DOI:
10.1039/C3RA47644H
(Paper)
RSC Adv., 2014,
4, 19396-19402
Ionic liquids from amino acids: fully green fluid lubricants for various surface contacts†
Received
15th December 2013
, Accepted 28th February 2014
First published on 4th March 2014
Abstract
Five tetrabutylammonium-based amino acid ionic liquids (AAILs), tetrabutylammonium serine ([N4444][Ser]), tetrabutylammonium threonine ([N4444][Thr]), tetrabutylammonium valine ([N4444][Val]), tetrabutylammonium leucine ([N4444][Leu]) and tetrabutylammonium lysine ([N4444][Lys]) have been easily prepared by the neutralization of tetrabutylammonium hydroxide ([N4444][OH]) with the five corresponding amino acids. These AAILs only contain the elements C, N, O and H in their structures, so they are more environmentally friendly than conventional phosphorus-, halogen- or sulfur-containing lubricants. The preparation process of these AAILs is simple and also fully green, because no other by-products except water were produced during the whole process. The AAILs were evaluated as green lubricants for various surface contacts, such as steel/steel, steel/copper and steel/aluminum, using an Optimol SRV-IV oscillating reciprocating friction and wear tester at room temperature (RT). Due to their halogen-free character, these AAILs have a higher hydrolysis stability and a far lower corrosion level than the halogen-containing lubricants imidazolium ILs, 1-butyl-3-methylimidazolium tetrafluoroborate ([C4mim][BF4]) and 1-hexyl-3-methylimidazolium bis(trifluoromethylsulfonyl)imide ([C6mim][NTf2]), which were also tested. The tribotest results revealed that these AAILs could act as lubricants to effectively reduce friction and wear of all sliding pairs, and they even showed superior tribological performances compared to poly-alpha-olefin (PAO40) and [C6mim][NTf2] under the experimental conditions. The worn surfaces of the lower discs were analyzed by X-ray photoelectron spectrometry (XPS). Based on the XPS results, it can be concluded that the good lubricating properties of the AAILs are attributed to the formation of physically adsorbed layers on the metal surfaces during the rubbing process.
1 Introduction
Globally, there is a growing awareness of the environmental impact of man-made chemicals used in various aspects of our daily life. The replacement of environmentally polluting lubricants by environmentally friendly compounds is one of the most important efforts being undertaken in modern tribology and in green chemistry. Other than their primary purpose of reducing friction and wear, “green lubricants” are expected to be chemically and thermally stable, involatile, environmentally benign and even biodegradable, in order to meet environmental requirements. The potential abilities of ionic liquids (ILs) to fulfill the requirements of environmental sustainability are remarkable, and they have been highlighted as versatile green liquids in a variety of industrial applications. During the last decade, ILs have been investigated as promising advanced lubricants and lubricant additives due to their remarkable properties, including negligible volatility, non-flammability, low melting point, high thermal stability and a broad liquid range.1–6 Literature studies in the tribological field were mainly focused on halogen-containing ILs, based on anions including [BF4]−, [PF6]−, [SO3CF3]−, [NTf2]− and so on.7–10 However, there are some potential disadvantages in using this kind of IL as a lubricant. For example, [BF4]− and [PF6]− based IL lubricants are easily hydrolyzed by moisture to generate corrosive HF, which can cause corrosion to the substrates and pollution to the environment.1,11 Highly toxic compounds containing P, F or S may be generated when phosphonium, [BF4]−, [PF6]− or [SO3CF3]− are used, which may also pollute the environment.1 Additionally, there is a significant cost drawback associated with the preparation of conventional halogen-containing ILs.12,13 These disadvantages limit the large-scale applications of ILs in the lubricant industry. Therefore, the development of hydrolysis-stable, “fully green” and relatively cheap IL lubricant alternatives is still highly desirable.
