Quantitation of organic acids in wine and grapes by direct infusion electrospray ionization mass spectrometry†
Received
13th January 2014
, Accepted 24th September 2014
First published on 25th September 2014
Abstract
Several organic acids such as malic, tartaric and citric acids are key grape and wine constituents. They greatly contribute to the organoleptic properties of grapes and wines and are responsible for their acidity, a crucial wine property that is known to considerably vary as a function of grape variety, environmental conditions and viticulture, as well as during wine maturation. A rapid and simple method, requiring minimum sample pre-treatment and no chromatographic separation, based on direct infusion electrospray ionization mass spectrometry (ESI-MS) was developed and validated to quantitate organic acids in wines and grapes. This method was also demonstrated to be able to monitor a common wine adulteration procedure, that is, addition of citric acid for pH adjustment. ESI-MS data when treated via principal component analysis (PCA) was also found to group wine samples based on their profiles and concentrations of organic acids.
1. Introduction
Organic acids greatly contribute to the organoleptic quality of wines and grapes and their preservative properties increase the physical and chemical stability of this highly appreciated and widely consumed beverage. Being produced from grapes, the organic acids found in wine are mainly those found in grapes such as malic and tartaric acids, which are the main components responsible for the acidity (ca. pH 3) and the balance of the gustatory characteristics of wine.1 Grapes are one of the few fruits where the l isomer (+) of tartaric acid is found in high amounts, with most of the acid taste of wine due to this acid. The l-(−) isomer of malic acid is found in leaves and fruits and is very common in nature but has little resistance to oxidation. Although present in small amounts in grapes and wines, citric acid plays an important role in the Krebs cycle, inhibits the growth of yeasts, and is therefore frequently used as an acidifying agent in food and beverages. Hence, an excessive amount of citric acid in wine indicates adulteration. The analysis of organic acids in wines is necessary for quality control as well as to monitor the evolution of acidity during the different stages of winemaking since important changes in wine may be detected by alterations in the acid content.2
The level of tartaric acid is also a critical control parameter in the stabilization of wine. Malic and tartaric acids are dominant in grapes and their levels are often used to determine the date of harvest, since each acid behaves differently during the maturation process. The content of malic acid declines continuously during the ripening process whereas the content of tartaric acid remains nearly constant resulting in different ratios between these acids during ripening. The ideal harvest date of grapes may be established based on the malic/tartaric acid ratio3 and the acid profile and concentration have also been found to correlate with the grape variety, region of winemaking, processing techniques (alcoholic and malolactic fermentation) and maturation.4
Various techniques have been used to identify and quantify organic acids in grapes, juice and wine, such as chromatography, electrophoresis and spectrophotometry.5–7 Although capillary electrophoresis has been shown to be faster and may be used in a broader analytical range,8,9 liquid chromatography seems to be more sensitive and precise.4,10
Direct infusion electrospray ionization mass spectrometry (ESI-MS) has been offered as an attractive alternative to characterize complex chemical mixtures. The main advantages of ESI-MS are that neither chromatographic separation nor derivatization is needed, having minimal or no sample pretreatment at all, being also relatively simple and rapid. Our group and others have been successfully applying this method for the fingerprinting characterization of several complex mixtures such as those found in propolis,11 plant extracts,12 wine,4,13–15 coffee,16 biodiesel,17 fruit juices,18,19 and essential oils.20
We have already applied direct infusion ESI-MS fingerprinting for wine analysis, more specifically to characterize samples of must from six grape varieties during the fermentation process and samples of their respective wines after malolactic fermentation.21 Chemometric analysis of wine ESI-MS fingerprints was shown to group the samples and define diagnostic ions. The ESI-MS fingerprints also reflected the changes in composition due to the fermentation process. This method was also used to monitor the aging process of wines produced from diverse varieties of grapes13 where PCA of the ESI-MS data was found to group the samples by pinpointing markers for each group. But in these studies the quantitation abilities of the method was not tested since it was applied mainly for wine typification and quality control.
The aim of the present study was therefore to test the ability of this rapid and simple direct infusion ESI-MS fingerprinting method as a step further in wine analysis. An analytical method was developed and validated, not only to typify wines by their organic acid contents but also to quantitate these organic acids both in wine and grapes.
2. Materials and methods
2.1. Reagents
The standards used had a purity exceeding 99.0%: l-(−) malic acid, tartaric acid and citric acid (Sigma Aldrich). Stock solutions of these acids were prepared in a de-acidified red wine (DARW) at a concentration of 1000 μg mL−1. Calibration curves were prepared from these stock solutions.
DARW was prepared from red wine and the acids were washed out using SPE cartridges. These C18 SPE cartridges (Supelco) were initially activated with 1 mL of methanol (analytical grade, JT Baker, 99.97%), then rinsed with 1 mL of ultrapure water (Milli-Q, Millipore). Subsequently, 3 mL of red wine was loaded on the cartridge and washed with 3 mL of ultrapure water to remove the acids. The matrix retained in the cartridges was then extracted with 3 mL methanol and stored at 6 °C in a refrigerator.
