DOI:
10.1039/C3RA47720G
(Paper)
RSC Adv., 2014,
4, 12441-12447
Synthesis and evaluation of 2-heteroaryl and 2,3-diheteroaryl-1,4-naphthoquinones that potently induce apoptosis in cancer cells†
Received
17th December 2013
, Accepted 13th February 2014
First published on 18th February 2014
Abstract
This article describes the preparation of 2-heteroaryl and 2,3-diheteroaryl-1,4-naphthoquinones by an environmentally benign short synthetic route with the goal of finding 1,4-naphthoquinone derivatives that induce apoptosis in cancer cells. We have identified three most active naphthoquinones 10, 12 and 15 that potently induce apoptosis in human cervical carcinoma (HeLa) cells. One of these three compounds perturbed both microtubule and actin filaments.
Introduction
During recent years the biological effects of 2-heteroaryl and 2,3-diheteroaryl-1,4-naphthoquinones have led to synthesis of 1,4-naphthoquinone derivatives by nucleophilic substitution and addition reactions in non aqueous medium leading to a mixture of products and lower yields.1–10 This prompted us to devise a new synthetic route for regioselective synthesis of 2-heteroaryl and 2,3-diheteroaryl-1,4-naphthoquinone derivatives in high yields by carrying out nucleophilic substitution reactions “in-H2O” using micelles as a catalyst which has not been reported earlier for the title compounds.
Naphthoquinones have a wide spectrum of anticancer activity.11 They covalently bind and intercalate into DNA, which leads to DNA breakage and chromosomal aberration.12,13 Naphthoquinones are also able to modulate receptor tyrosine kinase activity.14,15 Recently naturally occurring 1,4-naphthoquinone derivatives16 as well as synthetic 1,4-naphthoquinones17 have been found to induce apoptosis in HL-60 (human promyelocytic leukemia cells) and HT29 (human colon adenocarcinoma cell line).
One of the recent approaches in drug design which includes pharmacophore modelling and three dimensional quantitative structure–activity relationship (3D-QSAR)/comparative molecular similarity indices analysis (CoMSIA) methods applied to 2,3-disubstituted-1,4-naphthoquinones and heterocyclic quinones possessing nitrogen and sulfur atoms have unraveled the pronounced cytotoxic effects of these compounds.18,19
Results and discussion
In the course of a medicinal chemistry program aimed at discovering 1,4-naphthoquinone derivatives containing nitrogen and sulfur atoms endowed with cytotoxic activity, we have synthesized a series of 2-chloro-3-(sec/tert-arylamino)-naphthalene-1,4-diones 10–13, 2,3-diarylaminonaphthalene-1,4-dione 14, 2-amino-3-arylsulfanyl-naphthalene-1,4-diones 15, 16 and 2,3-diheteroaryl-naphthalene-1,4-diones 17–23.
Recently organic reactions performed in water have attracted considerable attention with respect to unique property of water in promoting reactions and enhancing selectivity.20 The surfactant-type catalyst plays a dual role both as a catalyst to activate the substrate molecules and as a surfactant to increase the concentration of organic reactions to form micelle particles in water which have been extensively used.21,22
The synthesis of 1,4-naphthoquinone derivatives 10–23 in water was performed as described in Scheme 1. Nucleophilic substitution reaction of 2,3-dichloro-1,4-naphthoquinoe 1 with primary and secondary aryl amines 3–6 in H2O using Et3N as a base and LD or SDS23,24 as catalyst resulted in the regioselective synthesis of 2-chloro-3(sec/tert-arylamino)-naphthalene-1,4-diones 10–13 in 74–99% yield. The reaction of 1 with 2-aminobenzonitrile 6 (2 eq.) under identical conditions afforded 14 regioselectively in moderate yield. 2-Chloro-3-amino-1,4-naphthoquinone 2 on nucleophilic substitution reaction with aryl thiol 7 and 9 (1 eq.) under identical conditions resulted in the formation of 15 and 16 respectively in excellent yields. In order to synthesize 2,3-diheteroaryl-naphthalene-1,4-diones 17–23; 10–13 were reacted with aryl thiols 7–9 under identical conditions to afford 17–23 in excellent yields.
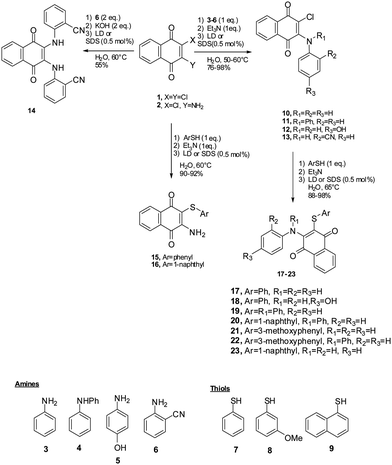 |
| Scheme 1 Synthesis of 1,4-naphthoquinone derivatives 10–23. | |
The use of surfactants LD or SDS invariably leads to almost identical yields of products 10–23. It is pertinent to note that use of LD or SDS as a catalyst in presence of Et3N results in excellent yields of 10–23. The absence of catalyst results in poor yields of 10–23 (0–25%) in presence of bases (KOH, Et3N etc.). In the presence of LD or SDS “in H2O”, micelles25 are formed and are responsible for observed catalytic influence during the course of reactions outlined in Scheme 1. The synthesis of 1,4-naphthoquinone derivatives 10–23 carried out by us in non aqueous solvents is uneconomical and involves longer duration of time for completion of reaction.26,27
The antiproliferative activities of compounds 10–23 were tested against HeLa cells proliferation at 5 μM. At 5 μM, compounds 10–23 showed different levels of inhibition on HeLa cells proliferation (Table 1). The compounds 10, 12 and 15 were found to inhibit HeLa cells proliferation more than 80% suggesting that these compounds have most potent activity among the synthesized derivatives (Table 1).
