DOI:
10.1039/C4RA10199E
(Paper)
RSC Adv., 2014,
4, 48085-48094
Synthesis of oxazolidinofullerenes/thiazolidinofullerenes: novel reaction of [60]fullerene with isocyanates/isothiocyanates promoted by ferric perchlorate†
Received
3rd August 2014
, Accepted 23rd September 2014
First published on 23rd September 2014
Abstract
The facile one-step reaction of [60]fullerene with isocyanates/isothiocyanates in the presence of ferric perchlorate generates a series of oxazolidinofullerenes/thiazolidinofullerenes, which are difficult to prepare by common methods. The direct dissolution approach plays an important role in the efficient synthesis of oxazolidinofullerenes/thiazolidinofullerenes. A possible reaction mechanism for the product formation is proposed.
Introduction
Since the availability of fullerenes in a macroscopic amount, many chemical reactions for the functionalization of fullerenes have been explored and numerous fascinating fullerene derivatives with widely structural diversities have been prepared.1 Radical reactions2 are one of the first investigated fullerene reactions and are still important methodologies to functionalize fullerenes.3 Recently, radical reactions of fullerenes promoted by transition metal salts2,4 such as Mn(OAc)3,5 Fe(ClO4)3,6 FeCl3,7 TBADT[(n–Bu4N)4W10O32],8 Cu(OAc)2,5d,9 Pb(OAc)4,5g,10 CoCl2dppe,11 and Ag2CO3 (ref. 12) have attracted extensive attention and have exhibited remarkable advantages over the traditional peroxide- or light-initiated processes. Through which, a large number of novel mono-addition fullerene derivatives have been synthesized. For instance, the reactions of C60 with nitriles,6a aldehydes/ketones,6b malonate esters,6c arylboronic acids,6d acid chlorides,6e and β-keto esters6f in the presence of Fe(ClO4)3 afforded the C60-fused oxazoles, C60-fused 1,3-dioxolanes, C60-fused disubstituted lactones, fullerenyl boronic esters, 1,2-fullerenols, and C60-fused hemiketal and dihydrofuran, respectively.
Although a large variety of fullerene derivatives have been prepared over the past two decades, oxazolidinofullerenes/thiazolidinofullerenes are still relatively scarce until now. Banks13a and Luh13b almost at the same time reported the synthesis of the first oxazolidinofullerene by chemical transformation of an aziridinofullerene product from cycloaddition of [60]fullerene (C60) with N3CO2Et. Minakata and co-workers described the preparation of another four oxazolidinofullerenes by a ring-expansion reaction of aziridinofullerenes with CO2 catalyzed by PCy3.13c Wang's group recently realized the synthesis of seven oxazolidinofullerenes by the FeCl3-catalyzed reaction of C60 with tert-butyl N-substituted carbamates.7f Among the reported oxazolidinofullerene derivatives, only those from Wang's group were prepared directly from C60. However, the one-step methodology of Wang's group mainly afforded alkyl-substituted oxazolidinofullerenes. As for thiazolidinofullerenes, only Gan's group described the synthesis of a few thiazolidinofullerenes through the reaction of aryl isothiocyanates with fullerene epoxides containing four tert-butylperoxo groups in the presence of a Lewis acid.14 It should be noted that the reported methodology for the preparation of thiazolidinofullerenes by Gan et al. required a multi-step procedure starting from C60 and gave only thiazolidinofullerenes with multiple addends.14 On the other hand, oxazolidinofullerenes/thiazolidinofullerenes may have promising applications in life science because oxazolidinones/thiazolidinones have exhibited a wide range of biological activities.15 Oxazolidinofullerenes/thiazolidinofullerenes are also valuable precursors for further functionalizations.13a,14 Therefore, it is still demanding to develop new protocol directly from C60 to obtain oxazolidinofullerenes/thiazolidinofullerenes, especially the rare aryl-substituted oxazolidinofullerenes as well as the unreported mono-addition thiazolidinofullerenes, in a straightforward and efficient way with a broad substrate scope.
In efforts to extend the Fe(ClO4)3-mediated radical reactions of C60, herein we describe the one-step reaction of C60 with isocyanates/isothiocyanates promoted by Fe(ClO4)3 to afford a series of scarce aryl-substituted oxazolidinofullerenes and mono-addition thiazolidinofullerenes.
Results and discussion
In our previous study, we found that the direct dissolution6a–c,e,f of Fe(ClO4)3 played a crucial role for the efficient synthesis of fullerene derivatives. Similarly, we explored the Fe(ClO4)3-promoted reaction of C60 with various isocyanates by adopting the direct dissolution method, i.e., Fe(ClO4)3 was first dissolved in a chosen isocyanate, and then the dichlorobenzene (ODCB) solution of C60 was added. Much to our satisfaction, we found that the Fe(ClO4)3-mediated reaction of C60 with isocyanates 1a–g, that is, phenyl isocyanate (1a), 4-tolyl isocyanate (1b), 3-tolyl isocyanate (1c), 4-methoxyphenyl isocyanate (1d), 3,5-dimethylphenyl isocyanate (1e), 4-chlorophenyl isocyanate (1f), and 3,4-dichlorophenyl isocyanate (1g), afforded oxazolidinofullerenes 2a–g.
Initially, the reaction of C60 with phenyl isocyanate (1a) in the presence of Fe(ClO4)3 was chosen to screen the reaction conditions. The details are listed in Table 1. As can be seen from Table 1, the reaction of C60, Fe(ClO4)3, and 1a in a molar ratio of 1
:
2
:
20 gave oxazolidinofullerene 2a in 26% of isolated yield at 100 °C (entry 1, Table 1). Decreasing the reaction temperature to 80 °C drastically reduced the isolated yield of oxazolidinofullerene 2a even by extending the reaction time to 22 h (entry 2, Table 1). Increasing the reaction temperature to 120 °C did not improve the yield of product 2a (entry 3, Table 1). No benefit to the yield of oxazolidinofullerene 2a could be achieved by variation of the amount of phenyl isocyanate (entries 4 and 5, Table 1). Slight lower yield was obtained by increasing or decreasing the amount of Fe(ClO4)3 (entries 6 and 7 vs. entry 1, Table 1). The dissolution of Fe(ClO4)3 in acetonitrile rather than in 1a lowered the product yield and gave some unknown highly polar byproducts (entry 8, Table 1). Therefore, the reagent molar ratio of C60–Fe(ClO4)3–1a as 1
:
2
:
20 and the reaction temperature as 100 °C together with the direct dissolution method were chosen as the optimized reaction conditions. These optimized reaction conditions could be extended to other isocyanates.
Table 1 Optimization of reaction conditions for the Fe(ClO4)3-mediated reaction of C60 with phenyl isocyanate 1aa
The reaction times and yields for the Fe(ClO4)3-mediated reaction of C60 with isocyanates 1a–g under the optimized conditions are summarized in Table 2.
Table 2 Reaction times and yields for the reaction of C60 with isocyanates 1a–g in the presence of Fe(ClO4)3a
As can be seen from Table 2, all of the examined isocyanates 1a–g bearing either electron-donating groups or electron-withdrawing groups could be successfully utilized to prepare oxazolidinofullerenes 2a–g in 9–39% yields (41–91% based on consumed C60), comparable to the previously reported data for most monoadducts. In the case of 4-methoxyphenyl isocyanate (1d), increasing the amount of Fe(ClO4)3 to 3 equiv. together with prolonging the reaction time to 24 h could provide an acceptable yield (20%) of oxazolidinofullerene 2d. As for 3,4-dichlorophenyl isocyanate (1g), some unknown byproducts besides the desired oxazolidinofullerene 2g were produced for a long reaction time probably attributed to the higher reactivity of 1g owing to the existence of two electron-withdrawing chloro groups, and thus shorter reaction time such as 5 h was chosen although the oxazolidinofullerene 2g was obtained only in 9% yield.