2.2. Wine samples
As Table 1 summarizes, samples of wines from different countries produced from the Syrah variety of Vitis vinifera, as well as samples of wine from Paulista hybrid cultivar (Maximum IAC 138-22) and Brazilian wines from other grape varieties such as Carmen, Merlot and Bordô, were used. Bordô is a common denomination for Vitis labrusca grapes cultivated in the south of Brazil.22
Table 1 Concentrations of tartaric, malic and citric acids in samples of Brazilian and imported red wines as measured by direct infusion ESI(−)-MS (mg mL−1)a
Wine |
Grape |
Origin |
Malic acid |
Tartaric acid |
Citric acid |
Bordô* – Brazilian denomination for Vitis labrusca grapes cultivated in the south of Brazil.
|
1 |
Bordô* |
RS/Brazil |
2.79 |
7.63 |
1.82 |
2 |
Bordô* + Merlot |
RS/Brazil |
2.88 |
9.94 |
3.88 |
3 |
Bordô* + Merlot |
RS/Brazil |
2.05 |
2.58 |
1.77 |
4 |
Syrah |
New Zealand |
4.18 |
5.48 |
1.70 |
5 |
Isabel + Bordô* |
RS/Brazil |
5.07 |
5.93 |
2.77 |
6 |
Syrah |
France |
5.38 |
4.91 |
2.62 |
7 |
Carmen |
RS/Brazil |
2.75 |
4.24 |
2.48 |
8 |
Merlot |
RS/Brazil |
6.30 |
3.01 |
1.49 |
9 |
Syrah |
Australia |
2.99 |
3.47 |
1.47 |
10 |
Syrah |
Italy |
4.56 |
3.49 |
1.61 |
11 |
Syrah |
Chile |
5.01 |
4.60 |
1.74 |
12 |
Syrah |
Greece |
4.58 |
2.83 |
3.59 |
13 |
Syrah |
Portugal |
3.61 |
5.55 |
1.78 |
14 |
Bordô* |
SP/Brazil |
4.16 |
4.52 |
1.35 |
15 |
Isabel |
SP/Brazil |
8.72 |
3.56 |
1.43 |
16 |
Syrah |
Argentina |
4.49 |
6.73 |
2.85 |
17 |
Syrah |
Portugal |
4.57 |
5.52 |
2.19 |
18 |
Syrah |
South Africa |
2.81 |
4.34 |
1.82 |
19 |
Syrah |
Vale São Francisco, Brazil |
4.20 |
3.74 |
1.39 |
20 |
Syrah |
Chile |
4.79 |
6.32 |
2.46 |
21 |
Syrah |
Argentina |
3.38 |
4.44 |
1.54 |
22 |
Maximum |
SP/Brazil |
2.90 |
3.66 |
1.28 |
23 |
Syrah |
Chile |
3.16 |
4.44 |
1.45 |
24 |
Maximum |
SP/Brazil |
3.15 |
4.61 |
1.21 |
25 |
Syrah |
France |
7.46 |
4.23 |
4.32 |
26 |
Merlot |
RS/Brazil |
6.37 |
3.02 |
1.67 |
2.3. Grape samples
Samples of Carmen BRS-hybrid grapes, derived from Muscat Belly and BRS-Rúbea developed by the Brazilian Agricultural Research (Embrapa) were donated by the Corol Cooperative juice industry, located in Rolândia, Parana State, Brazil. Samples of Bordô grapes (Vitis labrusca) were purchased from farmers in Santa Catarina State, Brazil. The other grape samples: a hybrid (Maximum IAC 138-22), an American (Niagara) and Vitis vinifera (Syrah) were donated by the College of Agricultural Engineering of Campinas (FEAGRI), located in Campinas, São Paulo State, Brazil. The grape samples were frozen at −20 °C until analysis.
2.4. Preparation, extraction and recovery of samples of grapes
An ultrasound assisted extraction method was used (UAE) to extract and isolate organic acids from the grapes. Samples of approximately 2.10 g of skins, pulp and seeds of grapes were separately weighed, and lyophilized. Aliquots of 100 mg of the frozen lyophilized samples were ground in the presence of liquid N2 and subsequently extracted with 1 mL of methanol
:
water (1
:
1) solution, stirred using a sorbex (BenchMixer-Benchmark) mixer for 1 min and then placed in an ultrasonic bath (UNIQUE computed 1400) for 30 min. This material was then centrifuged for 2 min and the supernatant was separated. The extraction was repeated two more times on the remaining precipitate and the supernatants were pooled. These extracts were filtered through a 22 μm membrane and diluted again with methanol
:
water (1
:
1) before ESI-MS analysis in different proportions, because the dilution was optimized for each part of the grapes. The validation of the recovery of tartaric and malic acids from this material was performed using extracts of skins and Syrah grapes at three concentration levels: low (1 mg mL−1), medium (2 mg mL−1) and high (3 mg mL−1).