Since amino acid ionic liquids (AAILs) were first synthesized and reported by Fukumoto and co-workers,14 they have received considerable attention from industry and the academic community.15–17 AAILs were highlighted as candidates to act as a platform for functional ILs due to their various residue groups and chiral carbon atom.18–20 Moreover, AAILs are easily biodegradable and biocompatible.14,21–23 Moreover, amino acids are easily obtained in large quantities at low cost, and the synthesis process of AAILs does not involve costly high pressure or high temperature (they are usually synthesized at ambient air pressure or temperature). Consequently, these AAILs are expected to find valuable applications in the lubricant industry as well as the biological, medical and pharmaceutical sciences. However, as far as we know, research on AAILs as neat lubricants is rarely found in the literature. Bearing this in mind, we decided to look for new AAILs to be used as promising green lubricants and lubricant additives for base oils or water. Our present work reports five tetrabutylammonium-based AAILs [tetrabutylammonium serine ([N4444][Ser]), tetrabutylammonium threonine ([N4444][Thr]), tetrabutylammonium valine ([N4444][Val]), tetrabutylammonium leucine ([N4444][Leu]) and tetrabutylammonium lysine ([N4444][Lys])], and their tribological properties as green lubricants for various surface contacts. It is clearly seen that these AAILs only contain the elements C, N, O and H in their structures, so they are more environmentally friendly than conventional phosphorus-, halogen- or sulfur-containing IL lubricants. The preparation process of these AAILs is simple, low-cost and also green, because no other by-products except water were produced during the whole process. It provides a practical way for expected bulk applications of fully green lubricants in industry in the future.
2 Experimental
2.1 Synthesis of AAILs
The chemical structures of all lubricants used are shown in Fig. 1 and Table 1. The following reagents were used as received: [N4444][OH] (40% in water) and five L-amino acids, including L-serine, L-threonine, L-valine, L-leucine and L-lysine, were all purchased from J&K Scientific Ltd. and used without further purification. The oil poly-alpha-olefin (PAO40) was purchased from the ExxonMobil Chemical Company. The halogen-containing imidazolium ILs, 1-butyl-3-methylimidazolium tetrafluoroborate ([C4mim][BF4]) and 1-hexyl-3-methylimidazolium bis(trifluoromethylsulfonyl)imide ([C6mim][NTf2]), were synthesized according to previously reported methods.24 The AAILs were synthesized according to a modified literature procedure, which was carried out by simply neutralizing the corresponding amino acids with [N4444][OH].14 An [N4444][OH] aqueous solution was added dropwise to an equimolar amino acid aqueous solution. The mixture was stirred at room temperature (RT) for 12 h. After evaporating the water, the neat product was further dried under vacuum at 80 °C for at least 2 days. It was clearly seen that no other by-products, except water, were produced in the preparation of these AAILs. This preparation process is simple, low-cost and fully green. The structures of the AAILs were characterized by nuclear magnetic resonance (NMR) spectroscopy (see ESI†).
 |
| Fig. 1 Molecular structures of all lubricants used: (a) AAILs, (b) PAO40, (c) [C4mim][BF4] and (d) [C6mim][NTf2]. | |
Table 1 Chemical structures of the anions of the AAILs
AAIL |
[N4444][Ser] |
[N4444][Thr] |
[N4444][Val] |
[N4444][Leu] |
[N4444][Lys] |
A− |
 |
 |
 |
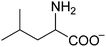 |
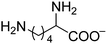 |
2.2 Characterization
The average moisture content (MC) of the AAILs was determined by a Karl Fischer titrator (TP653). An SYP1003-III kinematic viscosity tester of petroleum products was used to measure the kinematic viscosity (η) of all ILs at 40 °C and 100 °C. The viscosity index (VI) was calculated according to the ISO 2909-1981 procedure by software. The thermal behavior of all ILs was studied using an STA 449F3 simultaneous thermogravimetry instrument (TG, NETZSCH). The samples were heated from RT to 400 °C at a rate of 10 °C min−1 in air. The phase transition temperatures of the AAILs were measured on a DSC 200F3 differential scanning calorimeter (DSC, NETZSCH). The samples were first cooled to about −130 °C with liquid nitrogen and then heated to 30 °C at a rate of 10 °C min−1.