Table 1 Mean% inhibition of HeLa cells proliferation by naphthoquinone series of compounds at 5 μM concentration
Compounds 10, 12 and 15 were selected for further studies and were found to inhibit HeLa cells proliferation in a concentration dependent manner. Half maximal inhibitory concentrations (IC50) of compounds 10, 12 and 15 were determined by SRB assay28–33 and their IC50 was found to be 3.9 ± 0.3, 3.2 ± 0.7, and 3.7 ± 1 μM, respectively (Table 2). Under similar conditions, cisplatin, a widely used anticancer agent, inhibits the proliferation of HeLa cells with IC50 value of 8 ± 1 μM.33
Table 2 IC50 values of compounds 10, 12 and 15 in HeLa cells
Compound |
IC50 (μM) |
10 |
3.9 ± 0.3 |
12 |
3.2 ± 0.7 |
15 |
3.7 ± 1 |
Cisplatin33 |
8 ± 1 |
Regarding Structure–Activity Relationship (SAR) among 10, 11 and 12, replacement of H by the bulky phenyl substituent leads to considerable decrease in mean% inhibition of HeLa cells proliferation. The introduction of OH group leads to enhancement of mean% inhibition of HeLa cells proliferation leading to identification of 12 as the most active compound of the series. The SAR among 14–22 leads to infer that replacement of H by phenyl and naphthyl leads to decrease in activity. Thus in general the presence of larger number of bulky groups in compounds 10–22 lead to decrease in antiproliferative activity.
The structures of compound 12 and compound 10 are quite similar, so we have chosen compound 12 for further analysis as its cytotoxicity on HeLa cell proliferation was comparatively higher than that of the compound 10. Apoptosis is considered to be an important mechanism of cancer cell death. Therefore, we performed Annexin V/PI staining to determine the effects of compounds 12 and 15 on apoptosis.29,30 Compound 12 significantly increased the Annexin V positive cell population as compared to control (Fig. 1). The number of Annexin V positive cells was almost double the number of PI positive cells suggesting that cell death occurred through apoptosis (Table 3).
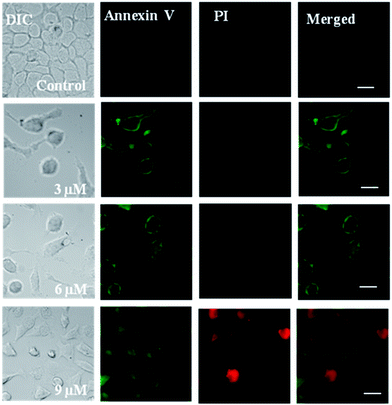 |
| Fig. 1 Annexin V and PI staining in the presence of compound 12 (scale bar 10 μm). | |
Table 3 % of Annexin V and PI positive cells in the presence of compound 12
Compound 12 (μM) |
% Cells with Annexin V staining |
% Cells with PI staining |
0 |
2 |
1 |
3 |
17 |
7 |
6 |
30 |
12 |
9 |
43 |
21 |
Compound 15 also increased the number of Annexin V positive cells (Table 4) but the effect was less pronounced as compared to compound 12.
Table 4 % of Annexin V and PI positive cells in the presence of compound 15
Compound 15 (μM) |
% Cells with Annexin V staining |
% Cells with PI staining |
0 |
2.2 |
1 |
4 |
10 |
6 |
8 |
25 |
9 |
12 |
33 |
12 |
Thus, Annexin V/PI staining results suggest that compound 12 is most potent apoptosis causing derivative among the synthesized naphthoquinone series of compounds 10–23.
Upon initiation of apoptosis, caspase 3 is known to be activated and translocated to the nucleus.31 Thus, the determination of caspase 3 localization can indicate the activation of cell death via apoptosis. Both compound 12 (Fig. 2) and compound 15 caused an increase in the nuclear localization of caspase 3 in the HeLa cells (Tables 5 and 6). However, the effect of compound 15 was lesser as compared to compound 12 (Tables 5 and 6).
