 2937 CrossRef CAS PubMed.
- Z. Kazimierczuk, J. A. Upcroft, P. Upcroft, A. Górska, B. Starooeciak and A. Laudy, Acta Biochim. Pol., 2002, 49, 185 CAS.
- Y. Tamura, N. Omori, N. Kouyama, Y. Nishiura, K. Hayashi, T. Watanabe, Y. Tanaka, T. Chiba, H. Yukioka, H. Sato and T. Okuno, Bioorg. Med. Chem. Lett., 2012, 22, 5498 CrossRef CAS PubMed.
- X. J. Wang, N. Y. Chu, Q. H. Wang, C. Liu, C. G. Jiang, X. Y. Wang, T. Ikejima and M. S. Cheng, Bioorg. Med. Chem. Lett., 2012, 22, 6297 CrossRef CAS PubMed.
-
(a) L. L. Brunton, J. S. Lazo and K. L. Parker, Goodman & Gilman's. The Pharmacological Basis of Therapeutics, Mc Graw-Hill, New York, 11th edn, 2006 Search PubMed;
(b) N. A. Meanwell and M. Krystal, Drugs Future, 2007, 32, 441 CrossRef CAS.
- Q. Yong, Y. M. Gao, Y. Ying, C. Kui, Z. Z. Qing, J. M. Wen, S. Lei and Z. Jing, Bioorg. Med. Chem., 2010, 18, 4310 CrossRef PubMed.
- A. Todorovic, C. G. Joseph, N. B. Sorensen, M. S. Wood and C. Haskell-Luevano, Chem. Biol. Drug Des., 2007, 69, 338 CAS.
- A. W. White, N. J. Curtin, B. W. Eastman, B. T. Golding, Z. Hostomsky, S. Kyle, J. Li, K. A. Maegely, D. J. Skalitzy, S. E. Webber, X. H. Yu and R. J. Griffin, Bioorg. Med. Chem. Lett., 2004, 14, 2433 CrossRef CAS PubMed.
- A. R. Mackenzie, A. P. Marchington, D. S. Middleton, S. D. Newman, C. N. Selway and N. K. Terrett, Bioorg. Med. Chem. Lett., 2003, 13, 2211 CrossRef CAS.
-
(a) A. A. Selcen, Z. Sevil, Z. Istvan, C. Gunes, R. Borbala, G. H. Semih and T. Zeki, J. Enzyme Inhib. Med. Chem., 2009, 24, 844 CrossRef PubMed;
(b) S. Alper, O. Arpaci, T. E. S. Aki and I. Yalcin, Farmaco, 2003, 58, 497 CrossRef CAS;
(c) A. A. Selcen, Z. Sevil, Z. Istvan, C. Gunes, R. Borbala, G. H. Semih and T. Zeki, Med. Chem., 2009, 24, 844 Search PubMed.
- S. Hirashima, T. Suzuki, T. Ishida, S. Noji, S. Yata, I. Ando, M. Komatsu, S. Ikeda and H. Hashimoto, J. Med. Chem., 2006, 49, 4721 CrossRef CAS PubMed.
- A. W. Denny, G. W. Rewcastle and B. C. Baguley, J. Med. Chem., 1990, 33, 814 CrossRef.
- C. Heinemann, S. Schliemann-Willers, C. Oberthur, M. Hamburger and P. Elsner, Planta Med., 2004, 70, 385 CrossRef CAS PubMed.
- T. Okuno, H. Takenaka, Y. Aoyama, Y. Kanda, Y. Yoshida, T. Okada, H. Hashizume, M. Sakagami, T. Nakatani, K. Hattori, T. Ichihashi, T. Yoshikawa, H. Yukioka, K. Hanasaki and Y. Kawanishi, Abstr. Pap., Jt. Conf. - Chem. Inst. Can. Am. Chem. Soc., 2010, 240, 284 Search PubMed.
-
(a) M. Carmena and W. C. Earnshaw, Nat. Rev. Mol. Cell Biol., 2003, 4, 842 CrossRef CAS PubMed;
(b) E. Hannak, M. Kirkham, A. A. Hyman and K. Oegema, J. Cell Biol., 2001, 155, 1109 CrossRef CAS PubMed;
(c) T. Sardon, I. Peset, B. Petrova and I. Vernos, EMBO J., 2008, 27, 2567 CrossRef CAS PubMed;
(d) H. Katayama, W. R. Brinkley and S. Sen, Cancer Metastasis Rev., 2003, 22, 451 CrossRef.
-
(a) J. R. Bischoff, L. Anderson, Y. Zhu, K. Mossie, L. Ng, B. Souza, B. Schryver, P. Flanagan, F. Clairvoyant, C. Ginther, C. S. M. Chan, M. Novotny, D. J. Slamon and G. D. Plowman, EMBO J., 1998, 17, 3052 CrossRef CAS PubMed;
(b) T. Tanaka, M. Kimura, K. Matsunaga, D. Fukada, H. Mori and Y. Okano, Cancer Res., 1999, 59, 2041 CAS;
(c) G. Mountzios, E. Terpos and M. A. Dimopoulos, Cancer Treat. Rev., 2008, 34, 175 CrossRef CAS PubMed;
(d) H. Katayama and S. Sen, Biochim. Biophys. Acta, Gene Regul. Mech., 2010, 1799, 829 CrossRef CAS PubMed.
- E. A. Harrington, D. Bebbington, J. Moore, R. K. Rasmussen, A. O. Ajose-Adeogun, T. Nakayama, J. A. Graham, C. Demur, T. Hercend, A. Diu-Hercend, M. Su, J. M. Golec and K. M. Miller, Nat. Med., 2004, 10, 262 CrossRef CAS PubMed.
-
(a) O. Gautschi, J. Heighway, P. C. Mack, P. R. Purnell, P. N. Lara and D. R. Gandara, Clin. Cancer Res., 2008, 14, 1639 CrossRef CAS PubMed;
(b) F. Girdler, K. E. Gascoigne, P. A. Eyers, S. Hartmuth, C. Crafter, K. M. Foote, N. J. Keen and S. S. Taylor, J. Cell Sci., 2006, 119, 3664 CrossRef CAS PubMed;
(c) S. Sen, H. Zhou and R. A. A. White, Oncogene, 1997, 14, 2195 CAS;
(d) S. D. Slattery, M. A. Mancini, B. R. Brinkley and R. M. Hall, Cell Cycle, 2009, 8, 2986 CrossRef CAS.
- Z. M. Nofal, E. A. Soliman, S. S. Abd El-Karim, M. I. El Zahar, A. M. Srour, S. Sethumadhavan and T. J. Maher, Acta Pol. Pharm., 2011, 68, 519 CAS.