2.5. ESI-MS
Samples were analyzed by direct infusion ESI-MS with an Acquity TQD mass spectrometer (Waters, Manchester, UK) in negative ion mode under the following conditions: capillary voltage −3.5 kV, cone −30 V, source temperature 150 °C, desolvation temperature 350 °C, mass range 100–620. An aliquot of 10 μL of wine was dissolved in 990 μL of methanol containing 0.1% ammonium hydroxide solution (Hexis). The extracts of skins, pulp and seeds were diluted with methanol
:
water in the proportion of 1
:
25, 1
:
100 and 1
:
40, respectively. All samples were analyzed in triplicate.
2.6. Statistical analysis
Principal component analysis (PCA) was performed using the Pirouette software version 2.60 from Infometrix, Woodinville, WA, USA.
2.7. Validation of the method
After defining the best conditions for MS analysis and sample dilutions, the ESI-MS method was validated in terms of selectivity, accuracy, linearity, precision and limits of detection (LOD) and quantification (LOQ).23,24
2.7.1. Selectivity.
Two sets of standard solutions in the concentration range from 1.25 to 10.00 μg mL−1 were analyzed: the first group was prepared with standard solutions of malic acid, tartaric acid and citric acid in methanol and the second set of solutions was prepared in the de-acidified wine. Each solution for each concentration was prepared in triplicate to assess the matrix effect. A simple statistical test (the F test) was applied. The ratio between the variances of the two samples (F = s12/s22) was calculated and compared with the critical value of F at a given confidence level of 95% for three acids.24
2.7.2. Accuracy.
Three samples of wine were fortified with the organic acids at concentrations of 1.25 μg mL−1, 2.50 μg mL−1 and 5.00 μg mL−1. These samples were also prepared and injected in triplicate. The accuracy of the analytical method was expressed by the ratio between the experimentally determined average and the corresponding theoretical concentration, with the result given as recovery %.24
2.7.3. Linearity.
The linear range was established using five different solutions with concentrations obtained from dilutions of the stock solution of 1000 μg mL−1 of each acid (malic, tartaric and citric acids) de-acidified wine as follows: 1.25 μg mL−1, 2.50 μg mL−1, 5.00 μg mL−1, 7.50 μg mL−1 and 10.00 μg mL−1. The samples were prepared and analyzed in triplicate and the analytical curve for each acid was obtained by correlation between the concentration and area of the analyte signal through the linear least squares model (Origin 6.0).
2.7.4. Limits of detection (LOD) and quantification (LOQ).
The limit of detection (LOD) was calculated as the lowest detectable concentration (and not necessarily quantified) whereas the limit of quantification (LOQ) was the lowest concentration of the acid of interest that could be measured. The LOD and LOQ parameters were calculated based on the calibration curve, and expressed as:
LOD = 3, 3 × s/S; LOQ = 10 × s/S |
where ‘s’ is the estimated standard deviation of the regression line equation and S is the slope of the calibration curve.24
2.7.5. Precision.
Precision, defined as the evaluation of the closeness of the results obtained from a series of measurements of multiple sampling of the same sample, was herein considered at three levels: through repeatability, intermediate precision and reproducibility, and was expressed by the standard deviation and coefficient of variation.
2.7.6. Repeatability (intra-run precision).
The repeatability, which can be expressed quantitatively in terms of characteristic dispersion of the results of areas, was obtained by integration of the analytical signals. The intra-run precision was assessed by analysis of the standard solutions prepared in de-acidified wine at three concentration levels (2.0 μg mL−1, 4.0 μg mL−1 and 8.0 μg mL−1). Five replicates of each level of acid concentration were analyzed, thereby obtaining values of five areas with their respective standard deviations.24
2.7.7. Intermediate (inter-run) precision.
To determine the intermediate precision, 5 replicates were analyzed at three concentration levels: low (2 μg mL−1), medium (4 μg mL−1) and high (8 μg mL−1) for each acid on different days by the same analyst. For each level of acid concentration, five values were calculated as well as their respective standard deviations.24
2.8. High resolution MS data
HRMS data were acquired using a 7.2T LTQ FT Ultra mass spectrometer (Thermo Scientific, Bremen, Germany) equipped with a direct infusion electrospray ionization source (ESI) operating in negative ion mode under the follow conditions: capillary voltage – 3.1 kV, tube lens – 160 V, temperature 280 °C. Data acquisition was performed along the m/z 100–1000 range by using the Xcalibur 2.0 software. Wine samples were prepared by dissolving the wine in methanol (1
:
1). For ESI(−)-MS, an aqueous solution of 0.1% ammonium hydroxide was added to facilitate deprotonation of more acidic molecules (M) to form [M − H]− ions.
2.9. Addition of citric acid to simulate adulteration
Using the stock solution of citric acid, pure wine samples were spiked with specified concentrations of citric acid between 1.0 and 100.0 mg L−1. Since Brazilian legislation fails to determine the maximum amount of citric acid that may be added to wine, the purpose of this study was to determine the LOD of citric acid that could be detected by direct infusion ESI(−)-MS.
