DOI:
10.1039/C4AY01986E
(Paper)
Anal. Methods, 2015,
7, 301-308
A new potentiometric sensor for the determination of ketamine hydrochloride in ampoules and urine
Received
22nd August 2014
, Accepted 2nd November 2014
First published on 5th November 2014
Abstract
Ketamine drug in urine and pharmaceutical preparations was determined by a new chemically modified carbon paste electrode (CMCPE) based on an ion-exchanger of ketamine hydrochloride with sodium tetraphenylborate (KT-TPB) as a chemical modifier. The best performance was exhibited by the electrode having a paste containing 0.5 wt% ion-exchanger (KT-TPB), 54.3 wt% graphite, 45.0 wt% tris(2-ethylhexyl) phosphate (TEPh) and 0.2 wt% sodium tetraphenylborate (Na-TPB). The prepared electrode showed a Nernstian slope of 58.9 ± 0.3 mV per decade for ketamine ions in the concentration range of 9.0 × 10−6 M to 1.0 × 10−2 M with a limit of detection of 7.3 × 10−6 M. The electrode has a short and stable response time of 8 s and good reproducibility, and it can be used in a pH range of 3.7–6.6. The selective coefficients were determined in relation to several inorganic and organic ions, sugars and some common drug excipients. Ketamine is determined successfully in ampoule and urine using the standard addition and the calibration curve methods.
Significance, novelty and wider societal impact of the work reported
Ketamine is a colorless, odorless, tasteless, hallucinogenic and anesthetic drug commonly used in animals and humans. It is used by youth in recreational parties for sedation and misused in drug-facilitated crimes for its pharmacological properties. Thus, there is a critical need for the development of a selective, inexpensive diagnostic tool for the determination of this analyte. Potentiometric methods employing carbon paste electrodes (CPEs) have attracted attention as ion-selective electrodes mainly due to their advantages over membrane electrodes, such as chemical inertness, robustness, renewability, stable response, low ohmic resistance, no need for internal solution and suitability for a variety of sensing and detection applications. Moreover, CPEs are nontoxic and environmentally friendly electrodes. In their case, problems with passivation are simply eliminated by a simple and quick renewal of their surface. Due to the above mentioned properties, carbon paste electrodes seem to be especially promising. On careful review of literature, there is no report on the determination of ketamine hydrochloride using a carbon paste electrode. The present work describes the construction, potentiometric characterization, and analytical application of a new modified carbon paste electrode selective for ketamine based on ion-exchange of ketamine hydrochloride with sodium tetraphenylborate as an electroactive material and tris(2-ethylhexyl) phosphate as a plasticizer. The electrode exhibits a near Nernstian slope, wide concentration range, low detection limit and short response time. This electrode was used successfully for the determination of ketamine ions in ampoules and urine samples.
Introduction
Ketamine hydrochloride (KTCl), [2-(2-chlorophenyl)-2-(methylamino) cyclohexanone hydrochloride], a general anaesthetic, was first synthesized in 1962 as an alternative to its analogue, phencyclidine.1 KTCl is an odorless, tasteless and colorless drug, and it can be added to beverages without being noticed by the victim, promoting stupor and sedation together with amnesia. Because of its pharmacological properties, this drug is also misused by offenders in cases of drug-facilitated crimes (DFC).2,3 Therefore, the determination of ketamine has important practical implications.
Several analytical techniques have been used to evaluate ketamine in pharmaceutical products including a direct UV-visible spectrophotometry method,4 gas chromatography-mass spectrometry,5–7 high performance liquid chromatography with UV detection (HPLC-UV),8–10 HPLC-CD-UV,11 and liquid chromatography high resolution mass spectrometry (LC-HRMS).12 In spite of the high sensitivity of these methods, they are very expensive, involve the use of complex procedures with several sample manipulations, and require long analysis time. Besides, none of them are easy to automate. Thus, there is a critical need for the development of a selective, inexpensive diagnostic tool for the determination of this analyte. Analytical methods based on potentiometric detection with ion-selective electrodes (ISEs) can be considered good alternatives because they have the longest history and probably the largest number of applications.13 For example, polymeric membrane-based ion-selective electrodes (ISEs) have been described for different analytes.13–33 ISEs were found effective for the analysis of pharmaceutical formulations13,15,20,24,30,33 due to their attractive properties of simple design, ease of construction, reasonable selectivity, fast response time, applicability to colored and turbid solutions and possible interfacing with automated and computerized systems.33 Carbon paste electrodes (CPEs) are considered an important type of ion-selective electrodes and are notable for their ubiquitous properties that entail advantages over membrane electrodes such as chemical inertness, robustness, renewability, response stability, low ohmic resistance, no need for internal solution and suitability for a variety of sensing and detection applications.34–36 Moreover, CPEs are nontoxic and environmentally friendly electrodes. In their case, problems with passivation are simply eliminated by a simple and quick renewal of their surface. The operational mechanism of the carbon paste electrodes depends on the properties of the modifier materials used to import selectivity towards the target species.37 Modified carbon electrodes have been widely used as sensitive and selective sensors in various electroanalytical methods.38
Two reports on potentiometric determination of ketamine hydrochloride based on polymeric membrane electrodes were found.39,40 The present electrode, as a carbon paste electrode, has advantages over membrane electrodes as indicated above. However, its detection limit is slightly smaller than but very close to reported electrodes. Overall, it provides a better alternative over the existing electrodes.