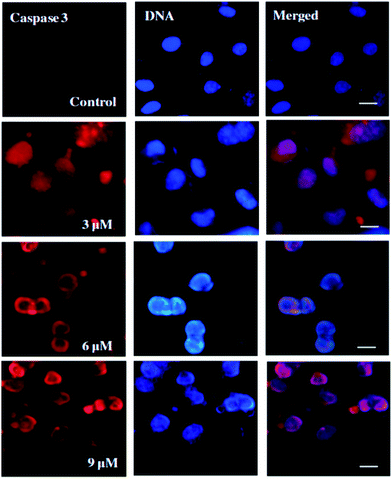 |
| Fig. 2 Increased caspase-3 nuclear localization in presence of compound 12 (scale bar 10 μm). | |
Table 5 Increase in caspase 3 localisation in presence of compound 12
Compound 12 (μM) |
% Cells with caspase 3 staining |
0 |
2 ± 1 |
3 |
15 ± 3 |
6 |
25 ± 2 |
9 |
41 ± 3 |
Table 6 Increase in caspase 3 localisation in presence of compound 15
Compound 15 (μM) |
% Cells with caspase 3 staining |
0 |
2 ± 1 |
4 |
12 ± 4 |
8 |
20 ± 2 |
12 |
27 ± 3 |
Further, we investigated the effects of compound 12 and 15 on HeLa cell cycle progression by determining mitotic index32 (Fig. 3) and phosphohistone staining.30 In control, 3% cells were found to be in mitosis whereas 11.7 ± 0.4% and 8.3 ± 0.3% cells were present in mitosis in presence of 6 μM compound 12 and compound 15, respectively. Compound 12 and 15 treatment also increased the number of phosphohistone positive cells. For example, 3.2 ± 0.3% control HeLa cells displayed phosphohistone staining while 13.3 ± 1% and 12.7 ± 5% cells showed phosphohistone staining in the presence of 9 μM compound 12 and 20 μM compound 15, respectively.
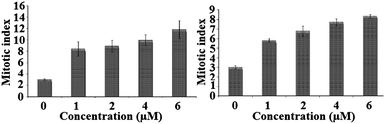 |
| Fig. 3 Effects of compound 12 & 15 on the mitotic index in HeLa cells. | |
Furthermore, upon short exposure of compound 12 to HeLa cells, microtubules and actin network got disassembled, while short exposure of compound 15 did not seem to cause any effect on microtubule and actin network. The actin network of 20 μM compound 12 treated HeLa cells was disassembled in a similar fashion as 60 μM cytochalasin D, a known actin depolymerizing agent. Compound 12 also caused an increase in the cyclin B1 staining33 (Table 7).
Table 7 Compound 12 treatment increased nuclear localization of cyclin B1
Concentration (μM) |
Cyclin B1 positive cells (%) |
0 |
3.3 |
3 |
11.5 |
6 |
15.2 |
9 |
17.3 |
The redox potential being the most significant physicochemical property determining the cytotoxicity of naphthoquinone derivatives, the reactive oxygen species (ROS) formed due to quinone redox cycling seem to be relevant to cytotoxicity of 1,4-naphthoquinone derivatives in addition to their hydrophobicities.10
Conclusion
In summary, we have identified a potent 1,4-naphthoquinone derivative 12 that induces apoptosis in HeLa cells as well as environmentally benign synthetic route for the synthesis of compound 12 and other derivatives has been developed. We are currently studying the details of selectivity of the potent naphthoquinone derivatives that induce apoptosis in HeLa cells.
Experimental section
General
1H and 13C NMR spectra were recorded on Perkin Elmer model R.32 spectrophotometers (300 MHz H, 75 MHz C) in deuterated chloroform (CDCl3) or deuterated dimethylsulfoxide (DMSO-d6). The data is reported as follows: chemical shifts in ppm (δ), multiplicities are indicated as s-singlet; d-doublet; t-triplet; q-quartet; m-multiplet, br-broad. Coupling constants, J, are reported in Hz. Infrared spectra were recorded on FTIR 8201 PC, Schimadzu spectrophotometers in KBr discs, and the peaks reported in cm−1. All compounds showed satisfactory elemental analysis for C, H, N and S. Progress of reactions and purity of compounds were monitored by thin layer chromatography (TLC), which was performed on silica gel G and the plates were visualized by visible, UV light and iodine (I2). Spectral facilities and elemental micro-analyses were carried out by SAIF division of Central Drug Research Institute, Lucknow, India. Melting points were determined on an electrically heated Townson Mercer melting point apparatus and are uncorrected. Chemicals and reagents were purchased from Aldrich and used as received.
General procedure for the synthesis of 1,4-naphthoquinone derivatives (10–23)
1 mmol nucleophile (3–6 or 7–9) was added to 5 mL aqueous suspension of surfactant (0.5 mol% LD) and Et3N–KOH (1 mmol). After stirring for five minutes, quinone 1/2/10–13 (1 mmol/2 mmol) was added and stirred at temperature (Table 2, ESI†). The products were filtered after neutralization with 5% HCl, if required, followed by column chromatography (hexane–EtOAc) of the product to yield the desired products (10–23): (Table 2, ESI†).
2-Chloro-3-(phenylamino)naphthalene-1,4-dione (10)26,27. Red powder from methanol; yield 99%; mp: 210–211 °C; IR (KBr): 1597 and 1675 (>C
O of quinone), 3243 (N–H) cm−1; 1H NMR (400 MHz, CDCl3): δ 8.19 (d, J = 8.0 Hz, 1H), 8.12 (d, J = 8.0 Hz, 1H), 7.77 (t, J = 8.0 Hz, 1H), 7.69 (t, J = 8.0, 1H), 7.35 (m, 3H), 7.22 (t, J = 7.6, 1H), 7.09 (d, J = 7.6, 2H). 13C NMR (100 MHz, CDCl3 + DMSO-d6): δ 115.1, 124.7, 125.9, 127.3, 127.3, 128.7, 130.2, 132.9, 133.3, 135.3, 137.9, 142.1, 177.8, 180.8. Anal. calcd for C16H10ClNO2 (284): C, 67.74; H, 3.55; N, 4.94. Found: C, 67.10; H, 3.53; N, 4.91; Beilstein test:34 Cl positive.
