DOI:
10.1039/C4BM00319E
(Paper)
Biomater. Sci., 2015,
3, 336-344
Media additives to promote spheroid circularity and compactness in hanging drop platform†
Received
4th September 2014
, Accepted 4th November 2014
First published on 13th November 2014
Abstract
Three-dimensional spheroid cultures have become increasingly popular as drug screening platforms, especially with the advent of different high throughput spheroid forming technologies. However, comparing drug efficacy across different cell types in spheroid culture can be difficult due to variations in spheroid morphologies and transport characteristics. Improving the reproducibility of compact, circular spheroids contributes to standardizing and increasing the fidelity of the desired gradient profiles in these drug screening three-dimensional tissue cultures. In this study we discuss the role that circularity and compaction has on spheroids, and demonstrate the impact methylcellulose (MethoCel) and collagen additives in the culture media can contribute to more compact and circular spheroid morphology. We demonstrate that improved spheroid formation is not a simple function of increased viscosity of the different macromolecule additives, suggesting that other macromolecular characteristics contribute to improved spheroid formation. Of the various macromolecular additives tested for hanging drop culture, MethoCel provided the most desirable spheroid formation. Additionally, the higher viscosity of MethoCel-containing media improved the ease of imaging of cellular spheroids within hanging drop cultures by reducing motion-induced image blur.
Introduction
Conventional methods for high throughput screening have primarily utilized two-dimensional cell cultures in well plates. Recently, the trend has been shifting towards three-dimensional (3-D) drug screening, especially in cancer therapeutics, due to the unique characteristics of these culture platforms. These cell aggregates allow for increased cell–cell interactions such as adhesion and junctional connections, reduced proliferation rates more aligned with physiological growth rates, restricted and non-linear diffusion gradients resulting in non-uniform metabolic gradients.1 Additionally, 3-D culturing is desirable as some cells have shown to lose their physiologic phenotypes and functions when cultured in two dimensional culture platforms, however, these phenotypes can be retrieved by culturing cells in 3-D emulating conditions.2 Spheroid culture has also been applied to creating complex co-culture systems.3 Multicellular spheroids represent an attractive platform because it provides a model of oxygen, metabolite, nutrient, and drug gradients observed in tumors due to non-uniform vascular perfusion in a radially symmetric architecture that is easier to reproduce and mathematically model.
Multicellular spheroid models have been generated through various methods, and reviewed thoroughly in recent literature.4,5 One of the common methods described is the ‘hanging drop’ technique, in which cells are placed in a suspended drop of media and as a result of gravity and the meniscus incurred by the air–liquid interface, cells localize at the bottom of the hanging drop, typically resulting in intercellular aggregation and adherence. This method of generating spheroids has been applied to fabricate 3-D tissues such as embryoid bodies, cancer tumor models, microlivers, microhearts, microcartilage.6–9 Spheroid culture in hanging drops has garnered popularity as a high throughput tool supported by published systems and methods for more standardized, robust generation and maintenance of hanging drop cultures.6,10,11 A major advantage of this system also extends from the ability to culture and image spheroids directly from the hanging drops over extended periods of time (weeks to months),11 whereas, in 2-D cultures, cells grown on the culture surface eventually form a confluent monolayer and begin to slough off the surface as a sheet or clump.
Requirements we believe critical to 3-D spheroid screening models are (1) tightly aggregated spheroids to facilitate physiologically relevant cell density, (2) the formation of diffusion gradients, and (3) tuneable and reproducible spheroid size based on initial seeding density. However, not all cell types have demonstrated spontaneous aggregation in spheroid cultures, resulting in loose aggregates, or disconnected, floating cells.12 This phenomenon poses a barrier in testing different cell types, which may only be able to establish weak intercellular interactions, if any, resulting in an inability to form spheroids. In order to overcome this inability to coalesce, researchers have applied different methods to induce cellular aggregation in cells of interest.4,5 One such method is the inclusion of additives to 3-D cultures for improved spheroid formation. Both biological and synthetic additives have been successfully employed, with mechanisms varying between cross-linking agents,13,14 adhesion stimulation,15 or rheological modifiers and crowding agents.16 Supplementation of cross-linking agents has generally been done with extracellular matrix proteins, such as collagen, fibronectin and reconstituted basement membrane matrigel.12,17 Additional methods of using cross-linkers to improve spheroidal aggregation include the use of polymer nanospheres13 or chemically modifying cell surfaces via biotinylation and culturing in the presence of supplemented avidin.14 Similar to using cross-linking agents, spheroid formation has also been accomplished by using β1-integrin-stimulating monoclonal antibodies.15 Unlike supplements that directly tether or act as a tether to cells, certain additives have improved the formation of spheroids through rheological modification or as a crowding agent. Methylcellulose (MethoCel) has been applied to spheroid formation and even though the mode of action has not been conclusively determined, it has been attributed to MethoCel's inert semi-solid gel like properties, or viscosity, or additionally crowding effect induced by the molecule.16,18,19 The addition of MethoCel to hanging drop cultures has been solely applied18 or co-supplemented with cross-linking additives, such as matrigel;17 these modifications have shown improvement in cellular aggregation and formation of 3-D structures. Additionally, supplemented cultures demonstrated increased reliability and robustness for spheroid formation; however concentrations of supplemented MethoCel have not been characterized.