We also investigated the reaction of C60 with 4-nitrophenyl isocyanate (1h) bearing the stronger electron-withdrawing NO2 group in the presence of Fe(ClO4)3 by the dissolution of Fe(ClO4)3 in 1h (direct dissolution method), and found that aziridinofullerene 3 was unexpectedly obtained as the major product in 7% of isolated yield under standard experimental conditions for 25 h (Scheme 1). The yield of aziridinofullerene 3 could be increased to 19% (Scheme 1) when the reaction was conducted by the dissolution of Fe(ClO4)3 in acetonitrile under air conditions for 40 h. To our disappointment, no anticipated oxazolidinofullerene was isolated from the above reaction. Very recently, Yang and co-workers have reported the anhydrous CuCl2-mediated reaction of C60 with 4-nitroaniline under air conditions to afford aziridinofullerene 3.16 We thus conjectured that the formation of aziridinofullerene 3 should proceed via the Fe(ClO4)3-mediated reaction of C60 with 4-nitroaniline generated in situ by the addition of H2O in our system to 4-nitrophenyl isocyanate (1h) accompanied with the elimination of CO2. Controlled experiments indicated that the reaction of C60, Fe(ClO4)3, and 4-nitroaniline by the dissolution of Fe(ClO4)3 in acetonitrile under air conditions at 150 °C for 22 h produced aziridinofullerene 3 in 14% yield (Scheme 1). It should be noted that controlled experiments were also conducted at 100 °C. Nevertheless, only a trace amount of aziridinofullerene 3 was observed even by prolonging the reaction time to 40 h. The difficulty in preparing aziridinofullerene 3 by the Fe(ClO4)3-mediated reaction of C60 with 4-nitroaniline was probably attributed to the existence of higher amount of H2O in the reaction system. In addition, other isocyanates such as tert-butyl isocyanate, cyclohexyl isocyanate, methylene diphenyl diisocyanate (MDI), and hexamethylene diisocyanate (HDI) have also be treated with C60 and Fe(ClO4)3 under similar reaction conditions. Unfortunately, the desired oxazolidinofullerenes could not be successfully isolated.
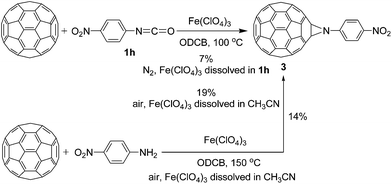 |
| Scheme 1 Fe(ClO4)3-mediated reaction of C60 with 4-nitrophenyl isocyanate 1h and 4-nitroaniline affording aziridinofullerene 3. | |
The structures of oxazolidinofullerenes 2a–g were fully characterized by HR MS, 1H NMR, 13C NMR, FT-IR, and UV-vis spectra. All of high-resolution mass spectra of these oxazolidinofullerene products gave the correct molecular ion peaks. Their 1H NMR spectra displayed the expected chemical shifts as well as the splitting patterns for all protons. In their 13C NMR spectra, the peak for the C
O carbon appeared at 152.12–154.51 ppm, and the two sp3-carbons of the C60 skeleton were located at 91.47–93.20 ppm and 78.72–80.17 ppm, close to those of the reported oxazolidinofullerenes in the previous literature.7f,13 No more than 28 peaks including some overlapped ones for the 58 sp2-carbons of the C60 moiety were observed in the range of 136–149 ppm, consistent with the Cs symmetry of their molecular structures. The IR spectra of 2a–g showed absorptions at 1768–1772 cm−1 due to the C
O group. Their UV-vis spectra exhibited a peak at 417–420 nm, which is a diagnostic absorption for the 1,2-adduct of C60, to which the oxygen atom is directly attached.5f–h,6c Aziridinofullerene 3 is a known compound and its structure was confirmed by comparison of its spectral data with those reported previously.16
To expand the scope of the reaction, the substrates were extended from isocyanates to isothiocyanates. Phenyl isothiocyanate (4a), 4-fluorophenyl isothiocyanate (4b), 4-chlorophenyl isothiocyanate (4c), 4-bromophenyl isothiocyanate (4d), 4-(trifluoromethyl)phenyl isothiocyanate (4e), 4-nitrophenyl isothiocyanate (4f), 3-tolyl isothiocyanate (4g), and 4-methoxyphenyl isothiocyanate (4h) were chosen to react with C60 in the presence of Fe(ClO4)3 by the direct dissolution method, and were found to generate thiazolidinofullerenes 5a–h.
The reaction conditions were also screened by choosing the reaction of C60 with phenyl isothiocyanate (4a) promoted by Fe(ClO4)3. The details are listed in Table 3. As can be seen from Table 3, the reaction of C60 with 50 equiv. of phenyl isothiocyanate in the presence of 5 equiv. of Fe(ClO4)3 afforded thiazolidinofullerene 5a in 18% of isolated yield at 100 °C for 18 h (entry 1, Table 3). Decreasing the reaction temperature to 80 °C or increasing the reaction temperature to 120 °C had no benefit to the isolated yield of product 5a (entries 2 and 3, Table 3). The variation of the amount of phenyl isothiocyanate and Fe(ClO4)3 did not improve the isolated yield of thiazolidinofullerene 5a (entries 4–7, Table 3). The dissolution of Fe(ClO4)3 in acetonitrile rather than in 4a obviously reduced the isolated yield of thiazolidinofullerene 5a even by extending the reaction time to 24 h (entry 8, Table 3). Accordingly, the best molar ratio of C60–Fe(ClO4)3x·H2O–4a was 1
:
5
:
50, and the reaction temperature was 100 °C. These optimized reaction conditions could also be extended to other isothiocyanates.
Table 3 Optimization of reaction conditions for the Fe(ClO4)3-mediated reaction of C60 with phenyl isothiocyanate 4aa
The reaction times and yields for the Fe(ClO4)3-mediated reaction of C60 with isothiocyanates 4a–h under the optimized conditions are summarized in Table 4.
Table 4 Reaction times and yields for the reaction of C60 with isothiocyanates 4a–h in the presence of Fe(ClO4)3a
As can be seen from Table 4, aromatic isothiocyanates bearing either electron-withdrawing or electron-donating groups (4a–h) could be successfully employed to prepare thiazolidinofullerenes in 11–66% yields (19–96% based on consumed C60). For the synthesis of thiazolidinofullerene 5f, higher reaction temperature was required for the melting of 5f and subsequent dissolution of Fe(ClO4)3, and thus resulted in the generation of some unknown byproducts even by decreasing the molar ratio of C60–Fe(ClO4)3·xH2O–4f to 1
:
2
:
20. As for 4-methoxyphenyl isothiocyanate (4h), an unknown byproduct was produced accompanied with the formation of thiazolidinofullerene 5h, and accordingly gave the lower product yield. It should be noted the reaction of aliphatic isothiocyanates such as benzyl isothiocyanate and butyl isothiocyanate under the same conditions was also investigated, and found that only less than 5% yield of thiazolidinofullerenes were obtained.
The identities of thiazolidinofullerenes 5a–h were unambiguously established by HR MS, 1H NMR, 13C NMR, FT-IR, and UV-vis spectra. Comparing the spectra of 5a–h with those of 2a–g, we found that the peak at 152.12–154.51 ppm for the C
O carbon in the 13C NMR spectra of 2a–g were shifted downfield to 165.72–168.87 ppm in those of 5a–h, and the peaks at 91.47–93.20 ppm and 78.72–80.17 ppm for the two sp3-carbons of the C60 skeleton were shifted upfield to 82.62–84.17 ppm and 65.38–67.07 ppm in those of 5a–h; the absorptions at 1768–1772 cm−1 for the carbonyl group in the IR spectra of 2a–g moved to 1678–1689 cm−1 in those of 5a–h. The change of the above data agrees well with reported data in the literature.7f,13,14
In our previous study, we have found that C60O could be formed via the reaction of C60 with metal oxidants such as Mn(OAc)3 (ref. 5d) under air conditions. To avoid the formation of C60O, the reaction of C60 with various organic compounds promoted by metal oxidants such as Fe(ClO4)3 (ref. 6) has been manipulated under nitrogen atmosphere in our subsequent study. Therefore, the reaction pathway for the formation of oxazolidinofullerenes/thiazolidinofullerenes 2/5 by the Fe(ClO4)3-mediated reaction of C60O with isocyanates/isothiocyanates may be excluded under nitrogen atmosphere although the study on the reaction of epoxides with isocyanates/isothiocyanates promoted by different species to result in the generation of oxazolidinones/thiazolidinoes has been reported by different groups.14,17 To further confirm the plausibility of the above-mentioned conclusion, controlled experiments were also conducted via the reaction of C60 with 3,5-dimethylphenyl isocyanate (1e) and 4-fluorophenyl isothiocyanate (4b) promoted by Fe(ClO4)3 under air conditions (Scheme 2). Experimental results showed that oxazolidinofullerene 2e was isolated only in 16% yield when the reaction of C60 with 3,5-dimethylphenyl isocyanate (1e) was carried out at 100 °C for 24 h, which are obviously lower than that obtained under nitrogen conditions at 100 °C for 24 h (23%, Table 2). As for 4-fluorophenyl isothiocyanate (4b), the reaction under air conditions produced 12% yield of thiazolidinofullerene 5b at 100 °C for 4 h, which are also obviously lower than that obtained under nitrogen conditions at 100 °C for 4 h (25%, Table 4).