2.3 Hydrolysis stability analysis
The AAILs were dissolved in equal quantities of water and then the mixtures were heated to 80 °C. The pH-values of the solutions were checked at intervals of 30 min to determine the hydrolysis stability of the AAILs. This property was also checked for [C4mim][BF4] and [C6mim][NTf2] under the same conditions.
2.4 Copper strip corrosion test
The copper strip corrosion test was performed by imitating the GB-T5096-1985(91) procedure. The length, width and thickness of the copper strips used were 12.0 mm, 8.0 mm and 3.0 mm, respectively. The copper strips were ground, polished until bright and completely cleaned with acetone, before being immersed in the IL samples (AAILs, [C4mim][BF4] and [C6mim][NTf2]). Then the samples were heated to 100 °C for 72 h. At the end of the test, the copper strips were washed with acetone and compared with the corrosion standard tint board.
2.5 Friction and wear test
The tribological properties of all lubricants were assessed on an Optimol SRV-IV oscillating reciprocating friction and wear tester with a ball-on-disc configuration. All friction experiments were conducted at RT, under an amplitude of 1 mm, a frequency of 25 Hz, a test duration of 30 min and a relative humidity of 20–50%. Contact between the frictional pairs was obtained by pressing the upper running ball against the lower stationary disc. The upper running ball was driven to reciprocate at a given frequency and displacement. The balls for all contacts were made of AISI 52100 steel with a diameter of 10 mm and a hardness of 700–800 HV. The lower stationary discs (ø 24 mm × 7.9 mm) were made of AISI 52100 steel, Cu alloy and 2024 Al alloy with hardnesses of 700–800 HV, 140–160 HV and 150–180 HV, respectively. Prior to the friction and wear test, a moderate amount of lubricant was applied to the ball-disc contact area. The corresponding friction curves were automatically recorded by the SRV test rig. The wear volume losses of the lower discs were measured using a MicroXAM-3D non-contact surface mapping microscope profilometer. At least three replicates were carried out for each test condition. The average values are reported in this paper.
XPS measurement was carried out on a PHI-5702 multifunctional X-ray photoelectron spectrometer, with Al-Kα radiation as the excitation source. The binding energies of the target elements were determined at a pass energy of 29.35 eV and the resolution was ±0.3 eV, with the binding energy of contaminated carbon (C1s: 284.8 eV) as a reference.
3 Results and discussion
3.1 Physical properties
Table 2 summarizes the physical properties of all ILs used in this work. The data revealed that the moisture content of the AAILs is less than 0.2%. And it is clearly seen that all AAILs have high viscosities, at both 40 °C and 100 °C. [N4444][Thr] and [N4444][Val] have similar chemical structures except for a hydroxyl group in the anion structure of [N4444][Thr]. This hydroxyl group leads to a high viscosity due to the effects of hydrogen bonding, so [N4444][Thr] has a higher kinematic viscosity than [N4444][Val] at both 40 °C and 100 °C. The viscosities of the ILs generally increase as the molecular weight increases. However, [N4444][Leu] has a much lower kinematic viscosity than [N4444][Val] at both 40 °C and 100 °C even though [N4444][Leu] has a higher molecular weight. This may be attributed to the more flexible structure of [N4444][Leu] compared to that of [N4444][Val] (there is a methylene group in the structure of [N4444][Leu]). So in this case, the flexibility of the molecular structures of the AAILs becomes the main parameter which affects the kinematic viscosity. [N4444][Lys] has a moderate kinematic viscosity among the five AAILs. This may be attributed to the effects of its chemical structure and molecular weight, and the additional interaction forces among the ions derived from its second amino group.23 All of the AAILs except [N4444][Val] exhibit middle-of-the-range viscosity indexes. Their viscosity indexes are not good enough compared with [C4mim][BF4], [C6mim][NTf2] and other previously studied ILs,25,26 which have high viscosity indexes.