-
(a) J. P. Arbitrario, B. J. Belmont, M. J. Evanchik, W. M. Flanagan, R. V. Fucini, S. K. Hansen, S. O. Harris, A. Hashash, U. Hoch, J. N. Hogan, A. R. Howlett, J. W. Jacobs, J. W. Lam, S. C. Ritchie, M. J. Romanowski, J. A. Silverman, D. E. Stockett, J. N. Teague, K. M. Zimmerman and P. Taverna, Cancer Chemother. Pharmacol., 2010, 65, 707 CrossRef CAS PubMed;
(b) P. Taverna, J. Hogan, J. Kumer, J. Arbitrario, U. Hoch, J. Silverman and A. Howlett, Ann. Oncol., 2007, 18, 204 Search PubMed.
-
(a) J. D. Oslob, S. A. Heumann, C. H. Yu, D. A. Allen, S. Baskaran, M. Bui, E. Delarosa, F. D. Fung, A. Hashash, J. Hau, S. Ivy, J. W. Jacobs, W. Lew, J. Maung, R. S. McDowell, S. Ritchie, M. J. Romanowski, J. A. Silverman, W. Yang, M. Zhong and T. Fuchs-Knotts, Bioorg. Med. Chem. Lett., 2009, 19, 1409 CrossRef CAS PubMed;
(b) J. D. Oslob, M. J. Romanowski, D. A. Allen, S. Baskaran, M. Bui, R. A. Elling, W. F. Flanagan, F. D. Fung, E. J. Hanan, S. Harris, S. A. Heumann, U. Hoch, J. W. Jacobs, J. Lam, C. E. Lawrence, R. S. McDowell, M. A. Nannini, W. Shen, J. A. Silverman, M. M. Sopko, B. T. Tangonan, J. Teague, J. C. Yoburn, C. H. Yu, M. Zhong, K. M. Zimmerman, T. O'Brien and W. Lew, Bioorg. Med. Chem. Lett., 2008, 18, 4880 CrossRef CAS PubMed.
- M. Zhong, M. Bui, W. Shen, S. Baskaran, D. A. Allen, R. A. Elling, W. M. Flanagan, A. D. Fung, E. J. Hanan, S. O. Harris, S. A. Heumann, U. Hoch, S. N. Ivy, J. W. Jacobs, S. Lam, S. Lee, R. S. McDowell, J. R. Oslob, H. E. Purkey, M. J. Romanowski, J. A. Silverman, B. A. Tangonan, P. Taverna, W. Yang, J. C. Yoburn, C. H. Yu, K. M. Zimmerman, T. O'Brien and W. Lew, Bioorg. Med. Chem. Lett., 2009, 19, 5158 CrossRef CAS PubMed.
- P. G. Wyatt, A. J. Woodhead, V. Berdini, J. A. Boulstridge, M. G. Carr, D. M. Cross, D. J. Davis, L. A. Devine, T. R. Early, R. E. Feltell, E. J. Lewis, R. L. McMenamin, E. F. Navarro, M. A. O'Brien, M. O'Reilly, M. Reule, G. Saxty, L. C. A. Seavers, D. M. Smith, M. S. Squires, G. Trewartha, M. T. Walker and A. Woolford, J. Med. Chem., 2008, 51, 4986 CrossRef CAS PubMed.
- S. Howard, V. Berdini, J. A. Boulstridge, M. G. Carr, D. M. Cross, J. Curry, L. A. Devine, T. R. Early, L. Fazal, A. L. Gill, M. Heathcote, S. Maman, J. E. Matthews, R. L. Mcmenamin, E. F. Navarro, M. A. O'Brein, D. C. Rees, M. Reule, D. Tisi, G. Williams, M. Vinkovic and P. G. Wyatt, J. Med. Chem., 2009, 52, 379 CrossRef CAS PubMed.
- A. J. Obaya and J. M. sedivy, Cell. Mol. Life Sci., 2002, 59, 126 CrossRef CAS.
-
(a) T. M. Sielecki, J. F. Boylan, P. A. Benfiled and G. T. Trainor, J. Med. Chem., 2000, 43, 1 CrossRef CAS;
(b) P. I. Toogood, Med. Res. Rev., 2001, 21, 487 CrossRef CAS PubMed;
(c) H. Hirai, N. Kawanishi and Y. Iwasawa, Curr. Top. Med. Chem., 2005, 5, 167 CrossRef CAS.
- S. Verma, D. Nagarathnam, J. Shao, L. Zhang, J. Zhao, L. Wang, T. Li, E. Mull, I. Enyedy, C. Wang, Q. Zhu, M. Altieri, J. Jordan, T. A. T. Dang and S. Reddy, Bioorg. Med. Chem. Lett., 2005, 15, 1973 CrossRef CAS PubMed.
- A. J. Bridges, Chem. Rev., 2001, 101, 2541 CrossRef CAS PubMed.
- R. A. Engh and D. Bossemeyer, Pharmacol. Ther., 2002, 93, 99 CrossRef CAS.
- S. M. Sondhi, N. Singh, A. Kumar, O. Lozachc and L. Meijerc, Bioorg. Med. Chem., 2006, 14, 3758 CrossRef CAS PubMed.
-
(a) S. Leclerc, M. Garnier, R. Hoessel, J. A. Marko, J. A. Bibb, G. L. Snyder, P. Greengard, J. Biernat, E. M. Mandelkow and G. Eisenbrand, J. Biol. Chem., 2001, 276, 251 CrossRef CAS PubMed;
(b) M. Leost, C. Schultz, A. Link, Y. Z. Wu, J. Biernat, E. M. Mandelkow, J. A. Bibb, G. L. Snyder, P. Greengard, D. W. Zaharevitz, R. Gussio, A. Senderovitz, E. A. Sausville, C. Kunick and L. Meijer, Eur. J. Biochem., 2000, 267, 5983 CrossRef CAS.
-
(a) S. Baumli, J. A. Endicott and L. N. Johnson, Chem. Biol., 2010, 17, 931 CrossRef CAS PubMed;
(b) S. Wang, C. Meades, G. Wood, A. Osnowski, S. Anderson, R. Yuill, M. Thomas, M. Mezna, W. Jackson, C. Midgley, G. Griffiths, I. Fleming, S. Green, I. McNae, S. Y. Wu, C. McInnes, D. Zheleva, M. D. Walkinshaw and P. M. Fischer, J. Med. Chem., 2004, 47, 1662 CrossRef CAS PubMed.