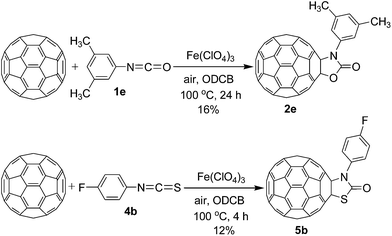 |
| Scheme 2 Fe(ClO4)3-mediated reaction of C60 with 3,5-dimethylphenyl isocyanate 1e and 4-fluorophenyl isothiocyanate 4b under air conditions. | |
On the basis of the previously suggested mechanisms for the reactions of C60 with nitriles,6a aldehydes/ketones,6b arylboronic acids,6d and acid chlorides6e in the presence of Fe(ClO4)3 together with the reported results from the reactions18 of isocyanates with active hydrogen compounds such as H2O to selectively afford carbonyl derivatives, we propose a possible formation mechanism for oxazolidinofullerenes/thiazolidinofullerenes 2/5 from the reaction of C60 with isocyanates/isothiocyanates 1/4 promoted by Fe(ClO4)3 (Scheme 3, path a). A chosen isocyanate or isothiocyanate reacts with the hydrated water in Fe(ClO4)3·xH2O or traces of water in the system to produce Fe(III)-complex 6 accompanied with the elimination of HClO4, and then isomerization to form the more stable Fe(III)-complex 7. The observed reddening of the mixtures of isocyanates/isothiocyanates and Fe(ClO4)3·xH2O hints the formation of complexes between Fe(ClO4)3·xH2O and isocyanates/isothiocyanates.19 Homolytical addition of 7 to C60 produces fullerenyl radical 8 with an elimination of Fe(ClO4)2, followed by coordination with another molecule of Fe(ClO4)3·xH2O to generate Fe(III)-complex 9, which undergoes intramolecular cyclization with the loss of a Fe(II) species to afford oxazolidinofullerenes/thiazolidinofullerenes 2/5. It should be noted that the formation of Fe(III)-complex 10 by the reaction of H2O with carbon–nitrogen double bond of isocyanate in the presence of Fe(ClO4)3 is also possible (Scheme 3, path b), and then addition of 10 to C60 produces fullerenyl radical 11, which undergoes coordination with another molecule of Fe(ClO4)3·xH2O to afford Fe(III)-complex 12, followed by intramolecular cyclization to give oxazolidinofullerenes 2. As for isothiocyanates, the reaction pathway by addition of H2O to carbon–nitrogen double bond of isothiocyanate could not afford the corresponding thiazolidinofullerenes 5, and was thus excluded. The exact reason for the exclusive addition of H2O to carbon–sulphur double bond of isothiocyanate to afford thiazolidinofullerenes 5 is not quite clear now. Additionally, an oxidative reaction pathway for the formation of oxazolidinofullerenes/thiazolidinofullerenes should also be mentioned, that is, fullerenyl radicals 8 and 11 with the aid of Fe(ClO4)3·xH2O could be oxidized to the corresponding fullerenyl cations, followed by cyclization with the loss of H+ to give the 2/5.
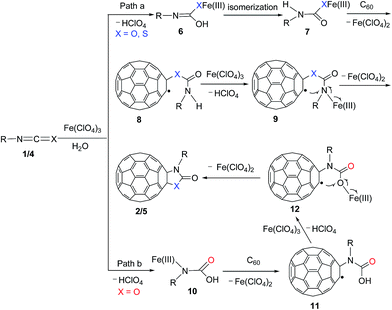 |
| Scheme 3 Proposed possible formation mechanism for oxazolidinofullerenes/thiazolidinofullerenes 2/5. | |
Conclusion
We have synthesized a series of oxazolidinofullerenes/thiazolidinofullerenes via the Fe(ClO4)3-mediated reaction of C60 with isocyanates/isothiocyanates. The current one-step approach to the preparation of oxazolidinofullerenes/thiazolidinofullerenes was obviously more straightforward and practical than the previously reported protocols.13,14 The direct dissolution of isocyanates/isothiocyanates and Fe(ClO4)3 proved to be crucial for the efficient synthesis of oxazolidinofullerenes/thiazolidinofullerenes. A plausible reaction mechanism for the formation of oxazolidinofullerenes/thiazolidinofullerenes is suggested.
Experimental section
General procedure for the Fe(ClO4)3-mediated reaction of C60 with isocyanates 1a–g
A mixture of isocyanate 1a (1b–g, 1.0 mmol) and Fe(ClO4)3·xH2O (0.10 mmol, 0.15 mmol in the case of 1d) was added to a 50 mL three-neck flask equipped with a reflux condenser, nitrogen inlet and outlet, and a magnetic stirrer. The mixture was heated in an oil bath preset at 100 °C for 10 min to allow ferric perchlorate to dissolve in the liquid isocyanate. Then to the flask was added the o-dichlorobenzene (6 mL) solution of C60 (36.0 mg, 0.05 mmol). The resulting solution was heated with vigorous stirring in the oil bath at the same temperature under nitrogen atmosphere. The reaction was carefully monitored by thin-layer chromatography (TLC) and stopped at the designated time. The resulting solution was directly separated on a silica gel column with carbon disulfide/toluene as the eluent. Oxazolidinofullerene 2a (2b–g) was obtained along with unreacted C60.
Fe(ClO4)3-mediated reaction of C60 with phenyl isocyanate 1a by the dissolution of Fe(ClO4)3 in 1a under different conditions
Fe(ClO4)3·xH2O (0.05–0.15 mmol) and 1a (0.5–1.5 mmol) was added to a 50 mL three-neck flask, which was equipped with a reflux condenser, nitrogen inlet and outlet, and a magnetic stirrer. The mixture was heated in an oil bath preset at desired temperature (80–120 °C) for 10 min to allow Fe(ClO4)3·xH2O to dissolve in liquid 1a. Then to the flask was added o-dichlorobenzene (6 mL) solution of C60 (36.0 mg, 0.05 mmol). The reaction mixture was vigorously stirred in the oil bath preset at the same temperature under nitrogen atmosphere. The reaction was monitored by TLC and stopped at the designated time (10–22 h). The resulting solution was directly separated on a silica gel column with carbon disulfide/toluene as the eluent to give unreacted C60 and oxazolidinofullerene 2a.
Fe(ClO4)3-mediated reaction of C60 with phenyl isocyanate 1a by the dissolution of Fe(ClO4)3 in acetonitrile
A mixture of C60 (36.0 mg, 0.05 mmol) and 1a (108 μL, 1.0 mmol) was dissolved in o-dichlorobenzene (6 mL), and then the resulting solution was deoxygenated by a nitrogen stream for 10 min. After Fe(ClO4)3·xH2O (46.0 mg, 0.10 mmol, dissolved in 2 mL of acetonitrile) was added, the reaction mixture was vigorously stirred at 100 °C under nitrogen atmosphere for 13 h. The resulting solution was directly separated on a silica gel column with carbon disulfide/toluene as the eluent to give first unreacted C60 (20.0 mg, 56%) and then oxazolidinofullerene 2a (6.0 mg, 14%).
Synthesis of oxazolidinofullerene 2a
According to the general procedure, the reaction of C60 (36.0 mg, 0.05 mmol) with 1a (108 μL, 1.0 mmol) and Fe(ClO4)3·xH2O (46.0 mg, 0.10 mmol) for 10 h afforded first unreacted C60 (24.9 mg, 69%) and then 2a (11.2 mg, 26%) as amorphous black solid. mp > 300 °C; 1H NMR (600 MHz, CS2/DMSO-d6) δ 7.80 (d, J = 7.4 Hz, 2H), 7.55 (t, J = 7.5 Hz, 2H), 7.48 (t, J = 7.1 Hz, 1H); 13C NMR (125 MHz, CS2/DMSO-d6) (all 2C unless indicated) δ 152.19 (1C, C
O), 147.32 (1C), 147.11 (1C), 145.48 (4C), 145.43, 145.21 (6C), 144.30, 144.14, 144.11 (4C), 143.96, 143.63, 143.53, 143.47, 143.41, 141.79, 141.72 (4C), 141.36, 141.27, 141.03, 140.98, 140.79, 140.51, 138.92, 138.59, 136.57, 136.24, 134.61 (1C, aryl C), 128.89 (aryl C), 128.66 (aryl C), 128.14 (1C, aryl C), 91.51 (1C, sp3-C of C60), 78.72 (1C, sp3-C of C60); FT-IR ν/cm−1 (KBr) 1771, 1506, 1451, 1430, 1361, 1343, 1227, 1172, 1153, 1087, 1071, 1060, 1016, 954, 857, 832, 738, 689, 546, 525; UV-vis (CHCl3) λmax/nm 257, 318, 417; HRMS (MALDI-TOF-MS with 4-hydroxy-α-cyanocinnamic acid as the matrix) m/z calcd for C67H5NO2 [M+] 855.0320, found 855.0308.