Table 2 Physical properties of the AAILs
AAIL |
MC/ppm |
η/mm2 s−1 |
VI |
Tonset/°C |
Tg/°C |
40 °C |
100 °C |
[N4444][Ser] |
1257.8 |
2991.50 |
61.04 |
31.69 |
167.8 |
−49.8 |
[N4444][Thr] |
1661.2 |
1906.87 |
48.18 |
33.95 |
172.6 |
−41.4 |
[N4444][Val] |
1465.3 |
1469.90 |
36.97 |
3.04 |
165.9 |
−50.4 |
[N4444][Leu] |
1308.6 |
1007.15 |
34.01 |
35.89 |
164.5 |
−46.1 |
[N4444][Lys] |
1552.8 |
2300.86 |
64.09 |
66.32 |
170.2 |
−43.9 |
[C4mim][BF4] |
— |
41.89 |
6.36 |
99.52 |
336.7 |
— |
[C6mim][NTf2] |
— |
27.20 |
5.39 |
136.90 |
354.5 |
— |
The thermogravimetric analysis (TGA) curves and typical differential scanning calorimetry (DSC) traces of the AAILs are shown in Fig. 2 and the corresponding data are listed in Table 2. Fig. 2a and the data in Table 2 reveal that the onset decomposition temperatures (Tonset) of [N4444][Ser], [N4444][Thr], [N4444][Val], [N4444][Leu] and [N4444][Lys] are in the range of 164.5 to 172.6 °C, which is lower than those of [C4mim][BF4], [C6mim][NTf2] and the ILs mentioned in the literature.25,27 However, this temperature is high enough for them to be used as neat lubricants at RT. DSC measurement results (in Fig. 2b and Table 2) show that these AAILs had no melting points in the measured temperature range, but had glass transition temperatures (Tg) ranging from −50.4 to −41.4 °C. The glass transition temperature depended on the chain structures of the anions. An increase in alkyl side-chain length coincided with a gradual increase in the glass transition temperature, probably due to the increasing van der Waals forces between alkyl side chains.14 So the glass transition temperature of [N4444][Thr] (−41.4 °C) is higher than that of [N4444][Ser] (−49.8 °C) (as there is a methyl group in the structure of [N4444][Thr] but not [N4444][Ser]). Besides, hydrogen bonding derived from the hydroxyl group in the anion structure should increase the glass transition temperature value.23 For example, [N4444][Thr] (−41.4 °C) has a higher glass transition temperature than [N4444][Val] (−50.4 °C).
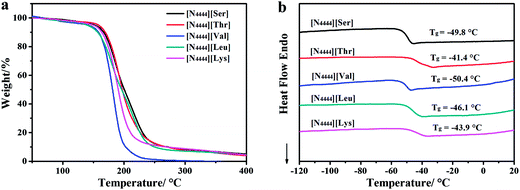 |
| Fig. 2 (a) TGA and (b) DSC curves of the AAILs. | |
3.2 Hydrolysis stability
To confirm the expected hydrolysis stabilities of the AAILs, we stirred each AAIL with the same quantity of water at 80 °C for several hours. The pH-value of the solutions was checked at regular intervals of 30 min.28 The halogen-containing ILs, [C4mim][BF4] and [C6mim][NTf2], were also used as a comparison, under the same conditions. The neutral aqueous solution of [C4mim][BF4] quickly changed to an acidic solution within the first 30 min of this experiment, which illustrates the hydrolysis instability of [C4mim][BF4] with the generation of HF under these conditions. No change of pH-value was determined for [C6mim][NTf2] or any of the AAILs during the 12 hour period. The above results indicate that [C6mim][NTf2] and the synthesized AAILs are hydrolysis stable in aqueous solution, even when the temperature rises to 80 °C. The good hydrolysis stabilities of the AAILs can be attributed to their halogen-free characters. It is reasonable to assume that these AAILs are suitable for use as lubricants, in terms of their hydrolysis stabilities.