A recent review of literature found no reports on the determination of ketamine hydrochloride using a carbon paste electrode. In this work, we describe the construction, performance characteristics and analytical application of a novel ketamine ion selective electrode based on an ion-exchanger of ketamine hydrochloride with sodium tetraphenylborate as an electroactive material and tris(2-ethylhexyl) phosphate as a plasticizer. The electrode exhibits a near Nernstian slope, wide concentration range, low detection limit and short response time. This electrode was used successfully for the determination of ketamine ions in samples of ampoules and urine.
Experimental
Reagents
Ketamine hydrochloride (KTCl) was provided by General Administration of Pharmacy, Ministry of Health (Gaza-Palestine). Graphite powder, dioctyl phthalate (DOP), dibutyl phthalate (DBP), tris(2-ethylhexyl) phosphate (TEPh) and dioctyl sebacate (DOS) as well as metal salts were purchased from Sigma-Aldrich (CH-9471 Buchs-Germany) and used as received. Silicotungstic acid (STA), silicomolybdic acid (SMA), phosphotungstic acid (PTA), phosphomolybdic acid (PMA) and sodium tetraphenyl borate (Na-TPB) were obtained from Sigma-Aldrich (CH-9471 Buchs-Germany). Glucose, galactose, fructose, sucrose, ceftriaxone sodium, ampicillin sodium, gentamycine sulphate, hydrocortisone sodium, lasix and diclofine sodium were obtained from local drug stores (Gaza-Palestine).
Apparatus
Potentiometric and pH measurements were made with a Pocket pH/mV Meter, pH315i (Wissenschaftlich-Technische Werkstatten GmbH (WTW), Weilheim, Germany). A saturated calomel electrode (SCE) was used as the reference electrode for potential measurements and was obtained from Sigma-Aldrich (St Louis, MO, USA). The emf measurements made with the CMCPE were carried out with the following cell notations: Hg, Hg2Cl2 (s), KCl (sat.)‖sample solution|CMCPE.
Preparation of ion-exchanger complex
The ion-exchangers, ketaminium silicotungstate (KT4-ST), ketaminium silicomolybdate (KT4-SM), ketaminium phosphotungstate (KT3-PT), ketaminium phosphomolybdate (KT3-PM) and ketaminium tetraphenylborate (KT-TPB) were prepared according to a previously reported method,15,27 by adding a hot solution of 50 mL of 0.01 M KTCl to 12.5 mL of 0.01 M of silicotungstic acid (STA) or silicomolybdic acid (SMA), 16.66 mL of 0.01 M phosphomolybdic acid (PMA) or phosphotungstic acid (PTA) and 50 mL of 0.01 M of sodium tetraphenylborate. The precipitates that formed were filtered off, washed thoroughly with distilled water, dried at room temperature and ground to fine powders. The ion-exchanger complex was used as the active substance for preparing the CMCPEs of ketamine hydrochloride.
Preparation of the modified and unmodified electrodes
Modified and unmodified carbon paste electrodes were made according to a general procedure, as described elsewhere.30,36 High purity graphite, ion-exchanger and different types of plasticizers were intimately hand mixed in a Petri dish to obtain a very fine paste. A portion of the composite mixture was packed firmly into the end of a disposable polypropylene syringe (ca. 3 mm i.d. and 6 cm long) where electrical contact was established with a copper screw wire. To obtain a stable electrochemical response, the outer layer of the carbon paste was renewed before each set of measurements by polishing the surface of the electrode. The sensor became ready for use in potentiometric measurements.
Selectivity coefficient determination
The separate solution method (SSM) and the matched potential method (MPM)41 were employed to determine the selectivity coefficients of the potentiometric sensor towards different species. The following equation was employed in SSM.
where E1 is the potential for the surfactant ion and E2 is that for the interfering ion J, with charge z and slope S of the calibration graph.
According to the MPM, the activity of (KT) was increased from aKT = 1.0 × 10−5 M (reference solution) to a∼KT = 5.0 × 10−5 M, and the changes in potential (ΔE) corresponding to this increase were measured. Next, a solution of an interfering ion of concentration aJ in the range 1.0 × 10−1 M to 1.0 × 10−2 M was added to a new 5.0 × 10−5 M reference solution until the same potential change (ΔE) was attained. The selectivity factor for each interferent was calculated using the following equation:
where
aJ is the activity of the added interferent.
Sample preparation
Samples of ketamine hydrochloride ranging from 9.0 × 10−6 M to 1.0 × 10−2 M KTCl were determined by the standard addition, potentiometric titration and the calibration curve methods. The required amount of the stock solution was transferred to a 50 mL volumetric flask and diluted to the mark with distilled water to make 0.01 M solutions of KTCl. Different volumes of these solutions equivalent to 1.0 × 10−5 M to 1.0 × 10−2 M were analyzed by the above methods using the present electrode. Each analysis was repeated five times. Ampoules containing 10.0 and 20.0 mg mL−1 of KTCl and urine analytes were measured as real samples that contained 2.7 × 10−2 M and 5.4 × 10−2 M of KTCl, respectively. Dilute solutions that had 1 × 10−4 M and 1 × 10−5 M KTCl in each solution were made by transferring the required amounts to 25 mL volumetric flasks and applying proper dilutions. These solutions were subjected to the standard addition method and the calibration curve method.