-
(a) S. Wang, G. Wood, C. Meades, G. Griffiths, C. Midgley, I. McNae, C. McInnes, S. Anderson, W. Jackson, M. Mezna, R. Yuill, M. Walkinshaw and P. M. Fischer, Bioorg. Med. Chem. Lett., 2004, 14, 4237 CrossRef CAS PubMed;
(b) X. Liu, S. Shi, C. Pepper, P. M. Fischer and S. Wang, Int. J. Cancer, 2012, 130, 1216 CrossRef CAS PubMed;
(c) S. Wang, C. A. Midgley, F. Scaerou, J. B. Grabarek, G. Griffiths, W. Jackson, G. Kontopidis, S. J. McClue, C. McInnes, C. Meades, M. Mezna, A. Plater, I. Stuart, M. P. Thomas, G. Wood, R. G. Clarke, D. G. Blake, D. I. Zheleva, D. P. Lane, R. C. Jackson, D. M. Glover and P. M. Fischer, J. Med. Chem., 2010, 53, 4367 CrossRef CAS PubMed.
-
(a) C. L. Moore, A. Zivkovic, J. W. Engels and R. D. Kuchta, Biochemistry, 2004, 43, 12367 CrossRef CAS PubMed;
(b) S. Wang, G. Griffiths, C. A. Midgley, A. L. Barnett, M. Cooper, J. Grabarek, L. Ingram, W. Jackson, G. Kontopidis, S. J. McClue, C. McInnes, J. McLachlan, C. Meades, M. Mezna, I. Stuart, M. P. Thomas, D. I. Zheleva, D. P. Lane, R. C. Jackson, D. M. Glover, D. G. Blake and P. M. Fischer, Chem. Biol., 2010, 17, 1111 CrossRef CAS PubMed;
(c) H. M. Alkahtani, A. Y. Abbas and S. Wang, Bioorg. Med. Chem. Lett., 2012, 22, 1317 CrossRef CAS PubMed.
- G. Navarrete-Vazquez, R. Cedillo, A. Hernandez-Campos, L. Yepez, F. Hernadez-Luis, J. Valdez, R. Morales, R. Cortes, M. Hernandez and R. Castillo, Bioorg. Med. Chem. Lett., 2001, 11, 187 CrossRef CAS.
-
(a) R. Lin, Y. Lu, S. K. Wetter, P. J. Connolly, I. J. Turchi, W. V. Murray, S. L. Emanuel, R. H. Gruninger, A. Fuentes-Pesquera, S. A. Middleton and L. K. Jolliffe, Bioorg. Med. Chem. Lett., 2005, 15, 2221 CrossRef CAS PubMed;
(b) R. Lin, S. K. Wetter, Y. Lu, P. J. Connolly, S. Emanuel, R. H. Gruninger and S. A. Middleton, PCT Int. Appl. , 2005, WO2005051387A1.
-
(a) S. Huang, R. Lin, Y. Yu, Y. Lu, P. J. Connolly, G. Chiu, S. Li, S. L. Emanuel and S. A. Middleton, Bioorg. Med. Chem. Lett., 2007, 17, 1243 CrossRef CAS PubMed;
(b) G. Chiu, S. Li, P. J. Connolly, S. A. Middleton, S. L. Emanuel, S. Huang, R. Lin, Y. Lu, PCT Int. Appl. , 2006, WO2006130673A1.
- R. Lin, G. Chiu, Y. Yu, P. J. Connolly, S. Li, Y. Lu, M. Adams, A. R. Fuentes-Pesquera, S. T. Emanuel and G. L. Greenberger, Bioorg. Med. Chem. Lett., 2007, 17, 4557 CrossRef CAS PubMed.
-
(a) M. F. Favata, K. Y. Horiuchi, E. J. Manos, A. J. Daulerio, D. A. Stradley, W. S. Feeser, D. E. Van Dyk, W. J. Pitts, R. A. Earl, F. Hobbs, R. A. Copeland, R. L. Magolda, P. A. Scherle and J. M. Trzaskos, J. Biol. Chem., 1998, 273, 18623 CrossRef CAS PubMed;
(b) M. A. Bogoyevitch and D. P. Fairlie, Drug Discovery Today, 2007, 12, 622 CrossRef CAS PubMed.
-
(a) C. Sticht, K. Freier, C. Flechtenmacher, S. Pungs, C. Hofele, M. Hahn, S. Joos and P. Lichter, Neoplasia, 2008, 10, 462 CAS;
(b) T. J. Dudderidge, S. R. McCracken, M. Loddo, T. R. Fanshawe, J. D. Kelly, D. E. Neal, H. Y. Leung, G. H. Williams and K. Stoeber, Br. J. Cancer, 2007, 96, 1384 CAS;
(c) J. C. Montero, A. Ocaña, M. Abad, M. J. Ortiz-Ruiz, A. Pandiella and A. Esparís-Ogando, PLoS One, 2009, 4, 5565 Search PubMed.
- P. T. Flaherty, I. Chopra, P. Jain, P. Monlish and P. Cavanaugh, Bioorg. Med. Chem., 2010, 18, 8054 CrossRef CAS PubMed.
-
(a) I. Kufareva and R. Abagyan, J. Med. Chem., 2008, 51, 7921 CrossRef CAS PubMed;
(b) P. T. Flaherty, I. Chopra, P. Jain, P. Monlish and P. Cavanaugh, Bioorg. Med. Chem. Lett., 2010, 20, 2892 CrossRef CAS PubMed.
- M. W. Karaman, S. Herrgard, D. K. Treiber, P. Gallant, C. E. Atteridge, B. T. Campbell, K. W. Chan, P. Ciceri, M. I. Davis, P. T. Edeen, R. Faraoni, M. Floyd, J. P. Hunt, D. J. Lockhart, Z. V. Milanov, M. J. Morrison, G. Pallares, H. K. Patel, S. Pritchard, L. M. Wodicka and P. P. Zarrinkar, Nat. Biotechnol., 2008, 26, 127 CrossRef CAS PubMed.
- M. J. Bamford, M. J. Alberti, N. Bailey, S. Davies, D. K. Dean, A. Gaiba, S. Garland, J. D. Harling, D. K. Jung, T. A. Panchal, C. A. Patt, J. G. Steadman, A. K. Takle, J. T. Townsend, D. M. Wilson and J. Witherington, Bioorg. Med. Chem. Lett., 2005, 15, 3402 CrossRef CAS PubMed.
- A. de Dios, C. Shih, B. Lopez de Uralde, C. Sanchez, M. del
Prado, L. M. M. Cabrejas, S. Pleite, J. Blanco-Urgoiti, M. J. Lorite, C. R. Nevill Jr, R. Bonjouklian, J. York, M. Vieth, Y. Wang, N. Magnus, R. M. Campbell, B. D. Anderson, D. J. McCann, D. D. Giera, P. A. Lee, R. M. Schultz, L. C. Li, L. M. Johnson and J. A. Wolos, J. Med. Chem., 2005, 48, 2270 CrossRef CAS PubMed.