3. Results and discussion
3.1. Method validation
The selectivity of direct infusion ESI(−)-MS was satisfactory for all three organic acids measured. The F test showed no significant difference between the variances of the set of samples diluted with methanol and with de-acidified wine. The matrix effect failed to affect the results, since the F value calculated for the three acids was less than the critical F (Table S1†). In complex matrixes, such as wine, co-ionization of several molecules affects the ESI efficiency of each individual molecule, usually reducing the relative abundance of the respective ion. Although the average areas of the ion peaks in methanol were greater than those using de-acidified wine, the differences were not significant (F test). Although we ran our calibration curves using de-acidified wine, solutions of malic and tartaric acid standards in methanol/water could also be used to build the calibration curves, further simplifying the proposed method.
Table S2† shows the results of linearity, LOD, LOQ and accuracy, expressed as percent recovery. The ESI-MS method showed good linearity with R2 values higher than 0.993 in all cases. The LOD between 0.278 and 0.711 μg mL−1 and the LOQ between 0.843 and 2.157 μg mL−1 were relatively low, and similar to values reported for these acids using other techniques.9,10 The standard deviations were lower than 5%, which demonstrates satisfactory repeatability and instrumental stability, providing values also similar to those obtained when applying more demanding methods.4,9
The accuracy of the ESI-MS method (Table S3†) was also satisfactory and within the accepted limits of 70–120%.24 Also for all acids, repeatability and intermediate precision tests were within the acceptable values: 5 and 15%, respectively.23 The intermediate precision of the method was evaluated by comparing the ion peak areas obtained for each acid on different days by F test and no significant difference between days was found (p < 0.01) for all the three organic acids. The CV% (coefficient of variation) for intra-day precision ranged from 0.82 to 2.99% and for inter-day precision from 0.82 to 4.26%, indicating good precision.
3.2. Concentration of malic, tartaric and citric acids in wine
Table 1 shows the concentrations of malic, tartaric and citric acids found in wine samples using the direct infusion ESI-MS method. Overall in wine samples, higher content of tartaric acid was found compared to other acids, except in the RS/Brazilian (Merlot grape), RS/Brazilian (Isabel grape) and in the Chilean and Argentinian (both Syrah grapes) wines. This variation is possibly due to the structure of these grapes, especially the skins, where higher concentration of tartaric acid is found. Another aspect that may have contributed to the higher content of tartaric acid than malic acid in some wines is malolactic fermentation (MLF) responsible for the transformation of malic acid into lactic acid and carbon dioxide through the action of lactic acid bacteria. Other variables such as climatic conditions, grape maturation cycle, preparation process and storage conditions can also affect the acid content.
3.3. Validation of the procedure for extraction and analysis of organic acids in grapes
Table S3† shows the results of the linearity of the analytical curve, limit of detection and quantification accuracy for grapes, expressed as percentage of recovery. The extraction and dilution parameters were optimized and linear coefficients of the calibration curves were R2 = 0.992 for malic acid and R2 = 0.998 for tartaric acid. The accuracy was considered satisfactory (within the limits accepted by INMETRO24 of 70 to 120% recovery), as well as the other parameters CV%, recovery, linearity, LOD, and LOQ. The CV% values and percentage of recovery of the extracts fortified with standards at different concentrations showed good precision and accuracy, as evaluated by the recovery tests.
3.4. Concentration of malic, tartaric and citric acids in grapes
Quite opposite to the wine samples (Table 1), predominance of malic acid over tartaric acid was observed for all grape samples analyzed (Table 2). Grapes of American origin and hybrids (Bordô, Niagara, Carmen and Maximum BRS) have skins and pulp with higher levels of tartaric acid in comparison with Vitis vinifera cultivar Syrah. For seeds, the reverse was observed and the Vitis vinifera cultivar had a higher content of tartaric acid than the group of American and hybrid grapes. Lower malic acid content was found in the skin, pulp and seeds of Bordô grapes.
Table 2 Concentrations of malic and tartaric acids in national samples of grapes and hybrids grown in Santa Catarina, Paraná and São Pauloa
Sample |
Concentration of tartaric acid |
Concentration of malic acid |
Diluted sample μg mL−1 |
CV% |
μg per 100 mg lyophilized material |
Diluted sample μg mL−1 |
CV% |
μg per 100 mg lyophilized material |
Bordô* – Brazilian denomination for Vitis labrusca grapes cultivated in the south of Brazil.