Sample analysis
The standard addition method, in which small increments (10–100 μL) of (0.1 M) KT solution were added to 25 mL aliquot samples at two concentrations (5.0 × 10−5 M and 5.0 × 10−4 M) of KTCl was applied. The change in potential at 25 ± 0.1 °C was recorded after each increment, and these data were used to calculate the concentration of KT ions in the solution samples. The potentiometric titration of different volumes of 1.0 × 10−3 M and 1.0 × 10−2 M KTCl solution was performed as follows: 5–10 mL equivalent to 1.87–37.4 mg, were transferred to a 25 mL beaker and titrated with a standard solution of Na-TPB using the prepared KT-TPB as an indicator electrode. The end points were determined from the S-shaped curve. In the calibration graph method, different amounts of KT were added to 50.0 mL of water to comprise a concentration range from 1.0 × 10−5 M to 1.0 × 10−2 M, and the measured potential was recorded using the present electrode. Data were plotted as potential versus logarithm of the KT+ activity, and the resulting graph was used for subsequent determination of the concentration of drug sample.
Results and discussion
Composition of the electrode
It is well known that the performance characteristics of a given CMCPE based on ion-exchangers depend to a large extent on the nature and amount of the ion-exchanger complex and their lipophilicities,27 the properties of the plasticizer,42 any additives used43 and the graphite (g)/plasticizer (p) ratio.44 Thus, the influences of paste composition, nature and amount of plasticizer and amount of additives such as sodium tetraphenylborate on the potential response of the proposed sensor were tested, and the obtained results are given in Table 1.
Table 1 Composition and slope of calibration curve for KT-CMCPE at 25.0 ± 0.1a
|
Ion exchanger |
Composition (%) |
A
|
Slope (mV per decade) |
Linear range (M) |
Detection limit (M) |
R. S. D % |
Response time (s) |
g/p |
I |
g |
p |
I: ion-exchanger, g: graphite, p: plasticizer, R. S. D: relative standard deviation, * selected composition, A: additive Na-TPB.
|
1 |
— |
— |
— |
54.5 |
45.5 |
TEPh |
— |
24.7 ± 0.3 |
9.0 × 10−5 to 1.0 × 10−2 |
7.8 × 10−5 |
1.10 |
18 |
2 |
KT-TPB |
1.20 |
0.1 |
54.5 |
45.2 |
TEPh |
0.20 |
35.8 ± 0.6 |
7.2 × 10−5 to 1.0 × 10−2 |
5.2 × 10−5 |
1.12 |
9 |
3 |
KT-TPB |
1.20 |
0.3 |
54.4 |
45.1 |
TEPh |
0.20 |
38.2 ± 0.5 |
5.3 × 10−5 to 1.0 × 10−2 |
2.7 × 10−5 |
1.15 |
12 |
4 |
KT-TPB* |
1.20 |
0.5 |
54.3 |
45.0 |
TEPh |
0.20 |
58.9 ± 0.3 |
9.0 × 10−6 to 1.0 × 10−2 |
7.3 × 10−6 |
0.76 |
8 |
5 |
KT-TPB |
1.20 |
0.5 |
54.3 |
45.2 |
TEPh |
— |
52.3 ± 0.7 |
6.4 × 10−5 to 1.0 × 10−2 |
2.1 × 10−5 |
0.84 |
15 |
6 |
KT-TPB |
1.20 |
1.0 |
54.0 |
44.8 |
TEPh |
0.20 |
45.2 ± 0.5 |
1.9 × 10−5 to 1.0 × 10−2 |
1.0 × 10−5 |
1.12 |
13 |
7 |
KT-TPB |
1.20 |
1.5 |
53.7 |
44.6 |
TEPh |
0.20 |
50.3 ± 1.3 |
3.6 × 10−5 to 1.0 × 10−2 |
1.4 × 10−5 |
0.77 |
9 |
8 |
KT-TPB |
1.20 |
2.0 |
53.5 |
44.3 |
TEPh |
0.20 |
41.8 ± 0.9 |
4.7 × 10−5 to 1.0 × 10−2 |
2.3 × 10−5 |
0.57 |
9 |
9 |
KT-TPB |
1.20 |
3.0 |
52.8 |
44.0 |
TEPh |
0.20 |
39.5 ± 1.1 |
7.4 × 10−5 to 1.0 × 10−2 |
4.0 × 10−5 |
0.77 |
10 |
10 |
KT-TPB |
1.20 |
4.0 |
52.3 |
43.5 |
TEPh |
0.20 |
34.3 ± 0.5 |
9.6 × 10−5 to 1.0 × 10−2 |
7.5 × 10−5 |
0.91 |
8 |
|
Effect of different plasticizer
|
11 |
KT-TPB |
1.20 |
0.5 |
54.3 |
45.0 |
DPB |
0.20 |
40.7 ± 0.8 |
8.0 × 10−5 to 1.0 × 10−2 |
3.0 × 10−5 |
1.10 |
12 |
12 |
KT-TPB |
1.20 |
0.5 |
54.3 |
45.0 |
DOP |
0.20 |
25.8 ± 0.6 |
2.5 × 10−4 to 1.0 × 10−2 |
5.0 × 10−5 |
1.10 |
10 |
13 |
KT-TPB |
1.20 |
0.5 |
54.3 |
45.0 |
DOS |
0.20 |
28.2 ± 0.7 |
1.0 × 10−4 to to 1.0 × 10−2 |
4.0 × 10−5 |
1.05 |
11 |
|
Effect of different ion pair
|
14 |
KT-SM |
1.20 |
0.5 |
54.3 |
45.0 |
TEPh |
0.20 |
26.3 ± 0.6 |
6.0 × 10−5 to 1.0 × 10−3 |
2.3 × 10−5 |
0.92 |
10 |
15 |
KT-ST |
1.20 |
0.5 |
54.3 |
45.0 |
TEPh |
0.20 |
22.4 ± 0.5 |
4.7 × 10−5 to 1.0 × 10−2 |
1.5 × 10−5 |
1.05 |
12 |
16 |
KT-PM |
1.20 |
0.5 |
54.3 |
45.0 |
TEPh |
0.20 |
25.8 ± 0.7 |
2.5 × 10−4 to 1.0 × 10−2 |
9.2 × 10−5 |
0.84 |
9 |
17 |
KT-PT |
1.20 |
0.5 |
54.3 |
45.0 |
TEPh |
0.20 |
30.2 ± 0.8 |
3.5 × 10−5 to 1.0 × 10−2 |
1.0 × 10−5 |
0.72 |
11 |
18 |
KT-TPB* |
1.20 |
0.5 |
54.3 |
45.0 |
TEPh |
0.20 |
47.8 ± 0.4 |
2.0 × 10−5 to 1.0 × 10−2 |
9.0 × 10−6 |