2-Chloro-3-(diphenylamino)naphthalene-1,4-dione (11)26,27. Yellow fine needle shaped crystals from methanol which on exposure in air changed color yellow to fluorescent light green; yield 62%; mp: 130–132 °C IR (KBr): 1592 and 1678 (>C
O of quinone) cm−1; 1H NMR (400 MHz, CDCl3): δ 8.22 (m, 1H), 8.15 (m, 3H), 8.09 (m, 3H), 7.81 (m, 1H), 7.75 (m, 6H); 13C NMR (100 MHz, CDCl3): δ 127.3, 127.3, 128.7, 131.2, 131.4, 134.3, 134.7, 157.2, 179.9, 180.1; MS: 360 (M+). Anal. calcd for C22H14ClNO2 (360): C, 73.44; H, 3.92; N, 3.89; found: C, 72.96; H, 3.98; N, 3.92; Beilstein test:34 Cl positive.
2-Chloro-3-(4-hydroxyphenylamino)naphthalene-1,4-dione (12)26,27. Violet powder from methanol; yield 96%; mp: 181 °C; IR (KBr): 1593 and 1678 (>C
O of quinone) 3269 (N–H), 3468 (O–H) cm−1; 1H NMR (400 MHz, CDCl3): δ 8.19 (d, J = 7.6, 1H), 8.12 (d, J = 7.6, 1H), 7.90 (s, 1H), 7.75 (t, J = 7.6, 1H), 7.69 (t, J = 7.6, 1H), 7.35 (d, 2H), 7.05 (d, 2H), 4.75 (s, 1H). 13C NMR (100 MHz, CDCl3 + acetone-d6): δ 112.1, 114.4, 126.2, 126.9, 127.0, 129.3, 129.4, 131.9, 134.3, 139.0, 142.0, 155.51, 179.9, 180.1; anal. calcd for C16H10ClNO3 (300): C, 64.12; H, 3.36; N, 4.67; found: C, 63.92; H, 3.42; N, 4.72; Beilstein test:34 Cl positive.
2-3-Chloro-1,4-dioxo-1,4-dihydronaphthalen-2-ylaminobenzonitrile (13)26,27. The general procedure was followed for 24 h to give orange crystals on crystallization with EtOAc–hexane; yield 76%; mp: 233–234 °C; IR (KBr): 554, 680, 734, 836, 879, 1002, 1158, 1229, 1274, 1380, 1478, 1539, 1591, 1679, 2364, 3450, cm−1; 1H NMR (CDCl3): δ 7.08 (d, J = 7.6 Hz, 1H), 7.31 (d, J = 7.6 Hz, 1H), 7.56–7.82 (m, 4H), 7.67 (s, 1H) 8.19 (dd, J = 7.6 Hz, 1.0 Hz, 2H); 13C NMR (CDCl3): 99.6, 113.3, 117.1, 118.3, 119.4, 127.3, 128.8, 130.1, 131.3, 133.9, 134.2, 135.1, 136.6, 151.7, 152.2, 178.3, 179.1; MS: 309 (MH+); anal. calcd (C17H9ClN2O2): C, 66.14; H, 2.94; N, 9.07; found: C, 65.85; H, 2.70; N, 8.94; Beilstein test:34 Cl positive.
2,2′-(1,4-Dioxo-1,4-dihydronaphthalene-2,3-diyl)bis(azanediyl)dibenzonitrile (14)26,27. Dark red solid after crystallization with EtOAc; yield 55%; mp 258–259 °C; IR (KBr): 505, 617, 645, 716, 761, 793, 851, 912, 1016, 1095, 1135, 1241, 1287, 1332, 1383, 1469, 1509, 1598, 1677, 2222, 2366, 3265, 3416 cm−1; 1H NMR (CDCl3 + DMSO-d6): δ 6.72–7.32 (m, 6H), 7.67–7.80 (m, 6H), 8.15 (dd, J = 5.5 Hz, 3.0 Hz, 2H); 13C NMR (100 MHz, CDCl3 + DMSO-d6): δ 99.1, 118.5, 119.0, 119.8, 120.8, 126.9, 131.9, 134.8, 135.4, 137.0, 151.2, 181.8; MS: M+ (M+ + 1): 391; anal. calcd (C24H14N4O2): C, 73.84; H, 3.61; N, 14.35; found: C, 74.02; H, 3.82; N, 14.60.