3.3 Copper strip corrosion test
The copper strip corrosion test was used to confirm the anti-corrosion properties of the AAILs. It was performed by immersing a piece of bright copper sheet in a certain amount of IL (AAIL, [C4mim][BF4] and [C6mim][NTf2]), which was heated at 100 °C for 72 h. Fig. 3 shows the photographs of the copper sheets before (Fig. 3a) and after (Fig. 3b–h) the corrosion test. This reveals that the copper sheets soaked in the AAILs were hardly corroded, and the surfaces of the copper sheets showed no color change (Fig. 3b–f). However, the color of the copper sheets soaked in [C4mim][BF4] and [C6mim][NTf2] (Fig. 3g and h) changed significantly under the same experimental conditions, which indicates that severe corrosion occurred in these cases. The copper corrosion control standard shade guide was used to determine the corrosion levels. It was found that the corrosion grades of the AAILs were all 1a, whereas those of the [C4mim][BF4] and [C6mim][NTf2] were 2a and 1b, respectively. These results indicate that the AAILs do not corrode copper, and are qualified as lubricants.
 |
| Fig. 3 The photographs of copper sheets before (a) and after (b–h) the corrosion test: (b) [N4444][Ser], (c) [N4444][Thr], (d) [N4444][Val], (e) [N4444][Leu], (f) [N4444][Lys], (g) [C4mim][BF4] and (h) [C6mim][NTf2]. | |
3.4 Friction and wear test
3.4.1 As lubricants for steel/steel contact. The tribological properties of the AAILs were first tested on steel/steel contacts, as steel is the most widely used material in various machines in our daily lives. The commercial oil PAO40 and a conventional IL [C6mim][NTf2] were chosen as a comparison. Fig. 4 compares the friction coefficient traces and wear volume losses of the steel discs lubricated by all lubricants. Fig. 4a shows that the friction coefficient trends for all lubricants are quite similar. All of them have a short running-in time and then transition to a lower steady-state value. However, all AAILs produce a lower friction coefficient value than [C6mim][NTf2] and especially PAO40, which proves the better friction-reducing performances of the former. As shown in Fig. 4b, the wear volume losses of the steel discs lubricated by all AAILs are lower than that of the [C6mim][NTf2], but higher than that of the PAO40. This indicates that the anti-wear properties of the AAILs should be improved compared with PAO40. Among the five AAILs, [N4444][Ser] and [N4444][Thr] exhibit the best anti-wear properties. This may be attributed to their anionic moiety. That is to say, the hydroxyl groups in the anion structures of [Ser]− and [Thr]− lead to the formation of more effective protection films on the metal surfaces due to the effect of hydrogen bonding, and these provide effective separation of the steel surfaces, further reducing friction and wear. The above results confirm the excellent tribological properties of the AAILs under these experimental conditions. Besides, from an application point of view, these five AAILs are more qualified to be used as lubricants than [C6mim][NTf2], because of the lower cost associated with their preparation, and their intrinsic environmentally friendly characters.
 |
| Fig. 4 Evolution of the friction coefficients (a) and wear volume losses (b) of steel discs after lubrication at RT (load = 200 N, stroke = 1 mm, frequency = 25 Hz, duration = 30 min). | |
3.4.2 As lubricants for steel/copper contact. Cu alloys are widely used as bearing materials in the field of bearing manufacture, but they are usually difficult to lubricate due to their low Young's modulus.29 The AAILs were selected to lubricate Cu alloys in our study. PAO40 and [C6mim][NTf2] were also chosen as a comparison. The changes in friction coefficients and the wear volume losses of the copper discs under lubrication by all of the oils are displayed in Fig. 5. The evolution of friction coefficients in Fig. 5a shows that [C6mim][NTf2] and [N4444][Leu] start at a moderately high value and then tend to become lower and more stable. During the next rubbing process, the five AAILs exhibit similar friction coefficient values to that of [C6mim][NTf2], and all the friction coefficients are low and stable. However, PAO40 produces the largest friction coefficient value, and an increasing friction trace, as time increases. As shown in Fig. 5b, the wear volume losses of all the AAILs are still larger than that of the PAO40 but smaller than that of the [C6mim][NTf2]. The above results reveal that all of the AAILs have good lubricating effects for steel/copper contact. So in this case, the AAILs can substitute PAO40 and especially halogen-containing ILs for use as neat lubricants for steel/copper contact. Additionally, the environmentally friendly and outstanding anti-corrosion properties of the AAILs also confirm that they are suitable for the lubrication of steel/copper contact.