0.57 |
8 |
|
Different of g/p ratios
|
19 |
KT-TPB |
0.75 |
0.5 |
42.6 |
56.7 |
TEPh |
0.20 |
42.1 ± 0.7 |
2.4 × 10−5 to 1.0 × 10−2 |
1.0 × 10−5 |
1.14 |
11 |
20 |
|
0.90 |
0.5 |
47.2 |
52.3 |
TEPh |
0.20 |
43.9 ± 0.2 |
2.6 × 10−5 to 1.0 × 10−2 |
9.2 × 10−6 |
0.53 |
9 |
21 |
|
1.05 |
0.5 |
51.0 |
48.3 |
TEPh |
0.20 |
45.3 ± 0.5 |
3.0 × 10−5 to 1.0 × 10−2 |
9.0 × 10−6 |
0.51 |
10 |
22 |
|
1.20 |
0.5 |
54.3 |
45.0 |
TEPh |
0.20 |
58.9 ± 0.3 |
9.0 × 10−6 to 1.0 × 10−2 |
7.0 × 10−6 |
0.47 |
8 |
23 |
|
1.35 |
0.5 |
57.1 |
42.2 |
TEPh |
0.20 |
42.0 ± 0.7 |
4.5 × 10−5 to 1.0 × 10−2 |
1.7 × 10−5 |
0.68 |
14 |
Effect of ion-exchanger
The ion-exchanger complex used in ISEs should have rapid exchange kinetics and adequate formation constants in the paste. In addition, they should have good solubility in the paste matrix and sufficient lipophilicity to prevent leaching from the paste into the sample solution.45 The ion-exchangers KT-ST, KT-SM, KT-PT, KT-PM and KT-TPB were prepared and tested as modifiers for the present electrode. The influence of the amount of the different ketamine ion-exchangers in the carbon paste was investigated, and the corresponding results are summarized in Table 1. It is noted that the electrode containing a zero percentage of ion-exchanger complex (sensor no. 1) showed a negligible response towards ketamine cations, whereas in the presence of the ion-exchanger complexes, the sensor displayed remarkable selectivity for ketamine cations. The electrode # 4 made of 0.5% (w/w) modifier exhibits the best performance. However, further addition of the modifier, (sensors no. 6 to 10), display somewhat smaller slopes and sensitivity, most probably due to some heterogeneities and possible saturation of the paste.46
Effect of plasticizers
The plasticizer, in particular, has a dual function: it acts as a liquifying agent, making the membrane material workable, that is enabling homogenous solubilization and modifying the distribution constant of the ion-exchanger used and sustaining these characteristics on continued use. The proportion of plasticizer must be optimized in order to minimize the electrical asymmetry of the membrane so as to keep the sensor as clean as possible and to stop leaching to the aqueous phase.47,48 In addition, the plasticizer influences the mobility of the ion-exchanger through extraction of both ions into the organic phase.49 Therefore, it is necessary to use plasticizers with different physical parameters, such as dielectric constant (ε), lipophilicity (log
PTLC) and molecular weight (Mw). In order to explore a suitable plasticizer for constructing this electrode, we used four plasticizers, with the values of dielectric constants (which is a measure of the molecular polarity), lipophilicity and molecular weight respectively listed in parentheses:44 DOS (εr = 3.9, log
PTLC = 10.1, Mw = 427), DOP (εr = 5.1, log
PTLC = 7.0, Mw = 391), DBP (εr = 6.4, log
PTLC = 4.5, Mw = 278) and DOPh (εr = 4.8, log
PTLC = 10.2, Mw = 434). The CPE with TEPh as a solvent mediator (plasticizer) produced the best response, as shown in Table 1 and Fig. 1. This is likely due to the relatively high molecular weight, low dielectric constant and high lipophilicity that may avoid exudation and considerably affect dissolution of ion associations within the paste. This effect is due to increasing its partition coefficient and providing suitable mechanical properties for comparison with low polarity plasticizers.50
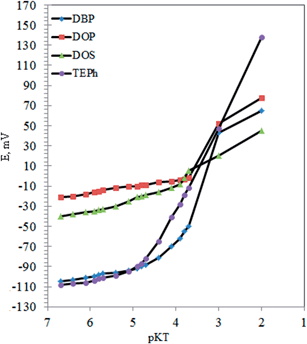 |
| Fig. 1 Variation of electrode potentials with different plasticizers. | |
The influence of anionic additive
The presence of lipophilic anion sites in cation-selective electrode reduces ohmic resistance and improves response behavior and selectivity. In addition, it enhances the selectivity of the paste electrode in cases where the extraction capability of the ion-exchanger is poor. Moreover, the lipophilic additive may catalyze the exchange kinetics at the sample-electrode interface.48,51 Comparison of the data (electrodes #4 and 5) revealed that the sensitivity of the sensor increased and the slope of the calibration curve also increased from 52.3 ± 0.7 to 58.9 ± 0.3 mV per decade with the addition of a trace of Na-TPB (about 0.20 wt%).