2-Amino-3-phenylsulfanyl-[1,4]naphthoquinone (15)26,27. Red crystals after crystallization with methanol; yield 92%; mp 160–161 °C; IR (KBr): 1598 and 1676 (>C
O of quinone), 3243 and 3462 (NH2) cm−1; 1H NMR (300 MHz, CDCl3): δ 8.08 (m, 2H), 7.65 (m, 2H), 7.38 (m, 2H), 7.26 (s, 3H), 7.09 (bs, 2H); 13C NMR (100 MHz, CDCl3 + DMSO-d6): δ 126.0, 126.9, 127.1, 127.3, 129.5, 131.1, 132.9, 134.2, 135.5, 136.5, 153.9, 179.3, 180.7; anal. calcd for C16H11NO2S (281): C, 68.31; H, 3.94; N, 4.98; S, 11.40; found: C, 67.98; H, 3.88; N, 4.93; S, 11.35.
2-Amino-3-(naphthalen-1-ylsulfanyl)-[1,4]naphthoquinone (16)26,27. Brown powder from methanol; yield 90%; mp 170–171 °C; IR (KBr): 1585 and 1662 (>C
O of quinone), 3270 and 3440 (NH2) cm−1; 1H NMR (300 MHz, CDCl3): δ 8.17 (m, 2H), 7.98 (m, 2H), 7.77 (m, 4H), 7.50 (m, 3H), 7.30 (bs, NH2); 13C NMR (100 MHz, CDCl3 + DMSO-d6): δ 125.0, 126.0, 126.1, 126.9, 127.1, 127.1, 127.5, 128.2, 128.9, 129.0, 131.2, 131.9, 133.0, 134.0, 134.2, 134.3, 135.5, 153.9, 179.4, 180.7; anal. calcd for C20H13NO2S (331): C, 72.49; H, 3.95; N, 4.23; S, 9.68; found: C, 71.92; H, 3.92; N, 4.27; S, 9.60.
2-Phenylamino-3-(phenylthio)naphthalene-1,4-dione (17)26,27. Brown solid from methanol; yield 99%; mp 143–145 °C; IR (KBr): 1589 and 1664 (>C
O of quinone), 3265 (N–H) cm−1; 1H NMR (400 MHz, CDCl3): δ 8.18 (d, J = 7.6, 1H), 8.13 (d, J = 7.6, 1H), 7.93 (bs, 1H), 7.76 (t, J = 7.6, 1H), 7.69 (t, J = 7.6, 1H), 7.17–7.15 (t, 2H), 7.13–6.98 (m, 4H), 6.75–6.68 (m, 4H); anal. calcd for C22H15NO2S (357): C, 73.93; H, 4.23; N, 3.92; S, 8.97; found: C, 74.22; H, 4.21; N, 3.98; S, 9.03.
2-(4-Hydroxyphenylamino)-3-(phenylthio)naphthalene-1,4-dione (18)26,27. Shining brown solid from methanol; yield 88%; mp 220–221 °C; IR (KBr): 1546 and 1624 (>C
O of quinone), 3315 (N–H), 3375 (O–H) cm−1; 1H NMR (400 MHz, CDCl3): δ 8.18 (d, J = 7.2, 1H), 8.12 (d, J = 7.2, 1H), 7.90 (bs, 1H), 7.68–7.76 (m, 3H) 7.31 (m, 1H), 7.03–7.05 (m, 3H), 6.77–6.80 (m, 2H), 6.62 (m, 3H); anal. calcd for C22H15NO3S (373): C, 70.76; H, 4.05; N, 3.75; S, 8.59; found: C, 70.02; H, 4.01; N, 3.70; S, 8.63.
2-(Diphenylamino)-3-(phenylthio)naphthalene-1,4-dione (19)26,27. Red oil; yield 90%; IR (KBr): 1590 and 1678 (>C
O of quinone) cm−1; 1H NMR (400 MHz, CDCl3): δ 8.04–8.08 (m, 4H), 7.94–7.98 (m, 2H), 7.60–7.80 (m, 4H), 7.43–7.49 (m, 3H), 7.02–7.11 (m, 3H), 6.60–6.69 (m, 3H); 13C NMR (100 MHz, CDCl3): δ 127.0, 127.3, 127.6, 127.8, 128.0 (4C), 129.4, 129.5, 130.7, 131.7, 131.8, 134.0, 134.4, 137.5, 178.5, 180.8. anal. calcd for C28H19NO2S (434): C, 77.57; H, 4.42; N, 3.23; S, 7.40; found: C, 77.42; H, 4.38; N, 3.18; S, 7.45.
2-(Diphenylamino)-3-(naphthalene-1-ylthio)naphthalene-1,4-dione (20)26,27. Yellow powder from methanol; yield 98%; mp: 120–121 °C; IR (KBr): 1590 and 1678 (>C
O of quinone) cm−1; 1H NMR (400 MHz, CDCl3): δ 8.04–8.08 (m, 4H), 7.94–7.7.98 (m, 2H), 7.60–7.80 (m, 9H), 7.43–7.49 (m, 6H); 13C NMR (100 MHz, CDCl3 + DMSO-d6): δ 125.6, 125.8, 125.9, 126.0, 126.6, 127.0, 127.5, 127.7, 128.3, 128.6, 130.0, 130.6, 130.7, 131.3, 131.5, 132.8, 133.0, 133.3, 158.5, 178.8, 181.1; anal. calcd for C32H21NO2S (484): C, 79.48; H, 4.38; N, 2.90; S, 6.63; found: C, 78.98; H, 4.34; N, 2.92; S, 6.58.