|
Carmen skins |
4.66 |
1.14 |
18.65 |
9.82 |
0.77 |
39.30 |
Niagara skins |
4.41 |
2.61 |
17.63 |
8.41 |
2.86 |
33.65 |
Bordô* skins |
2.91 |
0.64 |
11.63 |
4.88 |
1.32 |
19.51 |
Syrah skins |
1.73 |
6.34 |
6.90 |
8.19 |
4.54 |
32.77 |
Maximum skins |
4.55 |
6.29 |
18.18 |
6.05 |
3.26 |
24.22 |
Bordô* pulp |
6.69 |
1.15 |
334.41 |
7.25 |
1.59 |
725.48 |
Carmen pulp |
2.83 |
1.21 |
141.36 |
8.13 |
12.83 |
813.15 |
Maximum pulp |
4.72 |
1.65 |
236.02 |
9.55 |
6.05 |
954.79 |
Niagara pulp |
6.04 |
2.36 |
301.85 |
8.04 |
6.15 |
803.96 |
Syrah pulp |
4.53 |
0.79 |
226.54 |
7.63 |
8.26 |
762.78 |
Bordô* seeds |
2.02 |
20.61 |
40.39 |
1.22 |
9.74 |
48.99 |
Niagara seeds |
4.05 |
13.13 |
81.07 |
4.60 |
11.78 |
184.15 |
Syrah seeds |
3.32 |
4.70 |
132.72 |
7.86 |
10.04 |
314.31 |
Carmen seeds |
0.94 |
3.42 |
37.55 |
5.51 |
6.56 |
220.25 |
Maximum seeds |
2.60 |
7.85 |
104.03 |
5.24 |
9.21 |
209.77 |
3.5. PCA of the ESI-MS fingerprints
Wine ESI(−)-MS fingerprints were found to be similar to those of grape skins and therefore they are placed closely by PCA. When samples of wine and grape skins are individually evaluated, they formed however two distinct groups (Fig. 1). The ESI(−)-MS fingerprints of samples of skins, pulp and seeds of grapes differ mostly in the abundance of common ions of m/z 115, 133, 149 and 179 (Fig. 2). These fingerprints of the skins, pulp and seeds also present other common ions of m/z 119, 179, 191, 341.113, 161, 195 and 321.
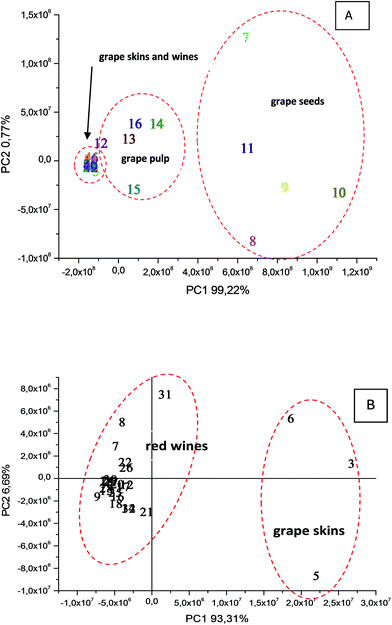 |
| Fig. 1 Principal component analysis (PC1 × PC2) applied to the ESI(−)-MS data: (A) samples of skin, pulp (12–16) and seeds (7–11) of grapes as well as wine samples; (B) samples of red wines (7–32) and skins from different varieties of grapes: Niagara (3), Syrah (5) and Maximum hybrid (6). | |
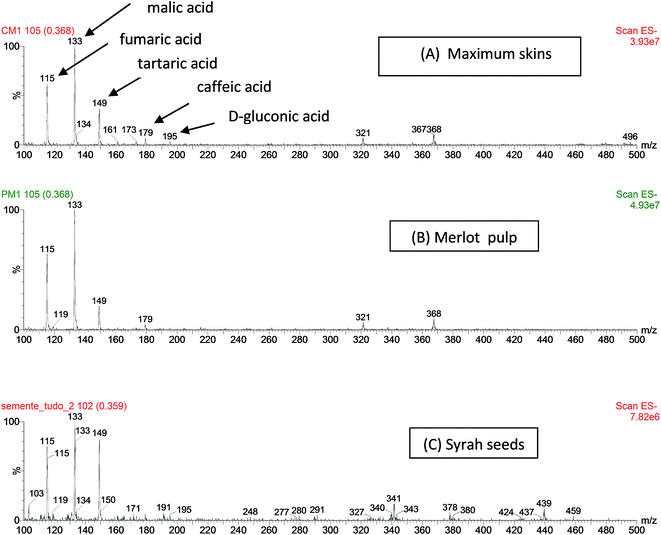 |
| Fig. 2 ESI(−)-MS fingerprints of samples of skins (A), pulp (B) and seeds (C) of different grapes. Major organic acids detected as [M − H]− ions are indicated in (A). | |
3.6. High resolution ESI(−)-MS fingerprints
High resolution ESI(−)-MS data of a wine sample permitted the separation of isobaric ions of m/z 191 from quinic acid and citric acid (Fig. 3 and Table 3). Although detected together in low resolution ESI(−)-MS as a single ion, both of these isobaric acids normally produce less abundant ions of m/z 191 in wines than the ion of m/z 193 from galacturonic acid. The m/z 191
:
193 ratio can be used therefore to monitor wine adulteration by the addition of citric acid. Fig. 4 shows a typical ESI(−)-MS fingerprint of wine with (A) a normal m/z 191
:
193 ratio as well as the same sample spiked with citric acid. Note that already at a concentration of 18 μg mL−1 (less than 20 ppm), this ratio is inverted. The presence of malic and tartaric acids in the wine sample was also confirmed by high resolution MS (Fig. 5). Note that no other ions of the same nominal m/z are present, therefore the low resolution ESI(−)-MS is capable of quantifying these acids without interference from isobaric compounds.