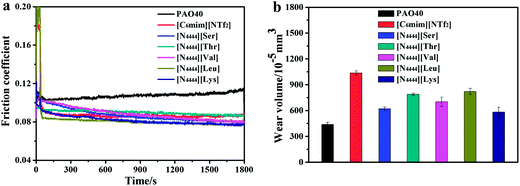 |
| Fig. 5 Evolution of the friction coefficients (a) and wear volume losses (b) of copper discs after lubrication at RT (load = 100 N, stroke = 1 mm, frequency = 25 Hz, duration = 30 min). | |
3.4.3 As Lubricants for steel/aluminum contact. The unique characteristics of aluminium alloys, such as a high strength to weight ratio, good corrosion resistance in an ambient environment and high thermal conductivity, have promoted their use in bearing components, particularly in the automotive industry.7,30 Among the lubrication of light alloys, the most studied are aluminium alloys, but their relatively poor wear-resistance makes them especially difficult to be lubricated at a modest load. Effective lubrication is particularly required for their smooth and reliable operation. We found that the five AAILs studied are effective lubricants for steel/aluminum contact. The friction coefficients, and a photograph of the wear scars for all lubricants are shown in Fig. 6. The wear volume losses of the lower discs lubricated by [C6mim][NTf2] are too large to be measured by a MicroXAM 3D noncontact surface mapping microscope profilometer, so the photograph of the worn surface is given in Fig. 6b. As can be observed from Fig. 6a, all AAILs show a short running-in time and a very smooth and constant low friction value (about 0.10). PAO40 shows a longer running-in time and a slightly larger friction coefficient value. In the case of [C6mim][NTf2], the friction coefficient starts with a longer running-in time than those of the AAILs, and then stays at a high value (about 0.19). In Fig. 6b, PAO40 shows a slightly larger wear scar (numbered 1) than the AAILs (numbered 3–7), and the largest wear scar (numbered 2) is observed after lubrication by [C6mim][NTf2]. In contrast, the AAILs exhibit excellent anti-wear properties with slight wear (numbered 3–7). The results indicate that the halogen-containing IL [C6mim][NTf2] is not an efficient lubricant for aluminum, and that severe wear may be caused by its tribo-corrosion during the sliding process.30 On the contrary, the AAILs are effective lubricants for this kind of aluminum alloy, and their tribological properties can be compared with those of PAO40.
 |
| Fig. 6 Evolution of the friction coefficients (a) and a photograph of the wear surface (b) for aluminum discs after lubrication at RT (load = 100 N, stroke = 1 mm, frequency = 25 Hz, duration = 30 min). | |
XPS analysis of the wear surfaces was carried out to explore the friction-reducing and anti-wear mechanism of the AAILs. However, no characteristic peaks of N1s, which has the most important role in proving the occurrence of a tribochemical reaction on a metal surface, were detected. Besides, the binding energies of C1s, O1s, Fe2p, Cu2p and Al2p on the worn surfaces lubricated by the AAILs are similar to those on a blank (non-lubricated) surface. It is well known that carboxylic acid can be easily adsorbed onto rubbed metallic surfaces to reduce friction and wear.31 Therefore, in this case, we speculate that the outstanding tribological properties of the AAILs are mostly attributed to the formation of physical adsorption films on the metal-worn surfaces. These physical adsorption films may have been removed by ultrasonic rinsing before the XPS test, meaning that no characteristic peaks of N1s were detected. As shown in Fig. 7, during the rubbing process, low-energy electrons are emitted from contact convex points on the rubbed metal surfaces, so carboxyl groups were firstly adsorbed onto these positive charge-occupied surfaces. Then the tetrabutylammonium cations were adsorbed around the amino acid anions, through an electrostatic attraction, leading to the formation of an AAIL adsorbed layer. Subsequently, other AAIL layers may be adsorbed around the initial adsorbate via electrostatic attraction to form fast physical adsorption films, preventing metal–metal contact and further reducing friction and wear.