- M. J. Bamford, N. Bailey, S. Davies, D. K. Dean, L. Francis, T. A. Panchal, C. A. Parr, S. Sehmi, J. G. Steadman, A. K. Takle, J. K. Townsend and D. M. Wilson, Bioorg. Med. Chem. Lett., 2005, 15, 3407 CrossRef CAS PubMed.
- M. Mader, A. de Dios, C. Shih, R. Bonjouklian, T. Li, W. White, B. L. de Uralde, C. Sánchez-Martinez, M. D. Prado, C. Jaramillo, E. de Diego, L. M. Martín Cabrejas, C. Dominguez, C. Montero, T. Shepherd, R. Dally, J. E. Toth, A. Chatterjee, S. Pleite, J. Blanco-Urgoiti, L. Perez, M. Barberis, M. Lorite, E. Jambrina, R. Nevill Jr, A. L. Paul, R. C. Schultz, J. A. Wolos, L. C. Li, R. M. Campbellb and B. D. Anderson, Bioorg. Med. Chem. Lett., 2008, 18, 179 CrossRef CAS PubMed.
-
(a) S. L. Warner, B. J. Stephens and D. D. Von Hoff, Curr. Oncol. Rep., 2008, 10, 122 CrossRef CAS PubMed;
(b) C. McInnes, M. Mezna and P. M. Fischer, Curr. Top. Med. Chem., 2005, 5, 181 CrossRef CAS.
- K. A. Emmitte, G. M. Adjebang, C. W. Andrews, G. A. Badiang Alberti, R. Bambal, S. D. Chamberlain, R. G. Davis-Ward, H. D. Dickson, D. F. Hassler, K. R. Hornberger, J. R. Jackson, K. W. Kuntz, K. W. Lansing, R. A. Mook Jr, K. E. Nailor, M. A. Pobanz, S. C. Smith, C. M. Sung and M. Cheung, Bioorg. Med. Chem. Lett., 2009, 19, 1694 CrossRef CAS PubMed.
-
(a) T. A. Rheault, K. H. Donaldson, J. G. Badiang-Alberti, R. G. Davis-Ward, C. W. Andrews, R. Bambal, J. R. Jackson and M. Cheung, Bioorg. Med. Chem. Lett., 2010, 20, 4587 CrossRef CAS PubMed;
(b) K. Emmitte, A. C. W. Andrews, J. G. Badiang, R. G. Davis-Ward, H. D. Dickson, D. H. Drewry, H. K. Emerson, D. F. Hassler, V. B. Knick, K. W. Kuntz, T. J. Lansing, J. A. Linn, R. A. Mook, K. E. Nailor, J. M. Salovich, G. M. Spehar and M. Cheung, Bioorg. Med. Chem. Lett., 2009, 19, 1018 CrossRef CAS PubMed.
-
(a) N. Ferrara, K. Carver-Moore, H. Chen, M. Dowd, L. Lu, K. S. O'Shea, L. Powell-Braxton, K. J. Hillan and M. W. Moore, Nature, 1996, 380, 439 CrossRef CAS PubMed;
(b) P. Carmeliet, V. Ferreira, G. Breier, S. Pollefeyt, L. Kieckens, M. Gertsenstein, M. Fahrig, A. Vandenhoeck, K. Harpal, C. Eberhardt, C. Declercq, J. Pawling, L. Moons, D. Collen, W. Risau and A. Nagy, Nature, 1996, 380, 435 CrossRef CAS PubMed.
-
(a) K. Reiss, C. D'Ambrosio, X. Tu, C. Tu and R. Baserga, Clin. Cancer Res., 1998, 4, 2647 CAS;
(b) D. Sachdev, S. L. Li, J. S. Hartell, Y. Fujita-Yamaguchi, J. S. Miller and D. Yee, Cancer Res., 2003, 63, 627 CAS;
(c) R. Baserga, Expert Opin. Ther. Targets, 2005, 9, 753 CrossRef CAS PubMed.
-
(a) K. Zimmermann, M. D. Wittman, M. G. Saulnier, U. Velaparthi, D. R. Langley, X. Sang, D. B. Frennesson, J. Carboni, A. Li, A. Greer, M. Gottardis, R. Attar, Z. Yang, P. Balimane, L. N. Discenza and D. Vyas, Bioorg. Med. Chem. Lett., 2008, 18, 4075 CrossRef CAS PubMed;
(b) K. Zimmermann, M. D. Wittman, M. G. Saulnier, U. Velaparthi, D. R. Langley, X. Sang, D. B. Frennesson, J. Carboni, A. Li, A. Greer, M. Gottardis, R. Attar, Z. Yang, P. Balimane, L. N. Discenza and D. Vyas, Bioorg. Med. Chem. Lett., 2010, 20, 1744 CrossRef CAS PubMed.
- M. Wittman, J. Carboni, R. Attar, B. Balasubramanian, P. Balimane, P. Brassil, F. Beaulieu, C. Chang, W. Clarke, J. Dell, J. Eummer, D. Frennesson, M. Gottardis, A. Greer, S. Hansel, W. Hurlburt, B. Jacobson, S. Krishnananthan, F. Y. Lee, A. Li, T. A. Lin, P. Liu, C. Ouellet, X. Sang, M. Saulnier, K. Stoffan, Y. Sun, U. Velaparthi, H. Wong, Z. Yang, K. Zimmermann, M. Zoeckler and D. Vyas, J. Med. Chem., 2005, 48, 5639 CrossRef CAS PubMed.
-
(a) J. M. Carboni, A. V. Lee, D. L. Hadsell, B. R. Rowley, F. Y. Lee, D. K. Bol, A. E. Camuso, M. Gottardis, A. F. Greer, C. P. Ho, W. Hurlburt, A. Li, M. G. Saulnier, U. Velaparthi, C. Wang, M. L. Wen, R. A. Westhouse, M. Wittman, K. Zimmermann, B. A. Rupnow and T. W. Wong, Cancer Res., 2005, 65, 3781 CrossRef CAS PubMed;
(b) P. Haluska, J. M. Carboni, D. A. Loegering, F. Y. Lee, M. Wittman, M. G. Saulnier, D. B. Frennesson, K. R. Kalli, C. A. Conover, R. M. Attar, S. H. Kaufmann, M. Gottardis and C. Erlichman, Cancer Res., 2006, 66, 362 CrossRef CAS PubMed.