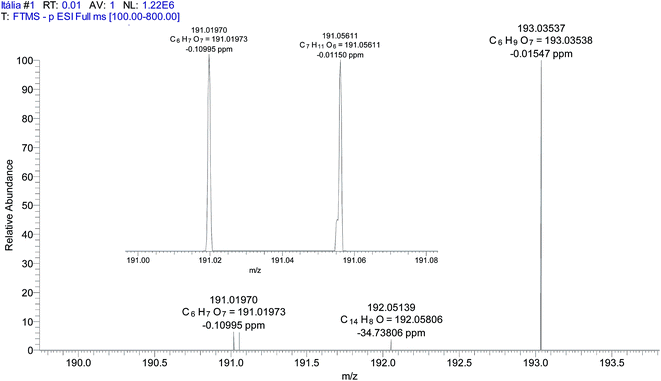 |
| Fig. 3 High resolution ESI FT-ICR MS in the m/z 190–194 range showing resolution and accurate m/z values for [M − H]− ions of citric (m/z 191.01989), quinic (m/z 191.05611) and galacturonic (m/z 193.03547) acids. | |
Table 3 Compounds identified in wine and grapes analyzed by ESI (−) FT-ICR MS
Acids |
Formula |
Theoretical m/z |
Experimental m/z |
Error (ppm) |
Malic |
C4H5O5 |
133.01430 |
133.01425 |
0.38552 |
Tartaric |
C4H5O6 |
149.00924 |
149.00916 |
−0.10995 |
Citric |
C6H7O7 |
191.01970 |
191.01973 |
−0.10995 |
Quinic |
C7H11O6 |
191.05611 |
191.05611 |
−0.01150 |
Galacturonic |
C6H9O7 |
193.03537 |
193.03538 |
−0.01547 |
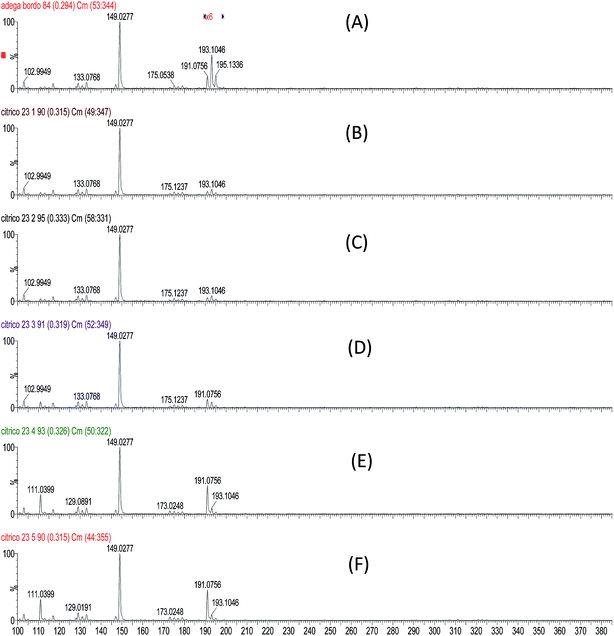 |
| Fig. 4 Direct infusion ESI(−)-MS fingerprints of: (A) a pure wine sample as well as the same sample adulterated with citric acid to the concentration of (B) 1.0 μg mL−1, (C) 3.0 μg mL−1, (D) 18 μg mL−1, (E) 70.0 μg mL−1 and (F) 100 μg mL−1. Relative abundances of the ions of m/z 149-tartaric acid, m/z 191-citric acid and m/z 193-galacturonic acid can be observed. | |
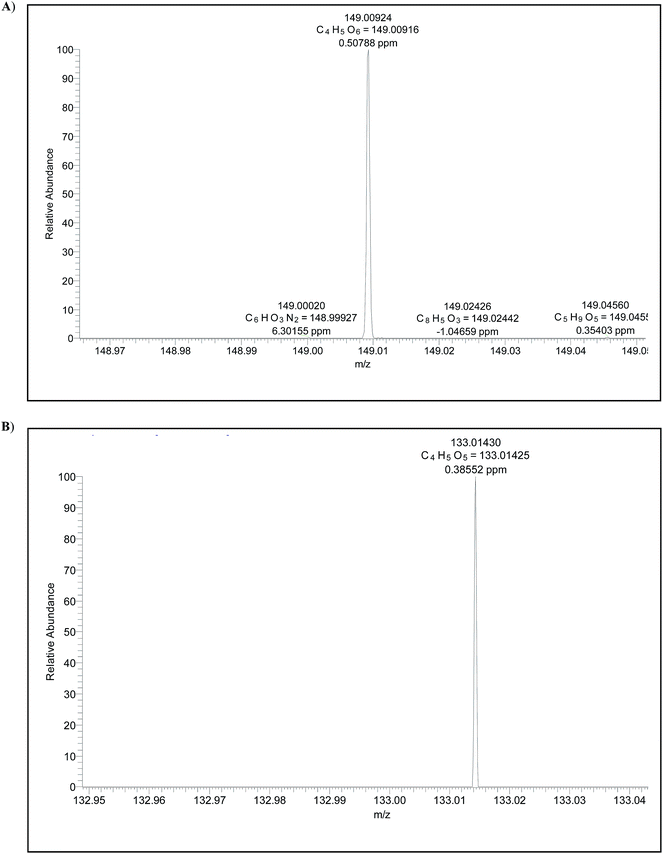 |
| Fig. 5 High resolution ESI(−) FT ICR MS data showing accurate m/z values for [M − H]− ions of: (A) tartaric acid and (B) malic acid found in wines and grapes. | |
4. Conclusions
Direct infusion ESI-MS is a method that provides quite comprehensive wine analysis. As shown herein, it can be successfully applied to quantitate organic acids in wine and grapes. The main advantages are its speed (less than 5 min per sample of total analysis time) and simplicity and the analysis of the intact sample with no pre-separation or pre-derivatization procedures. The linearity, precision, sensitivity and recuperation were as good as those of chromatographic methods using pre-separation via liquid chromatography4,10 or capillary electrophoresis.8,9 Additionally, direct infusion ESI-MS also enables wine typification as demonstrated herein via PCA analysis, which grouped samples according to similar concentrations of organic acids. Two groups were formed, the first group consisting of wines originating from American grapes (Merlot and Bordô) and the second one consisting of wines from national and hybrid grapes (Syrah, Maximum and Carmen). Tartaric acid was found to be predominant in most national and imported wines analyzed.