Synthesis of oxazolidinofullerene 2b
According to the general procedure, the reaction of C60 (36.0 mg, 0.05 mmol) with 1b (135 μL, 1.0 mmol) and Fe(ClO4)3·xH2O (46.0 mg, 0.10 mmol) for 14 h afforded first unreacted C60 (18.1 mg, 50%) and then 2b (17.1 mg, 39%) as amorphous black solid. mp > 300 °C; 1H NMR (600 MHz, CS2/CDCl3) δ 7.63 (d, J = 7.8 Hz, 2H), 7.32 (d, J = 7.8 Hz, 2H), 2.42 (s, 3H); 13C NMR (125 MHz, CS2/DMSO-d6) (all 2C unless indicated) δ 152.31 (1C, C
O), 147.41 (1C), 147.19 (1C), 145.56 (4C), 145.51, 145.30 (4C), 145.27, 144.39, 144.30, 144.23, 144.19, 144.12, 143.71, 143.70, 143.58, 143.51, 141.88, 141.82, 141.80, 141.45, 141.35, 141.11, 141.09, 140.88, 140.61, 139.00, 138.67, 138.18 (1C, aryl C), 136.65, 136.27, 132.07 (1C, aryl C), 129.42 (aryl C), 128.87 (aryl C), 91.47 (1C, sp3-C of C60), 78.90 (1C, sp3-C of C60), 20.64 (1C); FT-IR ν/cm−1 (KBr) 2916, 2849, 1772, 1512, 1435, 1364, 1352, 1231, 1172, 1155, 1086, 1061, 1014, 954, 864, 809, 793, 742, 546, 526; UV-vis (CHCl3) λmax/nm 257, 319, 420; HRMS (MALDI-TOF-MS with 4-hydroxy-α-cyanocinnamic acid as the matrix) m/z calcd for C68H7NO2 [M+] 869.0477, found 869.0472.
Synthesis of oxazolidinofullerene 2c
According to the general procedure, the reaction of C60 (36.0 mg, 0.05 mmol) with 1c (135 μL, 1.0 mmol) and Fe(ClO4)3·xH2O (46.0 mg, 0.10 mmol) for 24 h afforded first unreacted C60 (24.1 mg, 67%) and then 2c (10.2 mg, 30%) as amorphous black solid. mp > 300 °C; 1H NMR (600 MHz, CS2/DMSO-d6) δ 7.55 (s, 1H), 7.54 (d, J = 7.6 Hz, 1H), 7.37 (t, J = 7.6 Hz, 1H), 7.25 (d, J = 7.4 Hz, 1H), 2.45 (s, 3H); 13C NMR (125 MHz, CS2/DMSO-d6) (all 2C unless indicated) δ 152.24 (1C, C
O), 147.32 (1C), 147.10 (1C), 145.47 (4C), 145.42, 145.21 (4C), 145.19, 144.30, 144.17, 144.14, 144.10, 143.98, 143.62, 143.57, 143.46, 143.41, 141.78, 141.72 (4C), 141.35, 141.26, 141.03, 140.98, 140.78, 140.50, 138.91, 138.67 (1C, aryl C), 138.58, 136.56, 136.18, 134.52 (1C, aryl C), 129.48 (1C, aryl C), 128.97 (1C, aryl C), 128.46 (1C, aryl C), 125.83 (1C, aryl C), 91.47 (1C, sp3-C of C60), 78.73 (1C, sp3-C of C60), 20.60 (1C); FT-IR ν/cm−1 (KBr) 2916, 2848, 1771, 1605, 1488, 1425, 1363, 1350, 1281, 1246, 1188, 1167, 1151, 1086, 1064, 1011, 955, 887, 807, 777, 742, 693, 574, 562, 553, 526; UV-vis (CHCl3) λmax/nm 257, 319, 418; HRMS (MALDI-TOF-MS with 4-hydroxy-α-cyanocinnamic acid as the matrix) m/z calcd for C68H7NO2 [M+] 869.0477, found 869.0476.
Synthesis of oxazolidinofullerene 2d
According to the general procedure, the reaction of C60 (36.0 mg, 0.05 mmol) with 1d (149 mg, 1.0 mmol) and Fe(ClO4)3·xH2O (69.0 mg, 0.15 mmol) for 24 h afforded first unreacted C60 (24.9 mg, 69%) and then 2d (8.9 mg, 20%) as amorphous black solid. mp > 300 °C; 1H NMR (500 MHz, CS2/CDCl3) δ 7.66 (d, J = 8.9 Hz, 2H), 7.01 (d, J = 8.9 Hz, 2H), 3.83 (s, 3H); 13C NMR (125 MHz, CS2/CDCl3) (all 2C unless indicated) δ 160.08 (1C, aryl C), 154.38 (1C, C
O), 148.37 (1C), 148.16 (1C), 146.55, 146.51, 146.48, 145.26 (4C), 146.24, 145.26, 145.19, 145.15, 144.98, 144.82, 144.64, 144.52, 144.48, 144.40, 142.83, 142.75 (4C), 142.39, 142.29, 142.03, 142.01, 141.82, 141.51, 139.97, 139.70, 137.41, 137.04, 130.96 (aryl C), 127.72 (1C, aryl C), 114.99 (aryl C), 92.65 (1C, sp3-C of C60), 80.17 (1C, sp3-C of C60), 55.19 (1C); FT-IR ν/cm−1 (KBr) 2973, 2927, 1769, 1607, 1510, 1459,1435, 1369, 1301, 1252, 1169, 1155, 1087, 1049, 1030, 1012, 954, 864, 828, 742, 628, 547, 526; UV-vis (CHCl3) λmax/nm 258, 318, 417; HRMS (MALDI-TOF-MS with 4-hydroxy-α-cyanocinnamic acid as the matrix) m/z calcd for C68H7NO3 [M+] 885.0426, found 885.0429.
Synthesis of oxazolidinofullerene 2e
According to the general procedure, the reaction of C60 (36.0 mg, 0.05 mmol) with 1e (141 μL, 1.0 mmol) and Fe(ClO4)3·xH2O (46.0 mg, 0.10 mmol) for 24 h afforded first unreacted C60 (25.9 mg, 72%) and then 2e (10.1 mg, 23%) as amorphous black solid. mp > 300 °C; 1H NMR (600 MHz, CS2/CDCl3) δ 7.35 (s, 2H), 7.08 (s, 1H), 2.39 (s, 6H); 13C NMR (100 MHz, CS2/CDCl3) (all 2C unless indicated) δ 154.51 (1C, C
O), 148.37 (1C), 148.17 (1C), 146.54, 146.51, 146.47, 146.26 (4C), 146.24, 145.27, 145.19, 145.14, 144.95, 144.76, 144.63, 144.52, 144.46, 144.39, 142.82, 142.75 (4C), 142.38, 142.29, 142.04, 142.00, 141.80, 141.48, 139.98, 139.66, 139.57 (aryl C), 137.42, 136.98, 135.01 (1C, aryl C), 131.23 (1C, aryl C), 127.26 (aryl C), 92.86 (1C, sp3-C of C60), 79.99 (1C, sp3-C of C60), 21.42; FT-IR ν/cm−1 (KBr) 2917, 2850, 1771, 1611, 1465, 1435, 1366, 1277, 1230, 1195, 1151, 1088, 1014, 956, 851, 797, 764, 690, 575, 527; UV-vis (CHCl3) λmax/nm 258, 318, 418; HRMS (MALDI-TOF-MS with 4-hydroxy-α-cyanocinnamic acid as the matrix) m/z calcd for C69H9NO2 [M+] 883.0633, found 883.0641.
Synthesis of oxazolidinofullerene 2f
According to the general procedure, the reaction of C60 (36.0 mg, 0.05 mmol) with 1f (154 mg, 1.0 mmol) and Fe(ClO4)3·xH2O (46.0 mg, 0.10 mmol) for 10 h afforded first unreacted C60 (28.1 mg, 78%) and then 2f (8.1 mg, 18%) as amorphous black solid. mp > 300 °C; 1H NMR (600 MHz, CS2/DMSO-d6) δ 7.79 (d, J = 8.5 Hz, 2H), 7.48 (d, J = 8.5 Hz, 2H); 13C NMR (125 MHz, CS2/DMSO-d6) (all 2C unless indicated) δ 152.13 (1C, C
O), 147.33 (1C), 147.11 (1C), 145.48 (4C), 145.43, 145.23 (6C), 144.26, 144.13 (4C), 143.77, 143.73, 143.62, 143.39 (6C), 141.80, 141.73 (4C), 141.35, 141.25, 141.02, 140.91, 140.81, 140.45, 138.92, 138.65, 136.62, 136.43, 134.45 (1C, aryl C), 133.16 (1C, aryl C), 130.29 (aryl C), 128.88 (aryl C), 91.62 (1C, sp3-C of C60), 78.57 (1C, sp3-C of C60); FT-IR ν/cm−1 (KBr) 1768, 1490, 1424, 1363, 1349, 1229, 1175, 1154, 1087, 1061, 1010, 953, 863, 820, 810, 736, 725, 541, 526; UV-vis (CHCl3) λmax/nm 257, 318, 419; HRMS (MALDI-TOF-MS with 4-hydroxy-α-cyanocinnamic acid as the matrix) m/z calcd for C67H4ClNO2 [M+] 888.9931, found 888.9937.
