DOI:
10.1039/C4RA05687F
(Paper)
RSC Adv., 2014,
4, 35653-35658
Phenyl-capped cyclopenta[c]chalcogenophenes: synthesis, crystal structures, electrochemistry and theoretical insights†
Received
13th June 2014
, Accepted 30th July 2014
First published on 30th July 2014
Abstract
Two new phenyl capped cyclopenta[c]chalcogenophenes (PhCPTPh and PhCPSPh) were synthesized by zirconocene mediated coupling and characterized by single crystal X-ray diffraction (SCXRD). Replacement of sulfur by selenium destabilized the HOMO level and increased the structural rigidity. The crystal structures of both the compounds were found to be nearly planar. PhCPSPh, though isostructural with PhCPTPh, packed in a very-ordered and specific pattern by Se⋯π interactions between two neighboring molecules. Hirshfeld surface analysis supported the crystal structure study. Theoretical calculations exhibited very close reorganization energies of 0.23 and 0.22 eV for PhCPTPh and PhCPSPh, respectively.
Introduction
In recent years, π-conjugated semiconducting organic compounds have been widely investigated as materials for organic electronic devices1 such as organic and polymeric light emitting diodes,2 field effect transistors,3 and photovoltaic cells.4 The monodisperse oligomers are excellent candidates with many advantages including their well-defined chemical structures, better solubility, easier synthesis and purification, fewer defects and the ability of introducing versatile functionalities if compared to the corresponding polydisperse polymers.5 However, these present both benefits and challenges to design and synthesize π-conjugated small molecular systems for high-performance electronic materials.
Cyclopenta[c]chalcogenophene based solution-processable small molecules were electrochemically polymerized by our group to obtain polymers of band gap in the range of 1.69–1.98 eV.6 Single crystal X-ray diffraction (SCXRD) and density functional theory (DFT) studies revealed that the trimer and polymers are almost planar leading to highly conjugated systems. Cyclopenta[c]chalcogenophene based solution-processable polymers have been introduced and studied for the application in organic electronics.7–9 The comparative study between two isostructural cyclopenta[c]chalcogenophene based polymers differing only by the heteroatom (S or Se) has shown different optoelectronic properties.10–12 Thus, a change of heteroatoms13 in similar systems can alter the electronic properties, chemical stability6 and ease of polymerization.12 3,4-Cyclopentane substitution10 does not disturb the planarity of the resulting conjugated oligomer, which is similar to 3,4-ethylenedioxy substitution and in contrast to 3,4-dialkyl substitution in the form of two separate alkyl chains or six-membered or higher rings.
Cyclopenta[c]selenophene (CPS) containing conjugated oligomers represent an intriguing and promising class of materials.6–11 Synthesis of cyclopenta[c]chalcogenophene based compounds have remained interesting.14–19 Here we report synthesis of phenyl capped cyclopenta[c]chalcogenophenes and a comparative study from the crystal structures, Hirshfeld surface analysis, electrochemistry and DFT study.
Results and discussion
To investigate the structure–property relationship between phenyl capped cyclopenta[c]thiophene (CPT) (PhCPTPh) and cyclopenta[c]selenophene CPS (PhCPSPh), these compounds were synthesized as shown in Scheme 1. Compound 2 was synthesized by Sonogashira coupling of the 1,6-diyne (1)20 and bromobenzene. The PhCPTPh and PhCPSPh were prepared by zirconocene mediated coupling6 of the phenyl-capped 1,6-diyne 1 by addition of n-butyllithium (n-BuLi) and zirconocene dichloride (ZrCp2Cl2) followed by addition of S2Cl2 and in situ prepared SeCl2, respectively. An acidic work up afforded PhCPSPh and PhCPTPh. PhCPSPh possesses higher melting point than that of PhCPTPh.