-
(a) U. Velaparthi, M. Wittman, P. Liu, K. Stoffan, K. Zimmermann, X. Sang, J. Carboni, A. Li, R. Attar, M. Gottardis, A. Greer, C. Chang, B. Jacobson, J. Sack, Y. Sun, D. R. Langley, B. Balasubramanian and D. Vyas, Bioorg. Med. Chem. Lett., 2007, 17, 2317 CrossRef CAS PubMed;
(b) U. Velaparthi, P. Liu, B. Balasubramanian, J. Carboni, R. Attar, M. Gottardis, A. Li, A. Greer, M. Zoeckler, M. Wittman and D. Vyas, Bioorg. Med. Chem. Lett., 2007, 17, 3072 CrossRef CAS PubMed;
(c) M. Wittman, B. Balasubramanian, K. Stoffan, U. Velaparthi, P. Liu, S. Krishnananthan, J. Carboni, A. Li, A. Greer, R. Attar, M. Gottardis, C. Chang, B. Jacobson, Y. Sun, S. Hansel, M. Zoeckler and D. Vyas, Bioorg. Med. Chem. Lett., 2007, 17, 974 CrossRef CAS PubMed;
(d) M. G. Saulnier, D. B. Frennessn, M. D. Wittman, K. Zimmermann, U. Velaparthi, D. R. Langley, C. Struzynski, X. Sang, J. Carboni, A. Li, A. Greer, Z. Yang, P. Balimane, M. Gottardis, M. Attar and D. Vyas, Bioorg. Med. Chem. Lett., 2008, 18, 1702 CrossRef CAS PubMed.
-
(a) M. Wittman, J. Carboni, R. Attar, B. Balasubramanian, P. Balimane, P. Brassil, F. Beaulieu, C. Chang, W. Clarke, J. Dell, J. Eummer, D. Frennesson, M. Gottardis, A. Greer, S. Hansel, W. Hurlburt, B. Jacobson, S. Krishnanathan, F. Y. Lee, A. Li, T. A. Lin, P. Liu, C. Ouellet, X. Sang, M. Saulnier, K. Stoffan, Y. Sun, U. Velaparthi, H. Wong, Z. Yang, K. Zimmermann, M. Zoeckler and D. Vyas, J. Med. Chem., 2005, 48, 5639 CrossRef CAS PubMed;
(b) P. Haluska, J. M. Carboni, D. A. Loegering, F. Y. Lee, M. Wittman, M. G. Saulnier, D. B. Frennesson, K. R. Kalli, C. A. Conover, R. M. Attar, S. H. Kaufmann, M. Gottardis and C. Erlichman, Cancer Res., 2006, 66, 362 CrossRef CAS PubMed.
-
(a) S. Gibson, B. Leung, J. A. Squire, M. Hill, N. Arima, P. Goss, D. Hogg and G. B. Mills, Blood, 1993, 82, 1561 CAS;
(b) D. J. Fowell, K. Shinkai, X. C. Liao, A. M. Beebe, R. L. Coffman, D. R. Littman and R. M. Locksley, Immunity, 1999, 11, 399 CrossRef CAS;
(c) N. Yamada, Y. Kawakami, H. Kimura, H. Fukamachi, G. Baier, A. Altman, T. Kato, Y. Inagaki and T. Kawakami, Biochem. Biophys. Res. Commun., 1993, 192, 231 CrossRef CAS PubMed;
(d) E. M. Schaeffer, J. Debnath, G. Yap, D. McVicar, X. C. Liao, D. R. Littman, A. Sher, H. E. Varmus, M. J. Lenardo and P. L. Schwartzberg, Science, 1999, 284, 638 CrossRef CAS.
- M. P. Winters, D. J. Robinson, H. H. Khine, S. S. Pullen, J. R. Woska, E. L. Raymond, R. Sellati, C. L. Cywin, R. J. Snow, M. A. Kashem, J. A. Wolak, J. King, P. V. Kaplita, L. H. Liu, T. M. Farrell, R. DesJarlais, G. P. Roth, H. Takahashi and K. J. Moriarty, Bioorg. Med. Chem. Lett., 2008, 18, 5541 CrossRef CAS PubMed.
- B. N. Cook, J. Bentzien, A. White, P. A. Nemoto, J. Wang, C. C. Man, F. Soleymanzadeh, H. H. Khine, M. A. Kashem, S. Z. Kugler, J. P. Wolak, G. P. Roth, S. De Lombaert, S. S. Pullen and H. Takahashi, Bioorg. Med. Chem. Lett., 2009, 19, 773 CrossRef CAS PubMed.
- K. J. Moriarty, H. Takahashi, S. S. Pullen, H. H. Khine, R. H. Sallati, E. L. Raymond, J. R. Woska, D. D. Jeanfavre, G. P. Roth, M. P. Winters, L. Qiao, D. Ryan, R. DesJarlais, D. Robinson, M. Wilson, M. Bobko, B. N. Cook, H. Y. Lo, P. A. Nemoto, M. A. Kashem, J. P. Wolak, A. White, R. L. Magolda and B. Tomczuk, Bioorg. Med. Chem. Lett., 2008, 18, 5545 CrossRef CAS PubMed.
- R. J. Snow, A. Abeywardane, S. Campbell, J. Lord, M. A. Kashem, H. H. Khine, J. King, J. A. Kowalski, S. S. Pullen, T. Roma, G. P. Roth, C. R. Sarko, N. S. Wilson, M. P. Winters, J. P. Wolak and C. L. Cywin, Bioorg. Med. Chem. Lett., 2007, 17, 3660 CrossRef CAS PubMed.
- D. Riether, R. Zindell, J. A. Kowalski, B. A. Cook, J. Bentzien, S. A. Lombaert, D. Thomson, S. J. Kugler Jr, D. Skow, L. S. Martin, E. L. Raymond, E. E. Khine, K. O'Shea, J. R. Woska Jr, D. Jeanfavre, R. Sellati, K. L. M. Ralph, J. Ahlberg, G. Labissiere, M. A. Kashem, S. A. Pullen and H. Takahashi, Bioorg. Med. Chem. Lett., 2009, 19, 1588 CrossRef CAS PubMed.
- K. Moriarty, M. Winters, L. Qiao, D. Ryan, R. DesJarlais, D. Robinson, M. Wilson, M. Bobko, B. Cook, M. Kashem, P. Kaplita, L. Liu, T. Farrell, H. Khine, J. King, S. Pullen, G. Roth, R. Magolda and H. Takahashi, Bioorg. Med. Chem. Lett., 2008, 18, 5537 CrossRef CAS PubMed.
-
(a) J. Folkman, Nat. Rev. Drug Discovery, 2007, 6, 273 CrossRef CAS PubMed;
(b) L. K. Shawver, K. E. Lipson, T. A. T. Fong, G. McMahon, G. D. Plowman and L. M. Strawn, Drug Discovery Today, 1997, 2, 50 CrossRef CAS;
(c) J. Folkman, Nat. Med., 1995, 1, 27 CrossRef CAS PubMed.