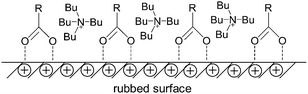 |
| Fig. 7 Schematic representation of an AAIL adsorbed layer. | |
4 Conclusions
We have designed a new family of green fluid lubricants (AAILs) for the lubrication of steel/steel, steel/copper and steel/aluminum contacts at RT. These AAILs can be obtained by simply neutralizing amino acids – which can be easily obtained in large quantities at low cost – with the corresponding onium hydroxide, such as [N4444][OH]. The preparation process is simple and provides no significant by-products except water, so it can significantly reduce the cost associated with lubricant purification. Use of natural amino acids as component ions makes the AAILs environmentally friendly with good biodegradability and reduced toxicity, making the AAILs good potential green lubricants. The hydrolysis stabilities of these AAILs are much higher than that of [C4mim][BF4] and the anti-corrosion properties of the AAILs are also far better than [C4mim][BF4] and [C6mim][NTf2], due to their halogen-free characters. The synthesized AAILs were found to have excellent friction-reducing and anti-wear properties for lubrication of all the contacts used. XPS analysis suggests that the lubrication mechanism of these AAILs is attributed the formation of fast physical adsorption films rather than tribochemical reaction films on the metal surfaces during the rubbing process, and these contribute to the low friction and wear of the AAILs. Consequently, our investigation strongly suggests the advantages of using AAILs as green lubricants. They are expected to be ideal candidates for future industrial lubricant fluids.
List of abbreviations
AAILs | Amino acid ionic liquids |
[N4444][Ser] | Tetrabutylammonium serine |
[N4444][Thr] | Tetrabutylammonium threonine |
[N4444][Val] | Tetrabutylammonium valine |
[N4444][Leu] | Tetrabutylammonium leucine |
[N4444][Lys] | Tetrabutylammonium lysine |
[N4444][OH] | Tetrabutylammonium hydroxide |
RT | Room temperature |
[C4mim][BF4] | 1-Butyl-3-methylimidazolium tetrafluoroborate |
[C6mim][NTf2] | 1-Hexyl-3-methylimidazolium bis(trifluoromethylsulfonyl)imide |
PAO40 | Poly-alpha-olefin |
XPS | X-ray photoelectron spectrometry |
IL | Ionic liquid |
NMR | Nuclear magnetic resonance spectroscopy |
MC | Moisture content |
η | Kinematic viscosity |
VI | Viscosity index |
TGA | Thermogravimetric analysis |
DSC | Differential scanning calorimetry |
Tonset | Onset decomposition temperature |
Tg | Glass transition temperature |
Acknowledgements
This research was supported by the “973” Program (2013CB632301), NSFC (51105353, 21173243, 21125316).
References
- M. Palacio and B. Bhushan, Tribol. Lett., 2010, 40, 247–268 CrossRef CAS PubMed.
- C. F. Ye, W. M. Liu, Y. X. Chen and L. G. Yu, Chem. Commun., 2001, 2244–2245 RSC.
- W. M. Liu, C. F. Ye, Q. Y. Gong, H. Z. Wang and P. Wang, Tribol. Lett., 2002, 13, 81–85 CrossRef CAS.
- H. Zhao, Chem. Eng. Commun., 2006, 193, 1660–1677 CrossRef CAS.
- F. Zhou, Y. M. Liang and W. M. Liu, Chem. Soc. Rev., 2009, 38, 2590–2599 RSC.
- I. Minami, Molecules, 2009, 14, 2286–2305 CrossRef CAS PubMed.
- M.-D. Bermúdez, A.-E. Jiménez, J. Sanes and F.-J. Carrión, Molecules, 2009, 14, 2888–2908 CrossRef PubMed.
- A. E. Jiménez, M. D. Bermúdez, P. Iglesias, F. J. Carrión and G. Martínez-Nicolás, Wear, 2006, 260, 766–782 CrossRef PubMed.