2-(3-Methoxyphenylthio)-3-(phenylamino)naphthalene-1,4-dione (21)26,27. Shining brown solid from methanol; yield 99%; mp: 143–145 °C; IR (KBr): 1587 and 1662 (>C
O of quinone), 2359 and 2925 (OMe), 3310 (N–H) cm−1; 1H NMR (400 MHz, CDCl3): δ 8.18 (d, J = 7.6, 1H), 8.13 (d, J = 7.6, 1H), 7.96 (bs, 1H), 7.77 (t, J = 7.6, 1H), 7.69 (t, J = 7.6, 1H) 7.19–7.17 (t, 2H, Ar-H), 7.15–7.09 (t, 1H, Ar-H), 6.96–6.92 (t, 1H, Ar-H), 6.73–6.71 (d, 2H, Ar-H), 6.60–6.58 (d, 1H, Ar-H), 6.38–6.39 (d, 1H), 6.22 (s, 1H, Ar-H), 3.63 (s, 3H, OCH3), mass (Fab): M+ = 387, M+ + 1 = 388, M+ + 2 = 389; 13C NMR (400 MHz, CDCl3): δ 55.0, 112.0, 112.7, 113.0, 114.1, 120.6, 123.0, 124.6, 126.7, 127.0, 127.7, 129.0, 130.3, 132.7, 133.3, 134.7, 136.3, 142.6, 159.3, 180.7, 181.0; anal. calcd for C23H17NO3S (387): C, 71.30; H, 4.42; N, 3.62; S, 8.28; found: C, 71.24; H, 4.39; N, 3.58; S, 8.32.
2-(Diphenylamino)-3-(3-methoxyphenylthio)naphthalene-1,4-dione (22)26,27. Red oil; yield 95%; IR (KBr): 1588 and 1662 (>C
O of quinone), 2359 and 2925 (OCH3) cm−1; 1H NMR (400 MHz, CDCl3): δ 8.03–8.07 (m, 4H), 7.92–7.96 (m, 2H), 7.59–7.78 (m, 4H), 7.43–7.45 (m, 2H), 7.02–7.11 (m, 3H), 6.62–6.70 (m, 3H), 3.73 (s, 3H, OCH3). Anal. calcd for C29H21NO3S (464): C, 75.14; H, 4.57; N, 3.02; S, 6.92; found: C, 75.42; H, 4.58; N, 3.08; S, 7.00.
2-(Naphthalene-1-ylthio)-3-(phenylamino)naphthalene-1,4-dione (23)26,27. Light brown solid from methanol; mp: 119–120 °C; yield 96%; IR (KBr): 1590 and 1662 (>C
O of quinone), 3329 (N–H) cm−1; 1H NMR (400 MHz, CDCl3): δ 8.04–8.08 (m, 4H), 7.94–7.98 (m, 2H), 7.68–7.80 (m, 4H), 7.43–7.49 (m, 3H) 7.32–7.26 (m, 2H), 6.62–6.56 (m, 2H); 13C NMR (100 MHz, CDCl3 + DMSO-d6): δ 123.29, 125.15, 126.03, 126.08, 126.60, 126.75, 127.71, 127.95, 128.82, 130.89, 131.48, 133.00, 133.36, 133.73, 133.87, 135.28, 136.87, 141.93, 143.13, 181.24, 181.60; anal. calcd for C26H17NO2S (407): C, 76.64; H, 4.21; N, 3.44; S, 7.87; found: C, 75.92; H, 4.19; N, 3.41; S, 7.91.
Evaluation of anticancer activity
SRB assay. Human cervical carcinoma (HeLa) cells were cultured in Minimal Essential medium (Hi Media) containing 10% fetal bovine serum.35 For cell proliferation assay, 1 × 105 cells per mL were seeded in 96 well plates and for microscopic studies, 0.5 × 105 cells per mL were grown as monolayer on glass cover slips.35 After 24 hours of seeding, 5 μM of each of the compounds tested was added to the wells and control cells were treated with vehicle (0.1% DMSO). Concentration of DMSO was kept 0.1% in both control and treated cells. Cells were incubated with the compounds for 24 hours and then, sulforhodamine B assay was performed.28 The half maximal inhibitory concentration of 10, 12 and 15 compounds on HeLa cell proliferation was determined after one cell cycle using sulforhodamine B assay.28
Annexin/PI and caspase 3 staining. HeLa cells were incubated with either vehicle or different concentrations of compound 12 (3 μM, 6 μM, 9 μM) and compound 15 (4 μM, 8 μM, 12 μM) for 24 h. Then, cells were stained with FITC labeled Annexin V and propidium iodide.29,30 The cells were observed with an Eclipse TE 2000U microscope (Nikon, Japan) at 40X magnification. The images were analyzed using Image-Pro Plus software (Media Cybernetics, Silver Spring, MD, USA).29,30 HeLa cells were incubated in the absence or presence of different concentrations of compound 12 (0, 3, 6 and 9 μM) and compound 15 (0, 4, 8 and 12 μM) for 24 hours. Then, cells were fixed with 3.7% (v/v) formaldehyde and caspase 3 staining was performed using anticaspase 3 antibody (1
:
200 dilution). The coverslips were washed with BSA–PBS at room temperature and then incubated with secondary antibody (anti-mouse Alexa-568 conjugate) for 1 h at 1
:
200 dilution. To visualize nuclei, cells were stained with Hoechst 33258 (1 μg mL−1). Immuno-stained cells were examined using 40X objective.