- T. M. Hansen, H. Singh, T. A. Tahir and N. P. Brindle, Cell. Signalling, 2010, 22, 527 CrossRef CAS PubMed.
-
(a) N. W. Johnson, M. Semones, J. L. Adams, M. Hansbury and J. Winkler, Bioorg. Med. Chem. Lett., 2007, 17, 5514 CrossRef CAS PubMed;
(b) N. Jones, K. Iljin, D. J. Dumont and K. Alitalo, Nat. Rev. Mol. Cell Biol., 2001, 2, 257 CrossRef CAS PubMed;
(c) B. L. Hodous, S. D. Geuns-Meyer, P. E. Hughes, B. K. Albrecht, S. Bellon, J. Bready, S. Caenepeel, V. J. Cee, S. C. Chaffee, A. Coxon, M. Emery, J. Fretland, P. Gallant, Y. Gu, D. Hoffman, R. E. Johnson, R. Kendall, J. L. Kim, A. M. Long, M. Morrison, P. R. Olivieri, V. F. Patel, A. Polverino, P. Rose, P. Tempest, L. Wang, D. Whittington and H. Zhao, J. Med. Chem., 2007, 50, 611 CrossRef CAS PubMed;
(d) V. J. Cee, B. K. Albrecht, S. D. Geuns-Meyer, P. E. Hughes, S. Bellon, J. Bready, S. Caenepeel, S. C. Chaffee, A. Coxon, M. Emery, J. Fretland, P. Gallant, Y. Gu, B. L. Hodous, D. Hoffman, R. E. Johnson, R. Kendall, J. L. Kim, A. M. Long, D. McGowan, M. Morrison, P. R. Olivieri, V. F. Patel, A. Polverino, D. Powers, P. Rose, L. Wang and H. Zhao, J. Med. Chem., 2007, 50, 627 CrossRef CAS PubMed.
- V. J. Cee, A. C. Cheng, K. Romero, S. Bellon, C. Mohr, D. A. Whittington, A. Bak, J. Bready, S. Caenepeel, A. Coxon, H. L. Deak, J. Fretland, Y. Gu, B. L. Hodous, X. Huang, J. L. Kim, L. Lin, A. M. Long, H. Nguyen, H. R. Olivieri, V. F. Patel, L. Wang, Y. Zhou, P. Hughes and S. Geuns-Meyer, Bioorg. Med. Chem. Lett., 2009, 19, 424 CrossRef CAS PubMed.
- H. Yang, T. Burke, J. Dempsey, B. Diaz, E. Collins, J. Toth, R. Beckmann and X. Ye, FEBS Lett., 2005, 579, 3385 CrossRef CAS PubMed.
-
(a) E. S. Emamian, D. Hall, M. J. Birnbaum, M. Karayiorgou and J. A. Gogos, Nat. Genet., 2004, 36, 131 CrossRef CAS PubMed;
(b) Z. Wang, K. S. Smith, M. Murphy, O. Piloto, T. C. P. Somervaille and M. L. Cleary, Nature, 2008, 455, 1205 CrossRef CAS PubMed;
(c) A. Martinez, A. Castro, I. Dorronsoro and M. Alonso, Med. Res. Rev., 2002, 22, 373 CrossRef CAS PubMed.
-
(a) I. Dorronsoro, A. Castro and A. Martinez, Expert Opin. Ther. Pat., 2002, 12, 1527 CrossRef CAS;
(b) D. B. Ring, K. W. Johnson, E. J. Henriksen, J. M. Nuss and D. Goff, Diabetes, 2003, 52, 588 CrossRef CAS;
(c) J. V. Wauwe and B. Haefner, Drug News Perspect., 2003, 16, 557 CrossRef.
- S. Lee, D. Shin, J. M. Cho, S. Ro and Y. Suh, Bioorg. Med. Chem. Lett., 2012, 22, 1891 CrossRef CAS PubMed.
- N. Dessalew and P. V. Bharatam, Eur. J. Med. Chem., 2007, 42, 1014 CrossRef CAS PubMed.
- J. M. Cho, S. Ro, T. G. Lee, K. J. Lee, D. Shin, Y. L. Hyun, S. C. Lee, J. H. Kim and Y. H. Jeon, PCT Int. App. , 2004, WO2004065370.
- D. Shin, S. Lee, Y. Heo, W. Lee, Y. Cho, Y. E. Kim, Y. Hyun, J. M. Cho, Y. S. Leeb and S. Roa, Bioorg. Med. Chem. Lett., 2007, 17, 5686 CrossRef CAS PubMed.
- J. B. Bolen and J. S. Brugge, Annu. Rev. Immunol., 1997, 15, 371 CrossRef CAS PubMed.
- M. Sabat, J. C. VanRens, M. L. Laufersweiler, T. A. Brugel, J. Maier, A. Golebiowski, B. De, V. Easwaran, L. C. Hsieh, R. L. Walter, M. J. Mekel, A. Evdokimovb and M. J. Janusza, Bioorg. Med. Chem. Lett., 2006, 16, 5973 CrossRef CAS PubMed.
-
(a) W. Harris, C. H. Hill and I. E. D. Smith, PCT Int. Appl. , 2000, WO2000024744;
(b) T. Sim, H. S. Lee, P. Ren, Q. Ding, X. Wang, T. Uno, G. Zhang, Y. Liu, B. Li, L. Li, N. S. Gray and S. You, PCT Int. Appl. , 2005, WO2005011597;
(c) G. Zhang, P. Ren, N. S. Gray, T. Sim, T. Liu, X. Wang, J. Che, S. Tian, M. L. Sandberg, T. S. Spalding, R. Romeo, M. Iskandar, D. Chow, H. M. Seidel, D. S. Karanewsky and Y. He, Bioorg. Med. Chem. Lett., 2008, 18, 5618 CrossRef CAS PubMed.
-
(a) A. Jansma, Q. Zhang, B. Li, Q. Ding, T. Uno, B. Bursulaya, Y. Liu, P. Furet, N. S. Gray and B. H. Geierstanger, J. Med. Chem., 2007, 50, 5875 CrossRef CAS PubMed;
(b) P. Furet, G. Caravatti, V. Guagnano, M. Lang, T. Meyer and J. Schoepfer, Bioorg. Med. Chem. Lett., 2008, 18, 897 CrossRef CAS PubMed.
- P. Traxler and P. Furet, Pharmacol. Ther., 1999, 82, 195 CrossRef CAS.