Synthesis of oxazolidinofullerene 2g
According to the general procedure, the reaction of C60 (36.0 mg, 0.05 mmol) with 1g (188 mg, 1.0 mmol) and Fe(ClO4)3·xH2O (46.0 mg, 0.10 mmol) for 5 h afforded first unreacted C60 (28.2 mg, 78%) and then 2g (4.1 mg, 9%) as amorphous black solid. mp > 300 °C; 1H NMR (600 MHz, CS2/DMSO-d6) δ 8.07 (s, 1H), 7.78 (d, J = 8.5 Hz, 1H), 7.62 (d, J = 8.5 Hz, 1H); 13C NMR (125 MHz, CS2/CDCl3) (all 2C unless indicated) δ 153.86 (1C, C
O), 148.41 (1C), 148.21 (1C), 146.58, 146.53 (4C), 146.38, 146.32 (4C), 145.21 (4C), 145.18, 144.65, 144.35, 144.32, 144.27, 144.05 (4C), 142.89, 142.80 (4C), 142.39, 142.31, 142.03, 141.88 (4C), 141.42, 140.06, 139.82, 137.30, 137.29, 134.72 (1C, aryl C), 134.16 (1C, aryl C), 134.10 (1C, aryl C), 131.47 (1C, aryl C), 131.30 (1C, aryl C), 128.66 (1C, aryl C), 93.20 (1C, sp3-C of C60), 79.55 (1C, sp3-C of C60); FT-IR ν/cm−1 (KBr) 1769, 1587, 1506, 1470, 1435, 1345, 1226, 1177, 1155, 1086, 1011, 954, 866, 765, 562, 541, 526; UV-vis (CHCl3) λmax/nm 257, 319, 417; HRMS (MALDI-TOF-MS with 4-hydroxy-α-cyanocinnamic acid as the matrix) m/z calcd for C67H3Cl2NO2 [M+] 922.9541, found 922.9539.
Fe(ClO4)3-mediated reaction of C60 with 4-nitrophenyl isocyanate 1h by the dissolution of Fe(ClO4)3 in 1h under nitrogen conditions
According to the general procedure for the preparation of oxazolidinofullerenes 2a–g, the reaction of C60 (36.0 mg, 0.05 mmol) with 1h (164 mg, 1.0 mmol) and Fe(ClO4)3·xH2O (46.0 mg, 0.10 mmol) for 25 h afforded first unreacted C60 (28.2 mg, 78%) and then aziridinofullerene 3 (ref. 16) (3.1 mg, 7%).
Fe(ClO4)3-mediated reaction of C60 with 4-nitrophenyl isocyanate 1h by the dissolution of Fe(ClO4)3 in acetonitrile under air conditions
A mixture of C60 (36.0 mg, 0.05 mmol) and 1h (164 mg, 1.0 mmol) was dissolved in o-dichlorobenzene (6 mL). After Fe(ClO4)3·xH2O (46.0 mg, 0.10 mmol, dissolved in 2 mL of acetonitrile) was added, the reaction mixture was vigorously stirred at 100 °C under air conditions for 40 h. The reaction mixture was passed through a silica gel plug to remove any insoluble material. After the solvent was evaporated in vacuo, the residue was separated on a silica gel column with carbon disulfide/toluene as the eluent to give unreacted C60 (28.0 mg, 78%) and then aziridinofullerene 3 (ref. 16) (8.1 mg, 19%).
Fe(ClO4)3-mediated reaction of C60 with 4-nitroaniline by the dissolution of Fe(ClO4)3 in acetonitrile under air conditions
According to the same procedure as for the preparation of aziridinofullerene 3 by 4-nitrophenyl isocyanate 1h under air conditions, the reaction of C60 (36.0 mg, 0.05 mmol) with 4-nitroaniline (138 mg, 1.0 mmol) and Fe(ClO4)3·xH2O (46.0 mg, 0.10 mmol) at 150 °C for 22 h afforded first unreacted C60 (30.2 mg, 84%) and then aziridinofullerene 3 (ref. 16) (6.0 mg, 14%).
Fe(ClO4)3-mediated reaction of C60 with 3,5-dimethylphenyl isocyanate 1e by the dissolution of Fe(ClO4)3 in 1e under air conditions
A mixture of 3,5-dimethylphenyl isocyanate 1e (141 μL, 1.0 mmol) and Fe(ClO4)3·xH2O (46.0 mg, 0.10 mmol) was added to a 50 mL round-bottom flask, which was heated in an oil bath preset at 100 °C for 10 min to allow ferric perchlorate to dissolve in the liquid isocyanate. Then to the flask was added the o-dichlorobenzene (6 mL) solution of C60 (36.0 mg, 0.05 mmol). The reaction mixture was vigorously stirred in the oil bath at the same temperature under air conditions for 24 h. The resulting solution was directly separated on a silica gel column with carbon disulfide/toluene as the eluent to give unreacted C60 (27.9 mg, 78%) and then oxazolidinofullerene 2e (7.1 mg, 16%).
General procedure for the Fe(ClO4)3-mediated reaction of C60 with isothiocyanates 4a–h
A mixture of isothiocyanate 4a (4b–h, 2.5 mmol, 1.0 mmol in the case of 4f) and Fe(ClO4)3·xH2O (0.25 mmol, 0.10 mmol in the case of 4f) was added to a 50 mL three-neck flask equipped with a reflux condenser, nitrogen inlet and outlet, and a magnetic stirrer. The mixture was heated in an oil bath preset at 100 °C (150 °C in the case of 4f) for 10 min to allow ferric perchlorate to dissolve in the liquid isothiocyanate. Then to the flask was added the o-dichlorobenzene (6 mL) solution of C60 (36.0 mg, 0.05 mmol). The resulting solution was heated with vigorous stirring in the oil bath at the same temperature under nitrogen atmosphere. The reaction was carefully monitored by thin-layer chromatography (TLC) and stopped at the designated time. The reaction mixture was passed through a silica gel plug to remove any insoluble material. After the solvent was evaporated in vacuo, the residue was separated on a silica gel column with carbon disulfide/toluene as the eluent. Thiazolidinofullerene 5a (5b–h) was obtained along with unreacted C60.
Fe(ClO4)3-mediated reaction of C60 with phenyl isothiocyanate 4a by the dissolution of Fe(ClO4)3 in 4a under different conditions
Fe(ClO4)3·xH2O (0.15–0.35 mmol) and 4a (1.5–5.0 mmol) was added to a 50 mL three-neck flask, which was equipped with a reflux condenser, nitrogen inlet and outlet, and a magnetic stirrer. The mixture was heated in an oil bath preset at desired temperature (80–120 °C) for 10 min to allow Fe(ClO4)3·xH2O to dissolve in liquid 4a. Then to the flask was added o-dichlorobenzene (6 mL) solution of C60 (36.0 mg, 0.05 mmol). The reaction mixture was vigorously stirred in the oil bath preset at the same temperature under nitrogen atmosphere. The reaction was monitored by TLC and stopped at the designated time (2–24 h). The resulting solution was passed through a silica gel plug to remove any insoluble material. After the solvent was evaporated in vacuo, the residue was separated on a silica gel column with carbon disulfide/toluene as the eluent to give unreacted C60 and thiazolidinofullerene 5a.
Fe(ClO4)3-mediated reaction of C60 with phenyl isothiocyanate 4a by the dissolution of Fe(ClO4)3 in acetonitrile
A mixture of C60 (36.0 mg, 0.05 mmol) and 4a (296 μL, 2.5 mmol) was dissolved in o-dichlorobenzene (6 mL), and then the resulting solution was deoxygenated by a nitrogen stream for 10 min. After Fe(ClO4)3·xH2O (115.0 mg, 0.25 mmol, dissolved in 2 mL of acetonitrile) was added, the reaction mixture was vigorously stirred at 100 °C under nitrogen atmosphere for 24 h. The resulting solution was passed through a silica gel plug to remove any insoluble material. After the solvent was evaporated in vacuo, the residue was separated on a silica gel column with carbon disulfide/toluene as the eluent to give first unreacted C60 (19.2 mg, 53%) and then thiazolidinofullerene 5a (2.3 mg, 5%).