The method is shown to be also able to monitor wine adulteration by the addition of exogenous acids, and citric acid with an LOD of ca. 20 ppm could be detected. Direct Infusion ESI-MS was also shown to be applicable to monitor different stages of ripeness in grapes and to establish the ideal time of grape harvest as the concentrations of tartaric and malic acids can be quantified. All national varieties and hybrid grapes were shown to display similar chemical ESI-MS profiles but differ in abundances of ions from malic and tartaric acids, therefore making it possible to simultaneously evaluate, both qualitatively and quantitatively, the presence of these key analytes in different samples of grape skins, pulps and seeds.
Acknowledgements
We thank the following Brazilian research foundations CNPq, CAPES, FINEP and FAPESP for the financial support and Paulo Mazzafera (FAPESP 2008/58035-6) for the use of the UPLC-MS equipment.
References
- L. L. Andrade Lima, A. Schuler, N. B. Guerra, G. E. Pereira, T. L. Andrade Lima and H. Rocha, Otimização e validação de métodos para determinação de ácidos orgânicos em vinhos por cromatografia líquida de alta eficiência, Quim. Nova, 2010, 33(5), 1186–1189 CrossRef PubMed.
- I. Mato, S. Suárez-Luque and J. F. Huidobro, A review of the analytical methods to determine organic acids in grape juice and wines, Food Res. Int., 2005, 38, 1175–1188 CrossRef CAS PubMed.
- M. Palma and C. G. Barroso, Ultrasound-assisted extraction and determination of tartaric and malic acids from grapes and winemaking by products, Anal. Chim. Acta, 2002, 458, 119–130 CrossRef CAS.
- Y. J. Zheng, Y. T. Duan, Y. F. Zhang, Q. H. Pan, J. M. Li and W. D. Huang, Determination of Organic Acids in Red Wine and Must on Only One RP-LC-Column Directly After Sample Dilution and Filtration, Chromatographia, 2009, 69, 1 Search PubMed.
- A. Villiers, P. Alberts, A. G. J. Tredoux and H. H. Nieuwoudt, Analytical techniques for wine analysis: an African perspective; a review, Anal. Chim. Acta, 2012, 730, 2–23 CrossRef PubMed.
- S. Ehling and S. Cole, Analysis of organic acids in fruit juice by liquid chromatography-mass spectrometry: an enhanced tool for authenticity testing, J. Agric. Food Chem., 2011, 59, 2229–2234 CrossRef CAS PubMed.
- R. F. Frayne, Direct analysis of the major organic components in grape must and wine using high performance liquid chromatography, Am. J. Enol. Vitic., 1986, 37(4), 281–287 CAS.
- R. G. Peres, E. P. Moraes, G. A. Micke, F. G. Tonin, M. F. M. Tavares and D. B. Rodriguez-Amaya, Rapid method for the determination of organic acids in wine by capillary electrophoresis with indirect UV detection, Food Control, 2009, 20, 548–552 CrossRef CAS PubMed.
- I. Mato, S. Suárez-Luque and J. F. Huidobro, Simple determination of main organic acids in grape juice and wine by using capillary zone electrophoresis with direct UV detection, Food Chem., 2007, 102, 104–112 CrossRef CAS PubMed.