- P. Bamborough, R. M. Angell, I. Bhamra, D. Brown, J. Bull, J. A. Christopher, A. W. J. Cooper, L. H. J. Fazal, I. Giordano, L. Hind, V. K. Patel, L. K. Ranshaw, M. J. Sims, P. A. Skone, K. J. Smith, E. Vickerstaff and M. Washington, Bioorg. Med. Chem. Lett., 2007, 17, 4363 CrossRef CAS PubMed.
- J. A. Hunt, R. T. Beresis, J. L. Goulet, M. A. Holmes, X. J. Hong, E. Kovacs, S. G. Mills, R. D. Ruzek, F. Wong, J. D. Hermes, Y. Park, S. P. Salowe, L. M. Sonatore, L. Wu, A. Woods, D. M. Zaller and P. J. Sinclair, Bioorg. Med. Chem. Lett., 2009, 19, 5440 CrossRef CAS PubMed.
-
(a) M. W. Martin, J. Newcomb, J. J. Nunes, C. Boucher, L. Chai, L. F. Epstein, T. Faust, S. Flores, P. Gallant, A. Gore, Y. Gu, F. Hsieh, X. Huang, J. L. Kim, S. Middleton, K. Morgenstern, A. Oliveira-dos-Santos, V. F. Patel, D. Poers, P. Rose, Y. Tudor, S. M. Turci, A. A. Welcher, D. Zack, H. Zhao, L. Zhu, X. Zhu, C. Ghiron, M. Ermann, D. Johnston and C. G. P. Saluste, J. Med. Chem., 2008, 51, 1637 CrossRef CAS PubMed;
(b) A. Burchat, Q. W. Borhani, D. J. Caldewood, G. C. Hirst, B. Li and R. F. Stachlewitz, Bioorg. Med. Chem. Lett., 2006, 16, 118 CrossRef CAS PubMed;
(c) J. A. Maier, T. A. Brugel, M. Sabat, A. Golebiowski, M. Laufersweiler, J. J. C. VanRens, C. R. Hopkins, B. De, L. C. Hsieh, K. K. Brown, V. Easwaran and M. J. Janusz, Bioorg. Med. Chem. Lett., 2006, 16, 3646 CrossRef CAS PubMed;
(d) P. Chen, A. M. Doweyko, D. Norris, H. H. Gu, S. H. Spergel, J. Das, R. V. Moquin, J. Lin, J. Wityak, E. J. Iwanowicz, K. W. McIntyre, D. J. Shuster, K. Behnia, S. Ching, H. F. de Fex, S. Pang, S. Pitt, D. R. Shen, S. Thrall, P. Stanley, O. R. Kocy, M. R. Witmer, S. B. Kanner, G. L. Schieven and J. C. Barrish, J. Med. Chem., 2004, 47, 4517 CrossRef CAS PubMed.
- D. J. Cole, D. P. Weil, J. Shilyansky, M. Custer, Y. Kawakami, S. A. Rosenberg and M. I. Nishimura, Cancer Res., 1995, 55, 748 CAS.
-
(a) L. Savelyeva and M. Schwab, Cancer Lett., 2001, 167, 115 CrossRef CAS;
(b) D. H. Kim and T. Sim, Arch. Pharmacal Res., 2012, 35, 605 CrossRef CAS PubMed;
(c) S. T. Yuen, H. Davies, T. L. Chan, J. W. Ho, G. R. Bignell, C. Cox, P. Stephens, S. Edkins, W. W. Tsui, A. S. Chan, P. A. Futreal, M. R. Stratton, R. Wooster and S. Y. Leung, Cancer Res., 2002, 62, 6451 CAS.
-
(a) H. Buchstaller, L. Burgdorf, D. Finsinger, F. Stieber, C. Sirrenberg, C. Amendt, M. Grell, F. Zenke and M. Krier, Bioorg. Med. Chem. Lett., 2011, 21, 2264 CrossRef CAS PubMed;
(b) M. Hasegawa, N. Nishigaki, Y. Washio, K. Kano, P. A. Harris, H. Sato, I. Mori, R. I. West, M. Shibahara, H. Toyoda, L. Wang, R. T. Nolte, J. M. Veal and M. Cheung, J. Med. Chem., 2007, 50, 4453 CrossRef CAS PubMed.
-
(a) S. Ramurthy, M. Aikawa, P. Amiri, A. Costales, A. Hashash, J. M. Jansen, S. Lin, S. Mab, P. A. Renhowe, C. M. Shafer, S. Subramanian, L. Sung and L. Verhagen, Bioorg. Med. Chem. Lett., 2011, 21, 3286 CrossRef CAS PubMed;
(b) S. Ramurthy, A. Costales, M. Johanna, B. Levine, A. R. Paul, M. S. Cynthia and S. Subramanian, Bioorg. Med. Chem. Lett., 2012, 22, 1678 CrossRef CAS PubMed;
(c) S. Ramurthy, S. Subramanian, M. Aikawa, A. Payman, A. Costales, J. Dove, S. Fong, J. M. Jansen, B. Levine, S. Ma, C. M. McBride, J. Michaelian, T. Pick, D. J. Poon, S. Girish, C. M. Shafer, D. Stuart, L. Sung and P. A. Renhowe, J. Med. Chem., 2008, 51, 7049 CrossRef CAS PubMed.
- J. R. Burke, Curr. Opin. Drug Discovery Dev., 2003, 6, 720 CAS.
- J. A. Christopher, B. G. Avitabile, P. Bamborough, A. C. Champigny, G. J. Cutler, S. L. Dyos, K. G. Grace, J. K. Kerns, J. D. Kitson, G. W. Mellor, J. W. Morey, M. A. Morse, C. F. O'Malley, C. B. Patel, N. Probst, W. Rumsey, C. A. Smithb and M. J. Wilsona, Bioorg. Med. Chem. Lett., 2007, 17, 3972 CrossRef CAS PubMed.
- Y. Chen, T. Bannister, A. Weiser, E. Griffin, L. Lin, C. Ruiz, M. Cameron, S. Schürer, D. Duckett, T. Schröter, P. LoGrasso and Y. Feng, Bioorg. Med. Chem. Lett., 2008, 18, 6406 CrossRef CAS PubMed.
-
(a) Y. Yin, M. D. Cameron, L. Lin, S. Khan, T. Schröter, W. Grant, J. Pocas, Y. T. Chen, S. Schürer, A. Pachori, P. LoGrasso and Y. Feng, ACS Med. Chem. Lett., 2010, 1, 175 CrossRef CAS;
(b) Y. Yin, L. Lin, C. Ruiz, M. Cameron, J. Pocas, W. Grant, T. Schröter, W. Chen, D. Duckett, S. Schürer, P. LoGrasso and Y. Feng, Bioorg. Med. Chem. Lett., 2009, 19, 6686 CrossRef CAS PubMed;
(c) X. Fang, Y. Yin, Y. T. Chen, L. Yao, B. Wang, M. D. Cameron, L. Lin, S. Khan, C. Ruiz, T. Schröter, W. Grant, A. Weiser, J. Pocas, A. Pachori, S. Schürer, P. Lo Grasso and Y. Feng, J. Med. Chem., 2010, 53, 5727 CrossRef CAS PubMed.