- M. R. Cai, Z. Zhao, Y. M. Liang, F. Zhou and W. M. Liu, Tribol. Lett., 2010, 40, 215–224 CrossRef CAS.
- M. J. Fan, Y. M. Liang, F. Zhou and W. M. Liu, RSC Adv., 2012, 2, 6824–6830 RSC.
- T. Torimoto, T. Tsuda, K.-I. Okazaki and S. Kuwabata, Adv. Mater., 2010, 22, 1196–1221 CrossRef CAS PubMed.
- Z. H. Song, Y. M. Liang, M. J. Fan, F. Zhou and W. M. Liu, Friction, 2013, 1, 222–231 CrossRef PubMed.
- A. Hernández Battez, R. González, J. L. Viesca, D. Blanco, E. Asedegbega and A. Osorio, Wear, 2009, 266, 1224–1228 CrossRef PubMed.
- K. Fukumoto, M. Yoshizawa and H. Ohno, J. Am. Chem. Soc., 2005, 127, 2398–2399 CrossRef CAS PubMed.
- J. Tong, M. Hong, Y. Chen, H. Wang, W. Guan and J. Z. Yang, J. Chem. Thermodyn., 2012, 54, 352–357 CrossRef CAS PubMed.
- G. H. Tao, L. He, W. S. Liu, L. Xu, W. Xiong, T. Wang and Y. Kou, Green Chem., 2006, 8, 639–646 RSC.
- Q. P. Liu, X. D. Hou, N. Li and M. H. Zong, Green Chem., 2012, 14, 304–307 RSC.
- K. Fukumoto and H. Ohno, Chem. Commun., 2006, 3081–3083 RSC.
- J. Ding and D. W. Armstrong, Chirality, 2005, 17, 281–292 CrossRef CAS PubMed.
- K. Fukumoto, Y. Kohno and H. Ohno, Chem. Lett., 2006, 35, 1252–1253 CrossRef CAS.
- X. W. Chen, X. H. Li, A. X. Hu and F. R. Wang, Tetrahedron: Asymmetry, 2008, 19, 1–14 CrossRef CAS PubMed.
- H. Ohno and K. Fukumoto, Acc. Chem. Res., 2007, 40, 1122–1129 CrossRef CAS PubMed.
- Y. Y. Jiang, G. N. Wang, Z. Zhou, Y. T. Wu, J. Geng and Z. B. Zhang, Chem. Commun., 2008, 505–507 RSC.
- P. Bonhôte, A.-P. Dias, N. Papageorgiou, K. Kalyanasundaram and M. Grätzel, Inorg. Chem., 1996, 35, 1168–1178 CrossRef PubMed.
- A. S. Pensado, M. J. P. Comuñas and J. Fernández, Tribol. Lett., 2008, 31, 107–118 CrossRef CAS.
- F. M. Gaciño, T. Regueira, L. Lugo, M. J. P. Comuñas and J. Fernández, J. Chem. Eng. Data, 2011, 56, 4984–4999 CrossRef.
- J. Salgado, M. Villanueva, J. J. Parajó and J. Fernández, J. Chem. Thermodyn., 2013, 65, 184–190 CrossRef CAS PubMed.
- P. Wasserscheid, R. van Hal and A. Bösmann, Green Chem., 2002, 4, 400–404 RSC.
- X. Q. Liu, F. Zhou, Y. M. Liang and W. M. Liu, Tribol. Lett., 2006, 23, 191–196 CrossRef CAS PubMed.
- J. Qu, P. J. Blau, S. Dai, H. M. Luo, H. M. Meyer and J. J. Truhan, Wear, 2009, 267, 1226–1231 CrossRef CAS PubMed.
- I. Minami and S. Mori, Lubr. Sci., 2007, 19, 127–149 CrossRef CAS.
Footnote |
† Electronic supplementary information (ESI) available: Characterization data of 1H NMR and 13C NMR spectra for the synthesized AAILs are presented. See DOI: 10.1039/c3ra47644h |
|
This journal is © The Royal Society of Chemistry 2014 |
Click here to see how this site uses Cookies. View our privacy policy here.