- A. Zotou, Z. Loukou and O. Karava, Method Development for the Determination of Seven Organic Acids in Wines by Reversed-Phase High Performance Liquid Chromatography, Chromatographia, 2004, 60, 39–44 CAS.
- S. M. Cottica, A. C. H. F. Sawaya, M. N. Eberlin, S. L. Franco, L. M. Zeoula and J. V. Visentainer, Antioxidant activity and composition of propolis obtained by different methods of extraction, J. Braz. Chem. Soc., 2011, 22(5), 929–935 CrossRef CAS PubMed.
- A. L. Schiozer, E. C. Cabral, L. A. F. Godoy, F. C. M. Chaves, R. J. Poppi, J. M. Riveros, M. N. Eberlin and L. E. S. Barata, Electrospray ionization mass spectrometry fingerprinting of extracts of the leaves of arrabidaea chica, J. Braz. Chem. Soc., 2012, 23(3), 409–414 CAS.
- A. C. H. F. Sawaya, R. R. Catharino, E. M. P. Facco, A. O. Fogaça, H. T. Godoy, C. E. Daudt and M. N. Eberlin, Monitoring of wine aging process by electrospray ionization mass spectrometry, Cienc. Tecnol. Aliment., 2011, 31(3), 730–734 CrossRef PubMed.
- H. J. Cooper and A. G. Marshall, Electrospray Ionization Fourier Transform Mass Spectrometric Analysis of Wine, J. Agric. Food Chem., 2001, 49, 5710–5718 CrossRef CAS PubMed.
- E. Villaga, L. S. Santos, B. G. Vaz, M. N. Eberlin and V. F. Laurie, Varietal discrimination of Chilean wines by direct injection mass spectrometry analysis combined with multivariate statistics, Food Chem., 2012, 131, 692–697 CrossRef PubMed.
- A. C. L. Amorin, A. M. C. Hovell, A. C. Pinto, M. N. Eberlin, N. P. Arruda, E. J. Pereira, H. R. Bizzo, R. R. Catharino, Z. B. Moraes Filho and C. M. Rezende, Green and roasted arabica coffees differentiated by ripeness, process and cup quality via electrospray ionization mass spectrometry fingerprinting, J. Braz. Chem. Soc., 2009, 20(2), 313–321 CrossRef PubMed.
- R. R. Catharino, H. M. S. Milagre, S. A. Saraiva, C. M. Garcia, U. Schuchardt and M. N. Eberlin, Biodiesel typification and quality control by direct infusion electrospray ionization mass spectrometry fingerprinting, Energy Fuels, 2007, 21, 3698–3701 CrossRef CAS.
- R. Roesler, R. R. Catharino, L. G. Malta, M. N. Eberlin and G. Pastore, Antioxidant activity of Caryocar brasiliense (pequi) and characterisation of components by electrospray ionization mass spectrometry, Food Chem., 2008, 110, 711–717 CrossRef CAS PubMed.
- P. Flores, P. Hellín and J. Fenoll, Determination of organic acids in fruits and vegetables by liquid chromatography with tandem-mass spectrometry, Food Chem., 2012, 132, 1049–1054 CrossRef CAS PubMed.
- J. K. S. Møller, R. R. Catharino and M. N. Eberlin, Electrospray ionization mass spectrometry fingerprinting of essential oils: spices from the labiatae family, Food Chem., 2007, 100, 1283 CrossRef PubMed.
- R. R. Catharino, I. B. S. Cunha, A. O. Fogaça, E. M. P. Facco, H. T. Godoy, C. E. Daudt, M. N. Eberlin and A. C. H. F. Sawaya, Characterization of must and wine of six varieties of grapes by direct infusion electrospray ionization mass spectrometry, J. Mass Spectrom., 2006, 41, 185–190 CrossRef CAS PubMed.
- E. S. Lago-Vanzela, R. Da-Silva, E. Gomes, E. Garcia-Romero and I. Hermosin-Gutiérrez, Phenolic Composition of the Edible Parts (Flesh and Skin) of Bordô Grape (Vitis labrusca) Using HPLC-DAD-ESI-MS/MS, J. Agric. Food Chem., 2011, 59(24), 13136–13146, DOI:10.1021/jf203679n.
- M. Ribani, C. B. G. Bottoli, C. H. Collins, I. C. S. F. Jardim and L. F. C. Melo, Validação em métodos cromatográficos e eletroforéticos, Quim. Nova, 2004, 27, 771–780 CrossRef CAS PubMed.
- Coordenação Geral de Acreditação, Orientação sobre Validação de métodos analíticos, DOQ-CGCRE-008-Revisão 03 – Fev/2010.
Footnote |
† Electronic supplementary information (ESI) available. See DOI: 10.1039/c4ay00114a |
|
This journal is © The Royal Society of Chemistry 2015 |
Click here to see how this site uses Cookies. View our privacy policy here.