- E. H. Sessions, M. Smolinksi, B. Wang, B. Frackowiak, S. Chowdhury, Y. Yin, Y. T. Chen, C. Ruiz, L. Lin, J. Pocas, T. Schröter, M. Cameron, P. LoGrasso, Y. Feng and T. Bannister, Bioorg. Med. Chem. Lett., 2010, 20, 1939 CrossRef CAS PubMed.
- E. Sessions, Y. Yin, T. Bannister, A. Weiser, E. Griffin, J. Pocas, M. Cameron, C. Ruiz, L. Lin, S. Schürer, T. Schröter, P. LoGrasso and Y. Feng, Bioorg. Med. Chem. Lett., 2008, 18, 6390 CrossRef CAS PubMed.
- X. Fang, Y. T. Chen, E. H. Sessions, S. Chowdhury, T. Vojkovsky, Y. Yin, J. R. Pocas, W. Grant, T. Schröter, L. Lin, C. Ruiz, M. D. Cameron, P. LoGrasso, T. D. Bannister and Y. Feng, Bioorg. Med. Chem. Lett., 2011, 21, 1844 CrossRef CAS PubMed.
-
(a) P. Blume-Jensen and T. Hunter, Nature, 2001, 411, 355 CrossRef CAS PubMed;
(b) L. D. Klaman, O. Boss, O. D. Peroni, J. K. Kim, J. L. Martino, J. M. Zabolotny, N. Moghal, M. Lubkin, Y. B. Kim, A. H. Sharpe, A. Stricker-Krongrad, G. I. Shulman, B. G. Neel and B. B. Kahn, Mol. Cell. Biol., 2000, 20, 5479 CrossRef CAS.
-
(a) P. Carmeliet and R. K. Jain, Nature, 2000, 407, 249 CrossRef CAS PubMed;
(b) A. Giatromanolaki, E. Sivridis, N. Athanassou, E. Zois, P. E. Thorpe, R. A. Brekken, K. C. Gatter, A. L. Harris, I. M. Koukourakis and M. I. Koukourakis, J. Pathol., 2001, 194, 101 CrossRef CAS PubMed;
(c) M. Detmar, J. Dermatol. Sci., 2000, 24, 78 CrossRef.
- A. G. Cole, A. C. Bohnstedt, V. Paradkar, C. Kingsbury, J. G. Quintero, H. Park, Y. Lu, M. You, I. Neagu, D. J. Diller, J. J. Letourneau, Y. Shao, R. A. James, C. M. Riviello, K. Ho, T. H. Lin, B. Wang, K. C. Appell, M. Sills, E. Quadros, E. F. Kimble, M. H. J. Ohlmeyer and M. L. Webb, Bioorg. Med. Chem. Lett., 2009, 19, 6788 CrossRef CAS PubMed.
-
(a) T. Hamaguchi, A. Takahashi, T. Kagamizono, A. Manaka, M. Satoa and M. Osadab, Bioorg. Med. Chem. Lett., 2000, 10, 2657 CrossRef CAS;
(b) M. H. Potashman, J. Bready, A. Coxon, T. M. DeMelfi, L. DiPietro, N. Doerr, D. Elbaum, J. Estrada, P. Gallant, J. Germain, Y. Gu, J. C. Harmange, S. A. Kaufman, R. Kendall, J. L. Kim, G. N. Kumar, A. M. Long, S. Neervannan, V. M. Patel, A. Polverino, P. Rose, S. van der Plas, D. Whittington, R. Zanon and H. Zhao, J. Med. Chem., 2007, 50, 4351 CrossRef CAS PubMed.
-
(a) M. Ruzzene, D. Penzo and L. A. Pinna, Biochem. J., 2002, 364, 41 CAS;
(b) R. Ravi and A. Bedi, Cancer Res., 2002, 62, 4180 CAS.
-
(a) M. Daya-Makin, J. S. Sanghera, T. L. Mogentale, M. Lipp, J. Parchomchuk, J. C. Hogg and S. L. Plech, Cancer Res., 1994, 54, 2262 CAS;
(b) K. Ahmed, Cell. Mol. Biol. Res., 1994, 40, 1 CAS.
- S. Sarno, H. Reddy, F. Meggio, M. Ruzzene, S. P. Davies, A. Donella-Deana, D. Shugar and L. A. Pinna, FEBS Lett., 2001, 496, 44 CrossRef CAS.
- R. Battistutta, M. Mazzorana, L. Cendron, A. Bortolato, S. Sarno, Z. Kazimierczuk, G. Zanotti, S. Moro and L. A. Pinna, ChemBioChem, 2007, 8, 1804 CrossRef CAS PubMed.
-
(a) F. Meggio, D. Shugar and L. A. Pinna, Eur. J. Biochem., 1990, 187, 89 CrossRef CAS;
(b) J. Raaf, E. Brunstein, O. G. Issinger and K. Niefind, Chem. Biol., 2008, 15, 111 CrossRef CAS PubMed.
- M. Andrzejewska, A. Pagano, F. Meggio, A. F. Brunatib and Z. Kazimierczuka, Bioorg. Med. Chem., 2003, 11, 3997 CrossRef CAS.
- M. Janeczko, A. Orzeszko, Z. Kazimierczuk, R. Szyszka and A. Baier, Eur. J. Med. Chem., 2012, 47, 345 CrossRef CAS PubMed.
- Z. Hua, X. Huang, H. Bregman, N. Chakka, E. F. DiMauro, E. M. Doherty, J. Goldstein, H. Gunaydin, H. Huang, S. Mercede, J. Newcomb, V. F. Patel, S. M. Turci, J. Yan, C. Wilson and M. W. Martin, Bioorg. Med. Chem. Lett., 2012, 22, 5392 CrossRef CAS PubMed.
- J. Trujillo, J. R. Kiefer, W. Huang, A. Thorarensen, A. Xing, N. L. Caspers, N. L. Day, K. L. Mathis, K. K. Kretzmer, B. A. Reitz, R. A. Weinberg, R. A. Stegeman, R. Wrightstone, L. Christine, L. Compton and X. Li, Bioorg. Med. Chem. Lett., 2009, 19, 908 CrossRef CAS PubMed.
|
This journal is © The Royal Society of Chemistry 2014 |
Click here to see how this site uses Cookies. View our privacy policy here.