Effect of graphite/plasticizer (g/p) ratio
Different graphite/plasticizer ratios 0.75, 0.90, 1.05, 1.20 and 1.35 using TEPh as the plasticizer were tested while keeping the amount of ion-exchanger complex constant (i.e. 0.5%) as shown in Table 1. The paste with a (g/p) ratio of 1.20 showed the optimum physical properties, ensured high enough mobilities of their constituents51 and was found to give the best reproducibility and sensitivity of 58.9 mV per decade over the widest linear concentration range of 9.0 × 10−6 M to 1.0 × 10−2 M in comparison with the other ratios tested. Pastes with g/p more than 1.35 produced “crumbly” pastes, and those with a ratio smaller than 0.75 had a consistency resembling that of “peanut butter”, i.e., not workable.
Among the electrodes tested, the electrode containing 45.0% TEPh, 54.3% graphite, 0.5% ion-exchanger (KT-TPB), and 0.20% of sodium tetraphenylborate (Na-TPB) as the additive exhibited the best response characteristics and the lowest detection limit. Therefore, this composition was used to study various operation parameters of the electrode. The electrochemical performance characteristics of these electrodes were systematically evaluated according to the International Union of Pure and Applied Chemistry (IUPAC) recommendations.52 The performance of the electrode was investigated by measuring the emfs of KTCl solutions within a concentration range of 10−7 M to 10−2 M by serial dilution. Each solution was stirred, and the potential reading was recorded when it became stable and plotted as a logarithmic function of KT cation activities as shown in Fig. 2.
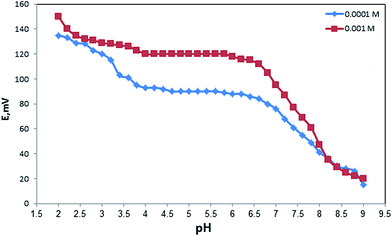 |
| Fig. 2 Influence of pH on the response of the present electrode at 1.0 × 10−4 M and 1.0 × 10−3 M. | |
Effect of pH
The most important factor in the functioning of most ion-selective electrodes is the medium acidity expressed as pH value. The effect of pH on the electrode potential at 25 °C of the KTCl solutions was studied in 1.0 × 10−3 M and 1.0 × 10−4 M over the pH range of 2.0–9.0. The acidity was adjusted by adding small volumes of 1.0 M HCl or NaOH to the test solutions and the variation in potential was followed. As can be seen in Fig. 2, the potential response remains almost constant over the pH range 3.7–6.6, which can be taken as the working pH range for the electrode. However, there is a slight deviation at pH values lower than 3.7, which may be due to H+ interference. On the other hand, the potential decreases gradually at pH values higher than 6.6. The decrease may be attributed to hydroxide ions that react with ketamine, leading to the formation of free drug in the test solution and neutral species, which could not be extracted into the membrane.
Response time, electrode renewal and repeatability of the electrode
For analytical applications, the dynamic response time is a significant parameter for any sensor. The response time of the electrode is defined as the time between addition of the analyte to the sample solution and the time when a limiting potential has been reached.52 In this work, the response time of the electrode was measured by varying the ketamine concentration over a range 1.0 × 10−5 M to 1.0 × 10−2 M. The electrode reaches equilibrium in about ∼8 s. As shown in Fig. 3, to check the effectiveness of surface-renewal, the calibration curve was constructed on a certain surface. The slope was found to decrease slightly from 58.9 ± 0.3 to 56.8 ± 0.7 mV per decade after five times of use. This decrease may be attributed to surface contamination and a memory effect. Therefore, the electrode surface should be polished to expose a fresh layer for use. The sensor repeatability was evaluated on the same surface by three successive measurements and resulted in standard deviations of 0.814 and 1.654 for 1.0 × 10−3 M and 1.0 × 10−4 M of ketamine, respectively. This indicates that the repeatability of the proposed electrode is satisfactory.
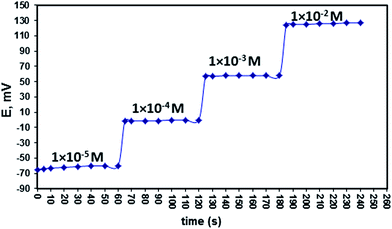 |
| Fig. 3 Typical potential–time plot for response of KT-CMCPE. | |
Thermal stability of KT-CPE
The response at different temperatures is an important factor in the characterization of a new sensor.53 By studying the temperature effect on the sensor, it is possible to determine the temperature range in which the sensor can be used. The thermal stability of the senor and the calibration graphs (electrode potential, vs. −log[KTCl, M]) were constructed at different test solution temperatures covering a range of 25 °C to 55 °C. The characteristics of the electrode, namely, the slope, LOD and usable concentration range at different test solution temperatures, were measured. The results indicate that no appreciable change in the calibration characteristics of the electrodes was observed in the temperature range 25 °C–55 °C, as shown in Fig. 4.