Determination of mitotic index. HeLa cells were treated without or with different concentrations of compound 12 and compound 15 for 24 hours. The mitotic index was determined by staining DNA with Hoechst 33258 (1 μg mL−1) for 10 min as described previously.32 500 cells were counted for each data set. HeLa cells were incubated without or with 3, 6 and 9 μM compound 12 for 24 hours. Subsequently, cells were fixed with 3.7% (v/v) formaldehyde. The cyclin B1 staining was done by incubating cells with 1
:
300 dilution of anti-cyclin B1 antibody.33 To visualize nuclei, cells were stained with Hoechst 33258 (1 μg mL−1). Immuno-stained cells were examined using a 40X objective.32
Microtubule and actin staining. HeLa cells were incubated without or with either 20 μM of compound 12, or 20 μM of compound 15 for 2.5 h at 37 °C. After treatment, cells were fixed with 3.7% (v/v) formaldehyde. Microtubules, actin and DNA were stained as described previously.29 Immuno-stained cells were examined using a 40X objective.
Acknowledgements
H.K.M. acknowledges DST, New Delhi, India for DST, Fast Track Young Scientist Scheme, Sandeep Kumar is thankful to DST, New Delhi, India for INSPIRE Fellowship Award and Aijaz Rashid thanks U.G.C., New Delhi, India for Senior research fellowship. VKT thanks CSIR, Government of India for Emeritus Scientist. DP thanks DAE, Government of India for SRC fellowship.
Notes and references
- L. Salmon-Chemin, E. Buisine, V. Yardley, S. Kohler, M.-A. Debreu, V. Landry, C. Sergheraert, S. L. Croft, R. L. Krauth-Siegel and E. Davioud-Charvet, J. Med. Chem., 2001, 44, 548 CrossRef CAS PubMed.
- J. M. Miguel del Corral, M. A. Castro, M. Gordaliza, M. L. Martin, S. A. Gualbertu, A. M. Gamito, C. Cuevas and A. S. Feliciano, Bioorg. Med. Chem., 2005, 13, 631 CrossRef CAS PubMed.
- V. K. Tandon, R. B. Chhor, R. V. Singh, S. Rai and D. B. Yadav, Bioorg. Med. Chem. Lett., 2005, 15, 1079–1083 CrossRef PubMed.
- V. K. Tandon, D. B. Yadav, A. K. Chaturvedi and P. K. Shukla, Bioorg. Med. Chem. Lett., 2005, 15, 3288–3291 CrossRef CAS PubMed.
- V. K. Tandon, D. B. Yadav, R. V. Singh, M. Vaish, A. K. Chaturvedi and P. K. Shukla, Bioorg. Med. Chem. Lett., 2005, 15, 3463–3466 CrossRef CAS PubMed.
- V. K. Tandon, D. B. Yadav, R. V. Singh, A. K. Chaturvedi and P. K. Shukla, Bioorg. Med. Chem. Lett., 2005, 15, 5324–5328 CrossRef CAS PubMed.
- V. K. Tandon, H. K. Maurya, D. B. Yadav, A. Tripathi, M. Kumar and P. K. Shukla, Bioorg. Med. Chem. Lett., 2006, 16, 5883–5887 CrossRef CAS PubMed.
- V. K. Tandon, D. B. Yadav, H. K. Maurya, A. K. Chaturvedi and P. K. Shukla, Bioorg. Med. Chem., 2006, 16, 6120–6123 CrossRef PubMed.
- E. A. Louladouros, Z. F. Plyta and V. P. Papageorgiou, J. Org. Chem., 1996, 61, 3031–3033 CrossRef PubMed.
- Y.-S. Kim, S.-Y. Park, H. J. Lee, M. E. Suh, D. Schollmeyer and C. O. Lee, Bioorg. Med. Chem., 2003, 11, 1709–1714 CrossRef CAS.
- R. P. Verma, Anti-Cancer Agents Med. Chem., 2006, 6, 489–499 CrossRef CAS.
- A. G. Ravelo, A. Estevez-Braun, H. Chavez-Orellana, E. Perez-Sacau and D. Mesa-Siverio, Curr. Top. Med. Chem., 2004, 4, 241–265 CrossRef CAS.
- L. Garuti, M. Roberti and D. Pizzirani, Mini-Rev. Med. Chem., 2007, 7, 481–489 CrossRef CAS.
- H.-J. Kwiak, M.-J. Park, C.-M. Park, S.-I. Moon, D.-H. Yoo, H.-C. Lee, S.-H. Lee, M.-S. Kim, H.-W. Lee, W.-S. Shin, I.-C. Park, C.-H. Rhee and S. Hong, Int. J. Cancer, 2006, 118, 2711–2720 CrossRef PubMed.
- K. Nakaya and T. A. Miyasaka, Anticancer Drugs, 2003, 14, 683–693 CrossRef CAS PubMed.