Synthesis of thiazolidinofullerene 5a
According to the general procedure, the reaction of C60 (36.0 mg, 0.05 mmol) with 4a (296 μL, 2.5 mmol) and Fe(ClO4)3·xH2O (115.0 mg, 0.25 mmol) for 18 h afforded first unreacted C60 (16.1 mg, 45%) and then 5a (8.0 mg, 18%) as amorphous black solid. mp > 300 °C; 1H NMR (500 MHz, CS2/CDCl3) δ 7.63 (d, J = 7.8 Hz, 2H), 7.50 (t, J = 7.6 Hz, 2H), 7.44 (t, J = 7.4 Hz, 1H); 13C NMR (125 MHz, CS2/CDCl3) (all 2C unless indicated) δ 167.75 (1C, C
O), 150.81, 148.18 (1C), 147.87 (1C), 146.54, 146.32, 146.27, 146.19, 146.09, 145.70, 145.56, 145.26, 145.17, 144.69, 144.58, 144.38, 144.28, 143.10, 143.02, 142.76, 142.68, 142.27, 142.16, 142.02, 141.95, 141.60, 141.56, 140.37, 139.17, 137.04 (1C, aryl C), 136.03, 134.34, 131.21 (aryl C), 129.64 (aryl C), 129.54 (1C, aryl C), 84.05 (1C, sp3-C of C60), 66.34 (1C, sp3-C of C60); FT-IR ν/cm−1 (KBr) 2918, 2849, 1678, 1487, 1462, 1431, 1313, 1201, 1180, 1154, 1103, 1069, 1051, 882, 842, 814, 743, 690, 548, 524; UV-vis (CHCl3) λmax/nm 258, 319, 422; HRMS (MALDI-TOF-MS with 4-hydroxy-α-cyanocinnamic acid as the matrix) m/z calcd for C67H5NOS [M + Na]+ 893.9990, found 893.9990.
Synthesis of thiazolidinofullerene 5b
According to the general procedure, the reaction of C60 (36.0 mg, 0.05 mmol) with 4b (308 μL, 2.5 mmol) and Fe(ClO4)3·xH2O (115.0 mg, 0.25 mmol) for 4 h afforded first unreacted C60 (24.9 mg, 69%) and then 5b (11.1 mg, 25%) as an amorphous black solid. mp > 300 °C; 1H NMR (500 MHz, CS2/CDCl3) δ 7.63 (dd, J = 8.8, 4.8 Hz, 2H), 7.18 (t, J = 8.4 Hz, 2H); 13C NMR (125 MHz, CS2/CDCl3) (all 2C unless indicated) δ 167.99 (1C, C
O), 162.88 (d, JC–F = 251.5 Hz, 1C, aryl C), 150.68, 148.22 (1C), 147.92 (1C), 146.58, 146.37, 146.31, 146.23, 146.16, 145.67, 145.60, 145.31, 145.21, 144.72, 144.31, 144.29, 144.21, 143.07, 143.05, 142.81, 142.72, 142.27, 142.19, 142.03, 141.97, 141.66, 141.54, 140.42, 139.29, 136.14, 134.30, 133.10 (d, JC–F = 8.9 Hz, aryl C), 132.89 (d, JC–F = 3.4 Hz, 1C, aryl C), 116.80 (d, JC–F = 22.8 Hz, aryl C), 83.98 (1C, sp3-C of C60), 66.29 (1C, sp3-C of C60); FT-IR ν/cm−1 (KBr) 2918, 2849, 1686, 1596, 1504, 1463, 1434, 1315, 1226, 1199, 1150, 1104, 1090, 1050, 885, 860, 816, 551, 525; UV-vis (CHCl3) λmax/nm 257, 319, 421; HRMS (MALDI-TOF-MS with 4-hydroxy-α-cyanocinnamic acid as the matrix) m/z calcd for C67H4FNOS [M + Na]+ 911.9895, found 911.9884.
Synthesis of thiazolidinofullerene 5c
According to the general procedure, the reaction of C60 (36.0 mg, 0.05 mmol) with 4c (424 mg, 2.5 mmol) and Fe(ClO4)3·xH2O (115.0 mg, 0.25 mmol) for 1 h afforded first unreacted C60 (11.2 mg, 31%) and then 5c (29.7 mg, 66%) as an amorphous black solid. mp > 300 °C; 1H NMR (500 MHz, CS2/CDCl3) δ 7.60 (d, J = 8.7 Hz, 2H), 7.47 (d, J = 8.7 Hz, 2H); 13C NMR (125 MHz, CS2/CDCl3) (all 2C unless indicated) δ 168.00 (1C, C
O), 150.61, 148.23 (1C), 147.93 (1C), 146.59, 146.38, 146.32, 146.24, 146.18, 145.67, 145.61, 145.33, 145.22, 144.72, 144.30, 144.21, 144.18, 143.08, 143.04, 142.82, 142.73, 142.26, 142.19, 142.04, 141.97, 141.69, 141.53, 140.42, 139.34, 136.16, 136.12 (1C, aryl C), 135.50 (1C, aryl C), 134.30, 132.50 (aryl C), 130.06 (aryl C), 83.93 (1C, sp3-C of C60), 66.43 (1C, sp3-C of C60); FT-IR ν/cm−1 (KBr) 2917, 2849, 1687, 1487, 1463, 1434, 1314, 1199, 1156, 1104, 1087, 1048, 1015, 884, 856, 807, 549, 524; UV-vis (CHCl3) λmax/nm 257, 320, 422; HRMS (MALDI-TOF-MS with 4-hydroxy-α-cyanocinnamic acid as the matrix) m/z calcd for C67H4ClNOS [M + Na]+ 927.9600, found 927.9603.
Synthesis of thiazolidinofullerene 5d
According to the general procedure, the reaction of C60 (36.0 mg, 0.05 mmol) with 4d (535 mg, 2.5 mmol) and Fe(ClO4)3·xH2O (115.0 mg, 0.25 mmol) for 0.5 h afforded first unreacted C60 (10.2 mg, 28%) and then 5d (28.1 mg, 59%) as an amorphous black solid. mp > 300 °C; 1H NMR (400 MHz, CS2/DMSO-d6) δ 7.59 (d, J = 8.7 Hz, 2H), 7.52 (d, J = 8.7 Hz, 2H); 13C NMR (100 MHz, CS2/DMSO-d6) (all 2C unless indicated) δ 165.72 (1C, C
O), 149.75, 147.19 (1C), 146.92 (1C), 145.56, 145.35, 145.32, 145.21, 145.17, 144.74, 144.58, 144.31, 144.20, 143.73, 143.43, 143.35, 143.33, 142.14, 142.07, 141.80, 141.72, 141.30, 141.20, 141.08, 140.97, 140.72, 140.57, 139.36, 138.32, 135.38, 135.27 (1C, aryl C), 133.54, 132.31 (aryl C), 132.04 (aryl C), 123.45 (1C, aryl C), 82.62 (1C, sp3-C of C60), 65.38 (1C, sp3-C of C60); FT-IR ν/cm−1 (KBr) 2918, 2849, 1683, 1484, 1463, 1432, 1314, 1198, 1155, 1102, 1065, 1012, 885, 854, 804, 549, 524; UV-vis (CHCl3) λmax/nm 257, 320, 422; HRMS (MALDI-TOF-MS with 4-hydroxy-α-cyanocinnamic acid as the matrix) m/z calcd for C67H4BrNOS [M + Na]+ 971.9095, found 971.9087.
Synthesis of thiazolidinofullerene 5e
According to the general procedure, the reaction of C60 (36.0 mg, 0.05 mmol) with 4e (508 mg, 2.5 mmol) and Fe(ClO4)3·xH2O (115.0 mg, 0.25 mmol) for 2 h afforded first unreacted C60 (20.9 mg, 58%) and then 5e (12.1 mg, 26%) as an amorphous black solid. mp > 300 °C; 1H NMR (600 MHz, CS2/CDCl3) δ 7.83 (d, J = 8.4 Hz, 2H), 7.79 (d, J = 8.4 Hz, 2H); 13C NMR (125 MHz, CS2/CDCl3) (all 2C unless indicated) δ 168.53 (1C, C
O), 150.54, 148.35 (1C), 148.05 (1C), 146.71, 146.51, 146.44, 146.36, 146.32, 145.75, 145.72, 145.46, 145.33, 144.83, 144.37, 144.04 (4C), 143.20, 143.08, 142.94, 142.85, 142.33, 142.29, 142.13, 142.08, 141.79, 141.60, 140.56, 140.33 (1C, aryl C), 139.48, 136.37, 134.39, 131.89 (q, JC–F = 32.8 Hz, 1C, aryl C), 131.87 (aryl C), 127.02 (q, JC–F = 3.6 Hz, aryl C), 123.45 (q, JC–F = 271.4 Hz, 1C), 83.98 (1C, sp3-C of C60), 66.80 (1C, sp3-C of C60); FT-IR ν/cm−1 (KBr) 2919, 2850, 1689, 1462, 1435, 1322, 1261, 1165, 1125, 1103, 1064, 1041, 1020, 817, 806, 542, 526; UV-vis (CHCl3) λmax/nm 258, 320, 422; HRMS (MALDI-TOF-MS with 4-hydroxy-α-cyanocinnamic acid as the matrix) m/z calcd for C68H4F3NOS [M + Na]+ 961.9863, found 961.9870.