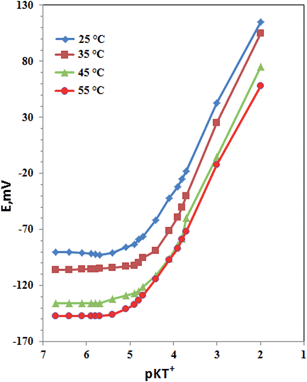 |
| Fig. 4 Calibration graphs for KT-CMCPE at test solution temperatures of 25, 35, 45 and 55 °C. | |
Effect of diverse ions
The selectivity coefficient is a summary of informations concerning interferences in the electrode response in analytical applications. The response for the analyte must be as high as possible compared to the response for foreign substances, which must be very small so that the electrode exhibits Nernstian dependence on the concentration of the primary ion over a wide range. The selectivity of the ion-exchanger of the electrode depends on the selectivity of the ion-exchange process at the sensor-test solution interface and the mobilities of the respective ions in the matrix of the sensor. Therefore, the response of the electrode towards different substances and ionic species such as inorganic and organic cations, should be measured. In addition, drug formulations may contain flavouring agents, diluents and excipients, such as maltose, glucose and lactose. The results listed in Table 2 reveal that there are no significant interferences from any of the tested substances due to the differences in their mobilities and permeabilities as compared with KT(I).54
Table 2 Selectivity coefficients of various interfering ions for the proposed electrode
Interfering ions |
MPM |
SSM |
Na+ |
1.45 × 10−4 |
4.64 × 10−5 |
K+ |
2.80 × 10−4 |
4.38 × 10−4 |
Ag+ |
1.88 × 10−4 |
3.95 × 10−4 |
Li+ |
5.47 × 10−4 |
7.61 × 10−5 |
Cd++ |
1.49 × 10−5 |
8.62 × 10−4 |
Ca++ |
4.24 × 10−4 |
2.18 × 10−4 |
Ni++ |
5.28 × 10−4 |
3.80 × 10−4 |
Co++ |
2.01 × 10−4 |
2.94 × 10−4 |
Cu++ |
1.65 × 10−5 |
4.16 × 10−4 |
Pb++ |
5.45 × 10−4 |
2.63 × 10−4 |
Zn++ |
4.21 × 10−4 |
2.15 × 10−4 |
Cr+++ |
4.04 × 10−4 |
2.00 × 10−4 |
Ampicilline |
1.23 × 10−4 |
5.45 × 10−4 |
Rocephen |
1.87 × 10−3 |
1.12 × 10−4 |
Gentamycine |
1.29 × 10−4 |
2.95 × 10−3 |
Lasix |
5.21 × 10−4 |
1.42 × 10−3 |
Hydrocortisone |
3.68 × 10−4 |
4.78 × 10−4 |
Diclofene |
3.10 × 10−4 |
5.24 × 10−4 |
Glucose |
4.86 × 10−5 |
— |
Galactose |
6.24 × 10−4 |
— |
Fructose |
1.68 × 10−5 |
— |
Sucrose |
3.75 × 10−4 |
— |
Applications
Titration of KTCl solution with Na-TPB.
The KT-CMCPE was successfully used as an indicator electrode in the potentiometric titration of 10.0 mL of 1.0 × 10−3 M KTCl with a 1.0 × 10−3 M Na–TPB of solution. As is obvious from Fig. 5, the amount of ketamine can be accurately determined from the end point of the titration curve.
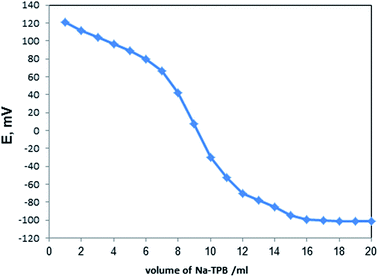 |
| Fig. 5 Potentiometric titration curve of 10.0 mL 1.0 × 10−3 M solution KTCl with 1.0 × 10−3 M solution Na-TPB using the present electrode. | |
Determination of KTCl in urine and pharmaceutical formulations.
Ketamine hydrochloride was measured in biological fluids (urine) and pharmaceutical preparations (ampoules). Each sample was analyzed in triplicate, using this sensor by the standard addition and the calibration methods. It is noted, from Table 3, that the results were accurate and reproducible, and the recovery of KTCl is almost quantitative.
Table 3 Analysis of KTCl in various samples using standard addition and calibration curve methodsa
Samples |
M |
X % |
R. S. D. % |
F-value |
t-value |
Taken |
Found |
C: calibration curve, S: standard addition method, R. S. D.: relative standard deviation, X: recovery. The critical value of F = 6.39 and the critical value of t = 3.707.
|
Ampoule
|
C |
1.00 × 10−5 |
9.95 × 10−6 |
99.50 |
1.06 |
2.36 |
3.01 |
2.00 × 10−4 |
1.95 × 10−4 |
97.50 |
1.25 |
2.54 |
0.29 |
S |
1.00 × 10−5 |
9.88 × 10−6 |
98.80 |
2.10 |
3.21 |
3.14 |
5.00 × 10−4 |
4.92 × 10−4 |
98.40 |
0.21 |
1.99 |
1.75 |
|
Urine
|
C |
1.00 × 10−5 |
9.85 × 10−6 |
98.50 |
1.08 |
2.35 |
0.71 |
2.00 × 10−4 |
1.93 × 10−4 |
98.75 |
0.24 |
2.19 |
1.86 |
S |
1.00 × 10−5 |
1.02 × 10−5 |
102.0 |
0.58 |
1.54 |
2.36 |
5.00 × 10−4 |
4.87 × 10−4 |
97.40 |
0.42 |
1.43 |
2.35 |
The results of applying the above methods are compared with the values obtained from the official method.55 The F-test was used for comparing the precision of the two methods and t-test for comparing the accuracy. The calculated F- and t-test in Table 3 were less than critical (tabulated) ones. Thus, there is no significant difference between the precisions or the accuracies of the two methods with 95% confidence levels.