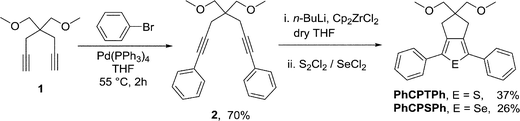 |
| Scheme 1 Synthesis of PhCPTPh and PhCPSPh. | |
Thermal analysis
Thermogravimetric analyses (TGA) were performed to check the thermal stability of these compounds (Fig. 1). Upon heating, PhCPTPh showed Td (temperature of 5% mass loss) at 203 °C, whereas PhCPSPh showed Td at 237 °C. This could be attributed to the presence of selenium atom, which provides more structural rigidity to PhCPSPh. To observe the presence of any melting or crystallization temperature, differential scanning calorimetry (DSC) was performed (Fig. S7†). PhCPTPh exhibited a typical DSC thermogram with two melting temperatures separated by a rapid crystallization. The cooling curve showed a reversible crystallization at 91 °C. In contrast, PhCPSPh showed a permanent melting at 156 °C which did not recrystallize during cooling. This could be attributed to more structural rigidity of the conjugated backbone of PhCPSPh.
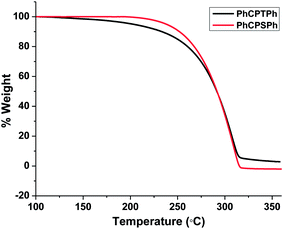 |
| Fig. 1 TGA of PhCPTPh and PhCPSPh. | |
Electrochemical properties
To estimate the highest occupied molecular orbital (HOMO) levels, cyclic voltammetry (CV) experiments were performed in a three-electrode cell comprising a Pt-disk working electrode, Pt-wire counter electrode and a Ag/AgCl reference electrode (Fig. 2). PhCPTPh showed oxidation peak at 1.32 V with an onset of oxidation potential (Eoxonset) of 1.14 V that corresponds to a HOMO level of −5.54 eV.21 Presence of more polarizable selenium atom in PhCPSPh eased the oxidation process and the oxidation peak for PhCPSPh appeared at 1.18 V with Eoxonset of 1.01 V. The HOMO level of PhCPSPh was calculated to be −5.41 eV. This can be attributed to the lower electronegativity of the selenium than sulfur which helped PhCPSPh to build up more electron density and increases the quinoidal character compared to PhCPTPh.22 So, the alteration in only heteroatom of the π-conjugated core was able to uplift the HOMO level (by 0.13 eV).23
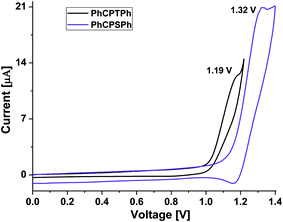 |
| Fig. 2 CV of PhCPTPh and PhCPSPh. | |
Single crystal X-ray diffraction (SCXRD) studies
Crystals of PhCPTPh were obtained by slow evaporation method from its dilute solution in hexane for SCXRD study. Due to failure to resolve the crystal data at room temperature, its SCXRD data was collected at 153 K (153 (2)), whereas the structure of PhCPSPh was resolved from the data obtained at 273 K (273 (2)). This could be attributed to the higher extent of free rotation of the benzene rings at room temperature in PhCPTPh compared to that of PhCPSPh. The increasing extent of double bond character of the C–C bonds between the benzene rings and the central CPS unit may afford more rigid structure for PhCPSPh than that of PhCPTPh. The poarizability of selenium atom is higher than sulfur, which increases the electron density in the conjugated core thus making it more quinoidal and less aromatic.22
PhCPTPh showed nearly planar geometry with the dihedral angles of 162.5° (C1–C6–C7–C8) and 156.7° (C16–C11–C10–C9) between benzene rings and central CPT unit (Fig. 3(a)). These angles are quite similar to those of thiophene capped CPT reported earlier.6 Six different kinds of non-bonding interactions are responsible for the molecule to pack in zigzag manner (Table S1†). The heteroatom of a molecule exhibits only one type of S⋯π (S1⋯C14 = 3.488) interaction with the neighboring molecule (Fig. 3(b)). The unit cell has a typical arrangement of 8 molecules in two separate zones.