Synthesis of thiazolidinofullerene 5f
According to the general procedure, the reaction of C60 (36.0 mg, 0.05 mmol) with 4f (180 mg, 1.0 mmol) and Fe(ClO4)3·xH2O (46.0 mg, 0.10 mmol) at 150 °C for 0.5 h afforded first unreacted C60 (8.0 mg, 22%) and then 5f (6.9 mg, 15%) as an amorphous black solid. mp > 300 °C; 1H NMR (600 MHz, CS2/CDCl3) δ 8.37 (d, J = 8.8 Hz, 2H), 7.89 (d, J = 8.8 Hz, 2H); 13C NMR (125 MHz, CS2/CDCl3) (all 2C unless indicated) δ 168.87 (1C, C
O), 150.40, 148.46 (1C), 148.38 (1C, aryl C), 148.14 (1C), 146.81, 146.60, 146.52, 146.46, 146.43, 145.81, 145.78, 145.56, 145.42, 144.90, 144.41, 143.85, 143.76, 143.29, 143.08, 143.04, 142.94, 142.82 (1C, aryl C), 142.36 (4C), 142.18, 142.16, 141.85, 141.62, 140.66, 139.56, 136.55, 134.41, 132.59 (aryl C), 125.19 (aryl C), 84.02 (1C, sp3-C of C60), 67.07 (1C, sp3-C of C60); FT-IR ν/cm−1 (KBr) 2922, 2850, 1678, 1606, 1591, 1521, 1490, 1427, 1342, 1313, 1261, 1199, 1155, 1124, 1101, 852, 829, 811, 742, 574, 549, 525; UV-vis (CHCl3) λmax/nm 257, 320, 422; HRMS (MALDI-TOF-MS with 4-hydroxy-α-cyanocinnamic acid as the matrix) m/z calcd for C67H4N2O3S [M + Na]+ 938.9840, found 938.9851.
Synthesis of thiazolidinofullerene 5g
According to the general procedure, the reaction of C60 (36.0 mg, 0.05 mmol) with 4g (338 uL, 2.5 mmol) and Fe(ClO4)3·xH2O (115.0 mg, 0.25 mmol) for 4 h afforded first unreacted C60 (24.9 mg, 69%) and then 5g (8.1 mg, 18%) as an amorphous black solid. mp > 300 °C; 1H NMR (600 MHz, CS2/DMSO-d6) δ 7.39 (s, 1H), 7.39 (d, J = 7.3 Hz, 1H), 7.34 (t, J = 7.3 Hz, 1H), 7.22 (d, J = 6.4 Hz, 1H), 2.40 (s, 3H); 13C NMR (100 MHz, CS2/CDCl3) (all 2C unless indicated) δ 166.78 (1C, C
O), 150.08, 147.39 (1C), 147.08 (1C), 145.75, 145.53, 145.49, 145.40, 145.29, 144.93, 144.77, 144.47, 144.40, 143.91, 143.86, 143.72, 143.50, 142.33, 142.24, 141.97, 141.90, 141.49, 141.38, 141.25, 141.17, 140.84, 140.79, 139.59, 139.00 (1C, aryl C), 138.38, 136.21 (1C, aryl C), 135.19, 133.56, 130.89 (1C, aryl C), 129.70 (1C, aryl C), 128.84 (1C, aryl C), 127.42 (1C, aryl C), 83.22 (1C, sp3-C of C60), 65.51 (1C, sp3-C of C60), 20.88 (1C); FT-IR ν/cm−1 (KBr) 2918, 2849, 1682, 1601, 1488, 1449, 1428, 1314, 1261, 1230, 1174, 1154, 1101, 1069, 775, 761, 691, 574, 548, 524; UV-vis (CHCl3) λmax/nm 257, 320, 422; HRMS (MALDI-TOF-MS with 4-hydroxy-α-cyanocinnamic acid as the matrix) m/z calcd for C68H7NOS [M + Na]+ 908.0146, found 908.0143.
Synthesis of thiazolidinofullerene 5h
According to the general procedure, the reaction of C60 (36.0 mg, 0.05 mmol) with 4h (345 uL, 2.5 mmol) and Fe(ClO4)3·xH2O (115.0 mg, 0.25 mmol) for 14 h afforded first unreacted C60 (15.2 mg, 42%) and then 5h (5.1 mg, 11%) as an amorphous black solid. mp > 300 °C; 1H NMR (600 MHz, CS2/CDCl3) δ 7.52 (d, J = 9.0 Hz, 2H), 6.96 (d, J = 9.0 Hz, 2H), 3.80 (s, 3H); 13C NMR (100 MHz, CS2/CDCl3) (all 2C unless indicated) δ 168.36 (1C, C
O), 159.92 (1C, aryl C), 150.59, 147.97 (1C), 147.69 (1C), 146.33, 146.12, 146.07, 145.98, 145.89, 145.49, 145.34, 145.06, 144.98, 144.47, 144.39, 144.28, 144.08, 142.90, 142.81, 142.54, 142.47, 142.05, 141.94, 141.81, 141.73, 141.42, 141.34, 140.13, 139.02, 135.77, 134.15, 132.00 (aryl C), 129.19 (1C, aryl C), 114.80 (aryl C), 84.17 (1C, sp3-C of C60), 65.83 (1C, sp3-C of C60), 55.10; FT-IR ν/cm−1 (KBr) 2922, 2850, 1686, 1606, 1507, 1462, 1436, 1326, 1297, 1250, 1182, 1156, 1105, 1034, 814, 765, 618, 562, 550, 526; UV-vis (CHCl3) λmax/nm 257, 321, 427; HRMS (MALDI-TOF-MS with 4-hydroxy-α-cyanocinnamic acid as the matrix) m/z calcd for C68H7NO2S [M + Na]+ 924.0095, found 924.0097.
Fe(ClO4)3-mediated reaction of C60 with 4-fluorophenyl isothiocyanate 4b by the dissolution of Fe(ClO4)3 in 4b under air conditions
According to the general procedure for the preparation of thiazolidinofullerenes 5a–h, the reaction of C60 (36.0 mg, 0.05 mmol) with 4b (308 μL, 2.5 mmol) and Fe(ClO4)3·xH2O (115.0 mg, 0.25 mmol) under air conditions at 100 °C for 4 h afforded first unreacted C60 (29.1 mg, 81%) and then thiazolidinofullerene 5b (5.2 mg, 12%) as an amorphous black solid.
Acknowledgements
The authors are grateful for the financial support from National Natural Science Foundation of China (Nos. 21102041 and 21071049), Natural Science Foundation of Hubei Province (No. 2013CFA087), Natural Science Fund for Creative Research Groups of Hubei Province of China (No. 2014CFA015), Scientific Research Foundation of Education Commission of Hubei Province (No. Q20120113), and Innovation and Entrepreneurship Training Program for Undergraduates of Hubei Province (No. 201310512011).
Notes and references
- For selected recent reviews, see:
(a) F. Giacalone and N. Martín, Chem. Rev., 2006, 106, 5136 CrossRef CAS PubMed;
(b) Y. Matsuo and E. Nakamura, Chem. Rev., 2008, 108, 3016 CrossRef CAS PubMed;
(c) M. Murata, Y. Murata and K. Komatsu, Chem. Commun., 2008, 6083 RSC For some recent papers, see: ;
(d) N. Xin, H. Huang, J. Zhang, Z. Dai and L. Gan, Angew. Chem., Int. Ed., 2012, 51, 6153 Search PubMed;
(e) W.-W. Chang, Z.-J. Li and X. Gao, Org. Lett., 2013, 15, 1642 CrossRef CAS PubMed.
- M. D. Tzirakis and M. Orfanopoulos, Chem. Rev., 2013, 113, 5262 CrossRef CAS PubMed , and references therein.
- For selected examples, see:
(a) I. E. Kareev, I. V. Kuvychko, S. F. Lebedkin, S. M. Miller, O. P. Anderson, K. Seppelt, S. H. Strauss and O. V. Boltalina, J. Am. Chem. Soc., 2005, 127, 8362 CrossRef CAS PubMed;
(b) S. Wang, P. Yan, H.-Y. Huang, Z.-P. Zhan, S.-Y. Xie, R.-B. Huang and L.-S. Zheng, Chem.–Asian J., 2012, 7, 2531 CrossRef CAS PubMed.