Conclusions
The proposed chemically modified carbon paste electrode based on a modifier namely ketaminium tetraphenylborate (KT-TPB) as an electroactive ion exchanger complex might be a useful analytical tool and an interesting alternative for the determination of KT ions in pharmaceutical preparations and urine samples. The electrode shows high sensitivity, reasonable selectivity, fast static response, wide pH range, concentration range 9.0 × 10−6 M to 1.0 × 10−2 M, and low detection limits of 7.3 × 10−6 M with minimal sample pretreatment.
References
- K. Hirota and D. G. Lambert, Br. J. Anaesth., 1996, 77, 441–444 CrossRef CAS PubMed.
- A. V. de Bairros, R. Lanaro, R. M. de Almeida and M. Yonamine, Forensic Sci. Int., 2014, 243, 47–54 CrossRef PubMed.
-
United Nations Office on Drugs and Crime (UNODC), Guidelines for the Forensic Analysis of Drug Facilitating Sexual Assault and Other Criminal Acts 2011, United Nations Office on Drugs and Crime (UNODC), United Nations, New York, 2011, http://www.unodc.org/documents/scientific/forensic_analys_of_drugs_facilitating_sexual_assault_and_other_criminal_acts.pdfi, accessed 15 may 2013 Search PubMed.
- R. El-Sheikh, M. Zaky, F. Z. Mohamed and A. S. Amin, J. Chin. Chem. Soc., 2006, 53, 831–838 CAS.
- N. Feng, F. X. Vollenweider, E. I. Minder, K. Rentsch, T. Grampp and D. J. Vonderschmitt, Ther. Drug Monit., 1995, 17, 95–100 CrossRef CAS PubMed.
- Y. Chen, Y. Yang and Y. Tu, Sens. Actuators, B, 2013, 183, 150–156 CrossRef CAS PubMed.
- K. Lian, P. Zhang, L. Niu, S. Bi, S. Liu, L. Jiang and W. Kang, J. Chromatogr. A, 2012, 1264, 104–109 CrossRef CAS PubMed.
- J. O. Svensson and L. L. Gustafsson, J. Chromatogr. B: Biomed. Sci. Appl., 1996, 678, 373–376 CrossRef CAS.
- S. Bolze and R. Boulieu, Clin. Chem., 1998, 44, 560–564 CAS.
- P. Li, H. Han, X. Zhai, W. He, L. Sun and J. Hou, J. Chromatogr. Sci., 2012, 50, 108–113 CAS.
- A. Gergely, F. Zsila, P. Horváth and G. Szász, Chirality, 1999, 11, 741–744 CrossRef CAS.
- D. Favretto, S. Vogliardi, G. Stocchero, A. Nalesso, M. Tucci, C. Terranova and S. D. Ferrara, Forensic Sci. Int., 2013, 226, 88–93 CrossRef CAS PubMed.
- E. M. Hussien, F. M. Abdel-Gawad and Y. M. Issa, Biochem. Eng. J., 2011, 53, 210–215 CrossRef CAS PubMed.
- V. K. Gupta, A. K. Jain, G. Maheshwari, H. Lang and Z. Ishtaiwi, Sens. Actuators, B, 2006, 117, 99–106 CrossRef CAS PubMed.
- H. M. Abu Shawish, S. M. Saadeh, A. R. Al-Dalou, N. Abu Ghalwa and A. A. Abou Assi, Mater. Sci. Eng., C, 2011, 31, 300–306 CrossRef CAS PubMed.
- V. K. Gupta, A. K. Jain and P. Kumar, Sens. Actuators, B, 2006, 120, 259–265 CrossRef CAS PubMed.
- V. K. Gupta, A. K. Singh, M. Al Khayat and B. Gupta, Anal. Chim. Acta, 2007, 290, 81–90 CrossRef PubMed.
- A. Malon, A. Radu, W. Qin, Y. Qin, A. Ceresa, M. Maj-Zurawska, E. Bakker and E. Pretsch, Anal. Chem., 2003, 75, 3865 CrossRef CAS.
- V. K. Gupta, S. Chandra and H. Lang, Talanta, 2005, 66, 75–580 CrossRef PubMed.
- H. Ibrahim, Y. M. Issa and H. M. Abu-Shawish, Anal. Chim. Acta, 2005, 532, 79 CrossRef CAS PubMed.
- V. K. Gupta, R. Prasad and A. Kumar, Talanta, 2003, 60, 149–160 CrossRef CAS.
- V. K. Gupta, A. K. Singh, S. Mehtab and B. Gupta, Anal. Chim. Acta, 2006, 566, 5–10 CrossRef CAS PubMed.
- J. Lenik, Mater. Sci. Eng., C, 2013, 33, 311–316 CrossRef CAS PubMed.