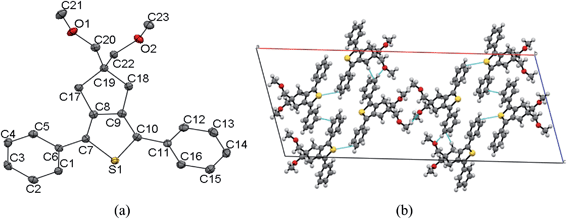 |
| Fig. 3 (a) ORTEP diagram of the crystals of PhCPTPh, drawn at 50% probability level. The hydrogen atoms are omitted for clarity. (b) Several non-bonding interactions observed via ac plane in a unit cell of PhCPTPh. | |
Crystals of PhCPSPh were obtained by its recrystallization from a saturated hexane solution. The molecule is nearly planar with two phenyl rings on the either side of the central CPS unit maintaining slightly different dihedral angles of 158.5° and 159.7°. Though PhCPSPh is isostructural with PhCPTPh, it exhibits a different packing pattern. The crystal packed in zigzag fashion initiated by non-bonding interactions among the molecules in the crystal (Fig. 4, Table S1†). The concomitant Se⋯π interactions (C23⋯Se1 = 3.51 Å) between to neighboring molecules enforce closely placed conjugated cores thus ensuring the proximity of the Se atoms and conjugated cores. One of the H of the terminal OCH3 group, which is protruded towards the conjugated part of another neighboring molecule interacts with CPS ring (C–H⋯π interaction, C12–H12B⋯C7 = 2.89 Å).
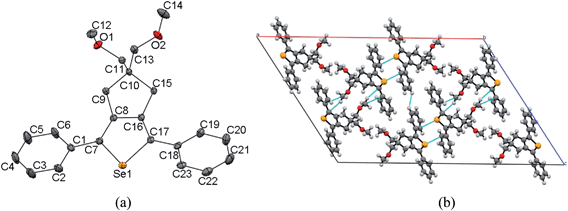 |
| Fig. 4 (a) ORTEP diagram of the crystals of PhCPSPh, drawn at 50% probability level. The hydrogen atoms are omitted for clarity. (b) Several non-bonding interactions observed via ac plane in a unit cell of PhCPSPh. | |
Hirshfeld surface analysis
The Hirshfeld surface approach as implemented in the program Crystal Explorer 3.1 (ref. 24) was employed. Directions and strengths of intermolecular interactions within the crystal were mapped onto the Hirshfeld surfaces using the descriptor ‘dnorm’, which is a ratio encompassing the distances of any surface point to the nearby interior (di) and exterior (de) atoms and the van der Waals radii of the atoms. Hirshfeld surface representation using dnorm is shown in Fig. 5. The red spots over the surface indicate the intermolecular-contacts involving strong interactions (for e.g.- O⋯H in case of PhCPTPh and Se⋯π in case of PhCPSPh). Fig. 5 shows that the concomitant Se⋯π interaction is more prominent as compared to S⋯π interaction which ensures close packing of conjugated cores in case of PhCPSPh.
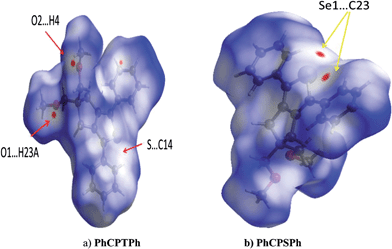 |
| Fig. 5 Hirshfeld surface representations with the property dnorm mapped, which ranges from (a) −0.049 (red) to 1.375 Å (blue) for PhCPTPh and (b) −0.048 (red) to 1.269 Å (blue) for PhCPSPh. | |
The associated fingerprint plots (plots of di vs. de) are useful in identifying and comparing different kinds of intermolecular interactions. Fig. 6 shows the fingerprint plots for PhCPTPh and PhCPSPh as obtained from a breakdown of the Hirshfeld surfaces mapped with the property dnorm. Fingerprint plot in both cases is identical with regard to its shape and relative contribution of different interactions (Table S3†). However relative contribution of Se⋯π interaction is more prominent than that of S⋯π interaction (Fig. S8†).