- For reviews, see:
(a) G.-W. Wang and F.-B. Li, J. Nanosci. Nanotechnol., 2007, 7, 1162 CrossRef CAS PubMed;
(b) G.-W. Wang and F.-B. Li, Curr. Org. Chem., 2012, 16, 1109 CrossRef CAS;
(c) F.-B. Li and G.-W. Wang, Sci. China: Chem., 2012, 55, 2009 CrossRef CAS.
-
(a) T.-H. Zhang, P. Lu, F. Wang and G.-W. Wang, Org. Biomol. Chem., 2003, 1, 4403 RSC;
(b) G.-W. Wang, T.-H. Zhang, X. Cheng and F. Wang, Org. Biomol. Chem., 2004, 2, 1160 RSC;
(c) C. Li, D. Zhang, X. Zhang, S. Wu and X. Gao, Org. Biomol. Chem., 2004, 2, 3464 RSC;
(d) G.-W. Wang and F.-B. Li, Org. Biomol. Chem., 2005, 3, 794 RSC;
(e) G.-W. Wang, H.-T. Yang, C.-B. Miao, Y. Xu and F. Liu, Org. Biomol. Chem., 2006, 4, 2595 RSC;
(f) G.-W. Wang, F.-B. Li and T.-H. Zhang, Org. Lett., 2006, 8, 1355 CrossRef CAS PubMed;
(g) F.-B. Li, T.-X. Liu, Y.-S. Huang and G.-W. Wang, J. Org. Chem., 2009, 74, 7743 CrossRef CAS PubMed;
(h) F.-B. Li, S.-E. Zhu and G.-W. Wang, Chin. Sci. Bull., 2010, 55, 2909 CrossRef CAS;
(i) G.-W. Wang, C.-Z. Wang, S.-E. Zhu and Y. Murata, Chem. Commun., 2011, 47, 6111 RSC;
(j) G.-W. Wang, C.-Z. Wang and J.-P. Zou, J. Org. Chem., 2011, 76, 6088 CrossRef CAS PubMed;
(k) T.-X. Liu, F.-B. Li and G.-W. Wang, Org. Lett., 2011, 13, 6130 CrossRef CAS PubMed;
(l) Z.-X. Chen and G.-W. Wang, J. Org. Chem., 2005, 70, 2380 CrossRef CAS PubMed.
-
(a) F.-B. Li, T.-X. Liu and G.-W. Wang, J. Org. Chem., 2008, 73, 6417 CrossRef CAS PubMed;
(b) F.-B. Li, T.-X. Liu, X. You and G.-W. Wang, Org. Lett., 2010, 12, 3258 CrossRef CAS PubMed;
(c) F.-B. Li, X. You and G.-W. Wang, Org. Lett., 2010, 12, 4896 CrossRef CAS PubMed;
(d) F.-B. Li, X. You, T.-X. Liu and G.-W. Wang, Org. Lett., 2012, 14, 1800 CrossRef CAS PubMed;
(e) F.-B. Li, X. You and G.-W. Wang, J. Org. Chem., 2012, 77, 6643 CrossRef CAS PubMed;
(f) F.-B. Li, S.-E. Zhu, X. You and G.-W. Wang, Chin. Sci. Bull., 2012, 57, 2269 CrossRef CAS PubMed.
-
(a) L. Gan, S. Huang, X. Zhang, A. Zhang, B. Cheng, H. Cheng, X. Li and G. Shang, J. Am. Chem. Soc., 2002, 124, 13384 CrossRef CAS PubMed;
(b) M. Hashiguchi, K. Watanabe and Y. Matsuo, Org. Biomol. Chem., 2011, 9, 6417 RSC;
(c) M. Hashiguchi, N. Obata, M. Maruyama, K. S. Yeo, T. Ueno, T. Ikebe, I. Takahashi and Y. Matsuo, Org. Lett., 2012, 14, 3276 CrossRef CAS PubMed;
(d) M. Hashiguchi, H. Inad and Y. Matsuo, Carbon, 2013, 61, 418 CrossRef CAS PubMed;
(e) Y.-T. Su and G.-W. Wang, Org. Lett., 2013, 15, 3408 CrossRef CAS PubMed;
(f) X. You and G.-W. Wang, J. Org. Chem., 2014, 79, 117 CrossRef CAS PubMed.
-
(a) M. D. Tzirakis and M. Orfanopoulos, Org. Lett., 2008, 10, 873 CrossRef CAS PubMed;
(b) M. D. Tzirakis and M. Orfanopoulos, J. Am. Chem. Soc., 2009, 131, 4063 CrossRef CAS PubMed;
(c) M. D. Tzirakis and M. Orfanopoulos, Angew. Chem., Int. Ed., 2010, 49, 5891 CrossRef CAS PubMed;
(d) M. D. Tzirakis, M. N. Alberti and M. Orfanopoulos, Chem. Commun., 2010, 46, 8228 RSC.
-
(a) S. Lu, T. Jin, E. Kwon, M. Bao and Y. Yamamoto, Angew. Chem., Int. Ed., 2012, 51, 802 CrossRef CAS PubMed;
(b) T.-X. Liu, Z. Zhang, Q. Liu, P. Zhang, P. Jia, Z. Zhang and G. Zhang, Org. Lett., 2014, 16, 1020 CrossRef CAS PubMed.
- S.-C. Chuang, F. R. Clemente, S. I. Khan, K. N. Houk and Y. Rubin, Org. Lett., 2006, 8, 4525 CrossRef CAS PubMed.
-
(a) S. Lu, T. Jin, M. Bao and Y. Yamamoto, J. Am. Chem. Soc., 2011, 133, 12842 CrossRef CAS PubMed;
(b) S. Lu, W. Si, M. Bao, Y. Yamamoto and T. Jin, Org. Lett., 2013, 15, 4030 CrossRef CAS PubMed.
- C.-L. He, R. Liu, D.-D. Li, S.-E. Zhu and G.-W. Wang, Org. Lett., 2013, 15, 1532 CrossRef CAS PubMed.
-
(a) M. R. Banks, J. I. G. Cadogan, I. Gosney, P. K. G. Hodgson, P. R. R. Langridge-Smith, J. R. A. Millar and A. T. Taylor, Tetrahedron Lett., 1994, 35, 9067 CrossRef CAS;
(b) L.-L. Shiu, K.-M. Chien, T.-Y. Liu, T.-I. Lin, G.-R. Her, S.-L. Huang and T.-Y. Luh, J. Chem. Soc., Perkin Trans. 1, 1994, 3355 RSC;
(c) Y. Takeda, H. Kawai and S. Minakata, Chem.–Eur. J., 2013, 19, 13479 CrossRef CAS PubMed.
- X. Yang, S. Huang, Z. Jia, Z. Xiao, Z. Jiang, Q. Zhang, L. Gan, B. Zheng, G. Yuan and S. Zhang, J. Org. Chem., 2008, 73, 2518 CrossRef CAS PubMed.
-
(a) T. A. Mukhtar and G. D. Wright, Chem. Rev., 2005, 105, 529 CrossRef CAS PubMed;
(b) T. Kato, T. Ozaki, K. Tamura, Y. Suzuki, M. Akima and N. Ohi, J. Med. Chem., 1999, 42, 3134 CrossRef CAS PubMed.
- H.-T. Yang, X.-C. Liang, Y.-H. Wang, Y. Yang, X.-Q. Sun and C.-B. Miao, J. Org. Chem., 2013, 78, 11992 CrossRef CAS PubMed.
- T. Baronsky, C. Beattie, R. W. Harrington, R. Irfan, M. North, J. G. Osende and C. Young, ACS Catal., 2013, 3, 790 CrossRef CAS and references therein.
-
(a) J. H. Saunders and R. J. Slocombe, Chem. Rev., 1948, 43, 203 CrossRef CAS;
(b) R. G. Arnold, J. A. Nelson and J. J. Verbanc, Chem. Rev., 1957, 57, 47 CrossRef CAS.
- A. Citterio, R. Sebastiano and M. C. Carvayal, J. Org. Chem., 1991, 56, 5335 CrossRef CAS.
Footnote |
† Electronic supplementary information (ESI) available: NMR spectra of products 2a–g, 3, and 5a–h, UV-vis spectra of 2b and 5f as well as HRMS of 2a and 5a. See DOI: 10.1039/c4ra10199e |
|
This journal is © The Royal Society of Chemistry 2014 |
Click here to see how this site uses Cookies. View our privacy policy here.