- A. Kawiak, J. Zawacka-Pankau, A. Wasilewska, G. Stasilojc, J. Bigda and E. Lojkowska, J. Nat. Prod., 2012, 75, 9–14 CrossRef CAS PubMed.
- M. L. Bolognesi, N. Calonghi, C. Mangano, L. Masotti and C. Melchiorre, J. Med. Chem., 2008, 51, 5463–5467 CrossRef CAS PubMed.
- I. Gomez-Monterrey, G. Santelli, P. Campiglia, D. Califano, F. Falasconi, C. Pisano, L. Vesci, T. Lama, P. Grieco and E. Novellino, J. Med. Chem., 2005, 48, 1152–1157 CrossRef CAS PubMed.
- V. K. Tandon, H. K. Maurya, A. Tripathi, G. B. Shivakeshava, P. K. Shukla, P. Srivastava and D. Panda, Eur. J. Med. Chem., 2009, 44, 1086–1092 CrossRef CAS PubMed.
-
(a) C. I. Herrerias, Y. Xiaoquan and C.-J. Li, Chem. Rev., 2007, 107, 2546 CrossRef CAS PubMed;
(b) S. Narayan, J. Muldoon, M. G. Finn, V. V. Fokin, H. C. Kolb and K. B. Sharpless, Angew. Chem., Int. Ed., 2005, 44, 3275–3279 CrossRef CAS PubMed;
(c) V. K. Tandon and H. K. Maurya, Tetrahedron Lett., 2009, 50, 5896–5902 CrossRef CAS PubMed.
-
(a) M. Shiria and M. A. Zolfigolb, Tetrahedron, 2009, 65, 587–598 CrossRef PubMed;
(b) S. Tascioglu, Tetrahedron, 1996, 52, 11113–11152 CrossRef CAS.
- V. K. Tandon and H. K. Maurya, Tetrahedron Lett., 2010, 51, 3843–3847 CrossRef CAS PubMed.
-
(a) LD is a common laundry detergent (common washing powder used for washing of cloths in home) which contains alkylbenzenesulfonates. It is economically more viable than SDS (Sodium Dodecyl Sulfate), Triton X-100 or other costly surfactants. We preferred to use LD as surfactant. Laundry detergent (LD) as surfactant is Ariel, detergent product made in India by Proctor and Gambel home products Ltd. Mumbai 400099 (Tel. +91 2224942113, E-mail: E-mail: intouch.lm@pg.com) no.: 4902430214 933, MRP: rupees 2.00 packed 03/2009, net weight 14 g. The cost of SDS is Rs. 1123.00 for 25 g in the Sigma Aldrich catalog (436143-25G)-2008;
(b) In place of Ariel, other laundry detergents (washing powder) can also be used. For details refer to http://enwikipedia.org/wiki/Laundrydetergent.
- C. Biot, H. Bauer, R. H. Schirmer and E. Davioud-Charvet, J. Med. Chem., 2004, 47, 5972–5983 CrossRef CAS PubMed.
- U. M. Lindstrom, Chem. Rev., 2002, 102, 2751–2772 CrossRef PubMed.
- V. K. Tandon, H. K. Maurya, N. N. Mishra and P. K. Shukla, Eur. J. Med. Chem., 2009, 44, 3130–3137 CrossRef CAS PubMed.
- V. K. Tandon and H. K. Maurya, Heterocycles, 2008, 76, 1007–1010 CrossRef CAS PubMed.
- P. Skehan, R. Storeng, D. Scudiero, A. Monks, J. McMahon, D. Vistica, J. T. Warren, H. Bokesch, S. Kenney and M. R. Boyd, J. Natl. Cancer Inst., 1990, 82, 1107–1112 CrossRef CAS PubMed.
- S. Kapoor and D. Panda, Biochem. Pharmacol., 2012, 83, 1495–1506 CrossRef CAS PubMed.
- K. K. Gireesh, A. Rashid, S. Chakrabortti, D. Panda and T. Manna, Biochem. Pharmacol., 2012, 84, 633–645 CrossRef CAS PubMed.
- S. Kamada, U. Kikkawa, Y. Tsujimoto and T. Hunter, J. Biol. Chem., 2004, 280, 857–860 CrossRef PubMed.
- M. Banerjee, P. Singh and D. Panda, FEBS J., 2010, 277, 3437–3448 CrossRef CAS PubMed.
- S. Ray, R. Mohan, J. K. Singh, M. K. Samantaray, M. M. Shaikh, D. Panda and P. Ghosh, J. Am. Chem. Soc., 2007, 129, 15042–15053 CrossRef CAS PubMed.
- H. Becker, Organicum (Practical Handbook of Organic Chemistry), Addisonweseley, MA, USA, 1973, p. 611 Search PubMed.
- R. Mohan and D. Panda, Cancer Res., 2008, 68, 6181–6189 CrossRef CAS PubMed.
Footnote |
† Electronic supplementary information (ESI) available: Copies of spectra of final compounds and figures related to biological activity. See DOI: 10.1039/c3ra47720g |
|
This journal is © The Royal Society of Chemistry 2014 |
Click here to see how this site uses Cookies. View our privacy policy here.