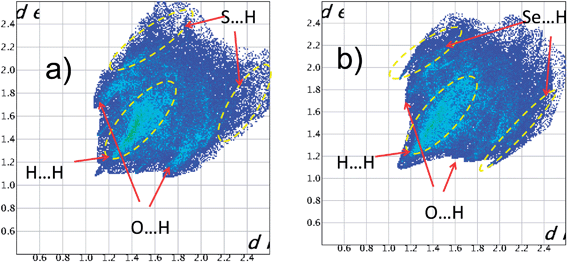 |
| Fig. 6 Fingerprint plot of (a) PhCPTPh and (b) PhCPSPh. | |
Theoretical calculation
To achieve insights about the geometries and reorganization energies of these compounds, DFT calculation at B3LYP/6-31G(d) were performed using Gaussian 09 program package.25 The optimized structures exhibited deviation of the capped phenyl rings from central CPS and CPT units by ∼27 and 31° for PhCPTPh and PhCPSPh, respectively. These dihedral angles are nearly 10° larger than that of the experimentally obtained torsional angles from SCXRD study (Table 1).
Table 1 Optimized structures of PhCPTPh and PhCPSPh
Compounds |
Top-view |
Side-view |
PhCPTPh |
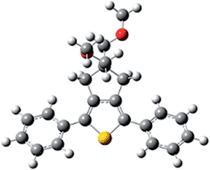 |
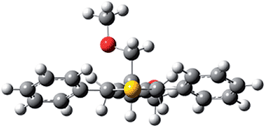 |
PhCPSPh |
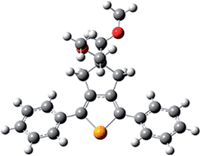 |
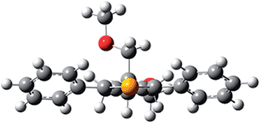 |
Further, the reorganization energies were calculated for both the compounds (Table S4†) using similar methodology as reported in the ref. 26. According to the Marcus theory (eqn (1)),27 the self-exchange rate (kct) and the charge carrier mobility are calculated by the electronic coupling between the adjacent molecules (t) and the reorganization energy (λ). Eqn (1) explains that, t is required to be maximized and λ needs to be small to obtain efficient charge carrier mobility.28 kct can be expressed as,
|
kct = 4πt2/h(1/4πλkbT)1/2 exp(−λ/4kbT)
| (1) |
Here, kb is the Boltzmann constant, h is the Planck constant, and T is the temperature. Fundamentally, the change in the geometry of the two interacting molecules during the electron transfer process is represented by λ, which is constituted of two factors,28 (a) internal and (b) external. The intramolecular reorganization energy belongs to the external factors. So, that has been neglected due to its comparatively smaller contribution in solid state.29 Both the title compounds, studied at the same level of theory (B3LYP/6-31G(d)), exhibited λ of only 0.22 and 0.23 eV for PhCPTPh and PhCPSPh, respectively. It indicates that the similar course of structural changes may be occurring in the molecules while shuttling between the neutral and charged states.
Conclusion
We have successfully synthesized phenyl capped cyclopenta[c]chalcogenophene and characterized by SCXRD technique. DFT calculation predicted almost similar structure and the reorganization energy for both the compounds. The selenium insertion in the conjugated system in place of sulfur destabilized the HOMO level by facilitating easy oxidation and also resulted in a more rigid conjugated core. The crystal structures of both the isostructural compounds were found to be closed to planarity, however, packing pattern of PhCPSPh and PhCPTPh are different due to different strength of Se⋯π and S⋯π interactions.
Experimental
Synthesis
Synthesis of compound 2. Compound 1 (1 g, 5.95 mmol), bromobenzene (1.8 g, 11.5 mmol), Pd(PPh3)4 (115 mg, 0.1 mmol) and CuI (19 mg, 0.1 mmol), were stirred in 15 mL of dry THF under nitrogen. To this solution was added 12 mL of diisopropylamine. The resulting solution was warmed at 55 °C for overnight. The crude reaction mixture was filtered through Celite-545 pad. Filtrate was diluted with 30 mL diethylether and washed with 10% NH4OH, water, 2 N HCl, water and brine. The organic layer was separated and dried over sodium sulphate. After the volatile material was removed by rotary evaporator, the residue was subjected to column chromatography (100–200 mesh silica gel, 1% ethyl acetate/hexane). A colorless pure liquid 2 was isolated, (1.4 g, yield = 77%). 1H NMR (400 MHz, CDCl3): δ 7.45–7.43 (m, 4H), 7.33–7.29 (m, 6H), 3.51 (s, 4H), 3.42 (s, 6H), 2.65 (s, 4H): 13C NMR (100 MHz, CDCl3): δ 131.5, 128.16, 127.6, 123.8, 86.5, 82.6, 74.0, 59.4, 42.6, 23.0. HRMS (ESI) calculated for C23H24O2 [M]+, 332.1776; found 332.1768.