- H. Ibrahim, Y. M. Issa and H. M. Abu-Shawish, J. Pharm. Biomed. Anal., 2005, 36, 1053 CrossRef CAS PubMed.
- V. K. Gupta, R. Prasad, P. Kumar and R. Mangla, Anal. Chim. Acta, 2000, 420, 19–27 CrossRef CAS.
- A. K. Jain, V. K. Gupta, L. P. Singh and U. Khurana, Analyst, 1997, 122, 583–586 RSC.
- H. M. Abu Shawish, A. M. Khedr, K. I. Abed-Almonem and M. Gaber, Talanta, 2012, 101, 211–219 CrossRef CAS PubMed.
- V. K. Gupta, S. Chandra and R. Mangla, Electrochim. Acta, 2002, 47, 1579–1586 CrossRef CAS.
- V. K. Gupta, R. Mangla, U. Khurana and P. Kumar, Electroanalysis, 1999, 11, 573–576 CrossRef CAS.
- H. M. Abu-Shawish, Electroanalysis, 2008, 20, 491–497 CrossRef CAS.
- V. K. Gupta, A. K. Jain, P. Kumar, S. Agarwal and G. Maheshwari, Sens. Actuators, B, 2006, 113, 182–186 CrossRef CAS PubMed.
- S. K. Srivastava, V. K. Gupta and S. Jain, Anal. Chem., 1996, 68, 1272–1275 CrossRef CAS PubMed.
- A. O. Santini, H. R. Pezza, J. E. de Oliveira and L. Pezza, J. Braz. Chem. Soc., 2008, 19, 162–168 CrossRef CAS PubMed.
- A. Soleymanpour and M. Ghasemian, Measurement, 2015, 59, 14–20 CrossRef PubMed.
- H. M. Abu-Shawish, A. A. Dalou, N. Abu Ghalwa, G. I. Khraish, J. Hammad and A. H. Basheer, Drug Test. Anal., 2013, 5, 213–221 CrossRef CAS PubMed.
- H. M. Abu Shawish, N. Abu Ghalwa, S. M. Saadeh and H. El Harazeen, Food Chem., 2013, 138, 126–132 CrossRef CAS PubMed.
- K. Kalcher, J. M. Kauffmann, J. Wang, I. Svancara, K. Vytras, C. Neuhold and Z. Yang, Electroanalysis, 1995, 7, 5–22 CrossRef CAS.
- R. F. Aglan, G. G. Mohamed and H. A. Mohamed, Am. J. Anal. Chem., 2012, 3, 576–586 CrossRef.
- Y. Ling, Y. Yang, S. Bian and Y. Tu, Drug Test. Anal., 2010, 2, 388–391 CrossRef CAS PubMed.
- N. Alizadeh and R. Mehdipour, J. Pharm. Biomed. Anal., 2002, 30, 725–731 CrossRef CAS.
- Y. Umezawa, P. Bühlmann, K. Umezawa, K. Tohda and S. Amemiya, Pure Appl. Chem., 2000, 72, 1851–2082 CrossRef CAS.
- T. Masadome, J. Yang and T. Imato, Microchim. Acta, 2004, 144, 217–220 CrossRef CAS.
- I. Svancara, K. Vytras, J. Barek and J. Zima, Crit. Rev. Anal. Chem., 2001, 31, 311–345 CrossRef CAS.
- H. M. Abu-Shawish, J. Hazard. Mater., 2009, 167, 602–608 CrossRef CAS PubMed.
- H. M. Abu-Shawish, S. M. Saadeh, K. Hartani and H. M. Dalloul, J. Iran. Chem. Soc., 2009, 6, 729–737 CrossRef CAS.
- S. M. Saadeh, H. M. Abu Shawish, H. M. Dalloul, N. M. E. L. -Halabi and B. K. Daher, Mater. Sci. Eng., C, 2012, 32, 619–624 CrossRef CAS PubMed.
- J. Sànchez and M. Valle, Determination of Anionic Surfactants Employing Potentiometric Sensors—A Review, Crit. Rev. Anal. Chem., 2005, 35, 15–29 CrossRef.
- U. Schaller, E. Bakker, U. E. Spichiger and E. Pretsch, Anal. Chem., 1994, 66, 391–398 CrossRef CAS.
- M. Bagheri, M. H. Mashhadizadeh, S. Razee and A. Momeni, Electroanalysis, 2003, 15, 23–24 CrossRef.
- G. A. E. Mostafa and A. Al-Majed, J. Pharm. Biomed. Anal., 2008, 48, 57–61 CrossRef CAS PubMed.
- M. Shamsipur, M. Yousefi, M. Hosseini and M. R. Ganjali, Anal. Chem., 2002, 74, 5538–5543 CrossRef.
- R. P. Buck and E. Lindner, Pure Appl. Chem., 1994, 66, 2527–2536 CrossRef CAS.
- R. P. Buck and V. V. Cosofret, Pure Appl. Chem., 1993, 65(8), 1849–1858 CrossRef CAS.
- M. A. Mazlum, H. E. A. A. Naeimi, A. Dastanpour and A. Shamlli, Sens. Actuators, B, 2003, 96, 441–445 CrossRef.
- British Pharmacopia, Vol. 1 & II, (M. G. Lee), MHRA, Market Towers, 1 Nine Elms Lane, London SW8 5NQ, (2009).
|
This journal is © The Royal Society of Chemistry 2015 |
Click here to see how this site uses Cookies. View our privacy policy here.