Synthesis of compound PhCPTPh. To Cp2ZrCl2 (1.04 g, 3.56 mmol) in 35 mL of dry THF was added n-BuLi (4.7 mL, 7.5 mmol, 1.6 M in hexanes) at −78 °C. After the solution was stirred for about 20 min, 2-(4,4-bis(methoxymethyl)-7-(benzene-2-yl)hepta-1,6-diynyl)benzene 2 (987 mg, 2.97 mmol) in 4 mL of dry THF was added dropwise. The resulting solution was allowed to warm to room temperature, and stirred for additional 2 h. The color of the solution changed from pale yellow to dark brown-red. S2Cl2 (0.26 mL) was added slowly via syringe at 0 °C, and the solution was stirred overnight. The reaction was quenched by pouring the resulting solution into ice-cold 3 N HCl, and the resulting mixture was filtered through celite pad and then extracted with diethylether. The organic layer was washed with water and brine, and then dried over Na2SO4. The volatile material was then removed and mixture was column chromatographed (230–400 mesh silica gel, 1% ethylacetate in hexane) to get pure compound PhCPTPh (400 mg, yield = 37%) as white solid. Mp: 131–136 °C. 1H NMR (400 MHz, CDCl3): δ 7.55 (d, J = 7.6 Hz, 4H), 7.37 (t, J = 7.6 Hz, 4H), 7.24 (t, J = 7.6 Hz, 2H), 3.42 (s, 4H), 3.36 (s, 6H), 2.85 (s, 4H). 13C NMR (100 MHz, CDCl3): δ 143.7, 134.5, 132.0, 128.7, 126.6, 126.1, 76.0, 59.2, 54.7, 34.7. HRMS (ESI) calculated for C23H24O2S [M]+, 364.1497; found 364.1469.
Synthesis of compound PhCPSPh. To Cp2ZrCl2 (703 mg, 2.4 mmol) in 35 mL of dry THF was added n-BuLi (3 mL, 4.8 mmol, 1.6 M in hexanes) at −78 °C. After the solution was stirred for about 20 min, 2-(4,4-bis(methoxymethyl)-7-(benzene-2-yl)hepta-1,6-diynyl)benzene 2 (758 mg, 2.28 mmol) in 4 mL of dry THF was added dropwise. The resulting solution was allowed to warm to room temperature, and stirred for additional 2 h. The color of the solution changed from pale yellow to dark brown-red. SeCl2 (Se = 183 mg, 2.31 mmol, SO2Cl2 = 189 mL, 2.31 mmol)30 in 2 mL dry THF was added slowly via syringe at 0 °C, and the solution was stirred overnight. The reaction was quenched by pouring the resulting solution into ice-cold 3 N HCl, and the resulting mixture was filtered through celite pad and then extracted with diethylether. The organic layer was washed with water and brine, and then dried over Na2SO4. The volatile material was then removed and the resulting dark red liquid was subjected to column chromatography (230–400 mesh, silica gel, 1% ethyl acetate/hexanes) to get white solid PhCPSPh (247 mg, yield = 26%). Mp: 150–155 °C. 1H NMR (400 MHz, CDCl3): δ 7.51 (d, J = 5.6 Hz, 4H), 7.38 (t, J = 6 Hz, 4H), 7.25 (t, J = 6 Hz, 2H), 3.39 (s, 4H), 3.35 (s, 6H), 2.78 (s, 4H). 13C NMR (100 MHz, CDCl3): δ 131.5, 128.1, 127.6, 123.8, 86.5, 82.6, 74.0, 59.4, 42.6, 23.0. HRMS (ESI) calculated for C23H24O2Se [M]+, 412.0942; found 412.0945.
Acknowledgements
This work is supported by CSIR, India.
References
-
(a) G. C. Bazan, J. Org. Chem., 2007, 72, 8615–8635 CrossRef CAS PubMed;
(b) C.-L. Wang, H.-L. Dong, W.-P. Hu, Y.-Q. Liu and D.-B. Zhu, Chem. Rev., 2012, 112, 2208–2267 CrossRef CAS PubMed.
-
(a) M. C. Gather, A. Kohnen and K. Meerholz, Adv. Mater., 2011, 23, 233–248 CrossRef CAS PubMed;
(b) T. Hu, L. He, L. Duan and Y. Qiu, J. Mater. Chem., 2012, 22, 4206–4215 RSC.
-
(a) M. C. R. Delgado, E. G. Kim, D. A. da Silva and J. L. Bredas, J. Am. Chem. Soc., 2010, 132, 3375–3387 CrossRef PubMed;
(b) F. Wurthner and M. Stolte, Chem. Commun., 2011, 47, 5109–5115 RSC.
- Y.-M. Sun, G. C. Welch, W. L. Leong, C. J. Takacs, G. C. Bazan and A. J. Heeger, Nat. Mater., 2012, 11, 44–48 CrossRef CAS PubMed.
- G. M. Farinola and R. Ragni, Chem. Soc. Rev., 2011, 40, 3467–3482 RSC.
- S. Das, A. Bedi, G. Ramakrishna, C. M. Reddy and S. S. Zade, Org. Biomol. Chem., 2011, 9, 6963–6972 CAS.
- S. Das, S. P. Senanayak, A. Bedi, K. S. Narayan and S. S. Zade, Polymer, 2011, 52, 5780–5787 CrossRef CAS.
- A. Bedi, S. P. Senanayak, S. Das, K. S. Narayan and S. S. Zade, Polym. Chem., 2012, 3, 1453–1460 RSC.
- A. Bedi, S. P. Senanayak, K. S. Narayan and S. S. Zade, J. Polym. Sci., Part A: Polym. Chem., 2013, 51, 4481–4488 CrossRef CAS.
- S. Das and S. S. Zade, Chem. Commun., 2010, 46, 1168–1170 RSC.
- A. Bedi, S. P. Senanayak, K. S. Narayan and S. S. Zade, Macromolecules, 2013, 46, 5943–5950 CrossRef CAS.
- A. Bedi and S. S. Zade, Macromolecules, 2013, 46, 8864–8872 CrossRef CAS.
- A. Saito, Y. Matano and H. Imahori, Org. Lett., 2010, 12, 2675–2677 CrossRef CAS PubMed.
- P. J. Fagan and W. A. Nugent, J. Am. Chem. Soc., 1988, 110, 2310–2312 CrossRef CAS.
- P. J. Fagan, W. A. Nugent and J. C. Calabrese, J. Am. Chem. Soc., 1994, 116, 1880–1889 CrossRef CAS.
- D. Payra, Z. Song, K.-i. Kanno and T. Takahashi, Chem. Lett., 2011, 40, 1447–1449 CrossRef CAS.
- G. He, L. Kang, W. T. Delgado, O. Shynkaruk, M. J. Ferguson, R. McDonald and E. Rivard, J. Am. Chem. Soc., 2013, 135, 5360–5363 CrossRef CAS PubMed.
- X. Yan, K. Fang, H. Liua and C. Xi, Chem. Commun., 2013, 49, 10650–10652 RSC.
- G. He, W. T. Delgado, D. J. Schatz, C. Merten, A. Mohammadpour, L. Mayr, M. J. Ferguson, R. McDonald, A. Brown, K. Shankar and E. Rivard, Angew. Chem., Int. Ed., 2014, 53, 4587–4591 CrossRef CAS PubMed.
- N. Cadran, K. Cariou, G. Hervé, C. Aubert, L. Fensterbank, M. Malacria and J. Marco-Contelles, J. Am. Chem. Soc., 2004, 126, 3408–3409 CrossRef CAS PubMed.
- The HOMO level was obtained using the equation: EHOMO = −4.40 − (Eoxonset) Eferrocene1/2 was estimated to be 0.4 V vs. Ag/AgCl electrode.
-
(a) S. S. Zade, N. Zamoshchik and M. Bendikov, Chem.−Eur. J., 2009, 15, 8613–8624 CrossRef CAS PubMed ; and references therein;
(b) Z. Chen, C. S. Wannere, C. Corminboeuf, R. Puchta and P. v. R. Schleyer, Chem. Rev., 2005, 105, 3842–3888 CrossRef CAS PubMed.
- U. Purushotham and G. N. Sastry, Phys. Chem. Chem. Phys., 2013, 15, 5039 RSC.
-
(a) M. A. Spackman and D. Jayatilaka, CrystEngComm, 2009, 11, 19 RSC;
(b) S. K. Wolff, D. J. Grimwood, J. J. McKinnon, M. J. Turner, D. Jayatilaka and M. A. Spackman, CrystEngComm, 2014, 16, 4508–4538 RSC;
(c) Crystal Explorer (Version 3.0), University of Western Australia, 2010 Search PubMed;
(d) M. J. Turner, J. J. McKinnon, D. Jayatilaka and M. A. Spackman, CrystEngComm, 2011, 13, 180 RSC.
- M. J. Frisch, G. W. Trucks, H. B. Schlegel, G. E. Scuseria, M. A. Robb, J. R. Cheeseman, G. Scalmani, V. Barone, B. Mennucci, G. A. Petersson, H. Nakatsuji, M. Caricato, X. Li, H. P. Hratchian, A. F. Izmaylov, J. Bloino, G. Zheng, J. L. Sonnenberg, M. Hada, M. Ehara, K. Toyota, R. Fukuda, J. Hasegawa, M. Ishida, T. Nakajima, Y. Honda, O. Kitao, H. Nakai, T. Vreven, J. A. Montgomery Jr, J. E. Peralta, F. Ogliaro, M. Bearpark, J. J. Heyd, E. Brothers, K. N. Kudin, V. N. Staroverov, R. Kobayashi, J. Normand, K. Raghavachari, A. Rendell, J. C. Burant, S. S. Iyengar, J. Tomasi, M. Cossi, N. Rega, J. M. Millam, M. Klene, J. E. Knox, J. B. Cross, V. Bakken, C. Adamo, J. Jaramillo, R. Gomperts, R. E. Stratmann, O. Yazyev, A. J. Austin, R. Cammi, C. Pomelli, J. W. Ochterski, R. L. Martin, K. Morokuma, V. G. Zakrzewski, G. A. Voth, P. Salvador, J. J. Dannenberg, S. Dapprich, A. D. Daniels, O. Farkas, J. B. Foresman, J. V. Ortiz, J. Cioslowski and D. J. Fox, Gaussian 09, Revision E.01, Gaussian Inc., Wallingford CT, 2009 Search PubMed.
- S. S. Zade and M. Bendikov, Chem.–Eur. J., 2008, 14, 6734–6741 CrossRef CAS PubMed.
- R. A. Marcus, J. Chem. Phys., 1956, 24, 966–978 CrossRef CAS.
- M. Mas−Torrent, P. Hadley, S. T. Bromley, X. Ribas, J. Tarrés, M. Mas, E. Molins, J. Veciana and C. Rovira, J. Am. Chem. Soc., 2004, 126, 8546–8553 CrossRef PubMed.
- V. Coropceanu, J. Cornil, D. A. Da Silva Filho, Y. Olivier, R. Silbey and J. L. Bredas, Chem. Rev., 2007, 107, 926–952 CrossRef CAS PubMed.
- A. Maaninen, T. Chivers, M. Parvez, J. Pietikäinen and R. S. Laitinen, Inorg. Chem., 1999, 38, 4093–4097 CrossRef CAS.
Footnote |
† Electronic supplementary information (ESI) available: DSC of PhCPTPh and PhCPSPh; crystallographic data and refinement parameters for crystal structures; more details about Hirshfeld surface analysis and coordinates of optimized structures; copies of the 1H and 13C NMR spectra for all new compounds. CCDC 995427 and 998094. For ESI and crystallographic data in CIF or other electronic format see DOI: 10.1039/c4ra05687f |
|
This journal is © The Royal Society of Chemistry 2014 |