In this study we aim to compare the individual and combined effects of two widely used additives (collagen and MethoCel) in hanging drop cultures to promote the circularity and compactness of 3-D spheroids for a variety of cell types. To focus exclusively on the impact of additives of spheroids within the context of high throughput screening, we used previously reported high throughput hanging drop array plates (Fig. S4†) coupled with a liquid handling robot to minimize sample variability, provide robust culturing conditions with minimal to no spreading, loss of shape, or rupturing of hanging drops as compared to the more commonly known inverted lid methodology.6 Using finite element models we first demonstrate the importance of these two morphologic parameters in the establishment of radial transport gradients, a hallmark feature of spheroid models. We then compare the effects of different macromolecule thickening agents in hanging drop cultures. Even though increasing viscosity results in a reduction of spheroid motion while imaging, we identify that modulating viscosity is not the sole requirement to induce spheroid formation, indicating the need to consider other molecular characteristics when selecting additives to promote spheroid morphology.
Results and discussion
Effects of spheroid geometry and physical characteristics on diffusion gradient
Diffusion kinetics is a major factor that influences cellular responses in 3-D spheroids. Nutrients and waste byproducts face diffusion resistance as they enter or leave a spheroid, respectively. Additional compounds in the surrounding medium will similarly develop gradients as they penetrate the spheroid. The rate of diffusion and steepness of the diffusion gradient are primarily influenced by the circularity and compactness of a spheroid. Circularity refers to the symmetry of a spheroid, with an ideal equidistant symmetry from the center of the spheroid to any point on the surface. This ideal symmetry would result in a concentric diffusion gradient field; facilitating robust modeling of molecular diffusion into the spheroid. In contrast, elliptical 3-D cellular masses would result in an increase of surface area and a reduction of average distance from the long axis to the spheroid's center of mass. As expected this was confirmed using a finite element model to compare oxygen diffusion into an ideal spheroid versus an ellipsoid of the same volume. Using oxygen diffusion coefficients20 and consumption rates21,22 from the literature, the model predicted the presence of a hypoxic region in an ideal spheroid, whereas this region is absent in an ellipsoid of the same volume with a 2
:
1 deformed aspect ratio (Fig. 1A). This simulation shows that spheroid geometry has a direct effect on the physical characteristics of transport gradients.
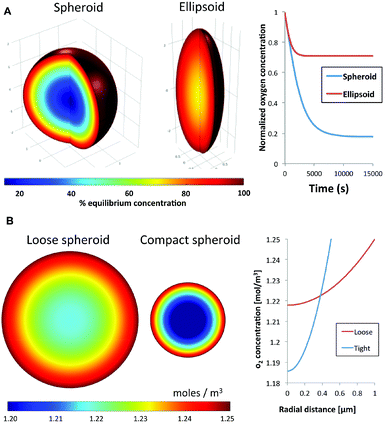 |
| Fig. 1 Computational models of oxygen gradients formed in a spheroid containing a constant number of cells with different circularity and compactness. (A) The model predicts that as the spheroid elongates and becomes less circular, the average core-to-surface distance decreases and the oxygen gradient decreases with the spheroid becoming more evenly saturated with oxygen. (B) Similarly, modeling of a loose, non-compact spheroid demonstrates shallower hypoxic gradient compared to a compact spheroid with the same number of cells due to the lower volumetric oxygen consumption rate. | |
Using the same approach we also estimated the effect of compactness on spheroid transport properties. Compactness refers to the degree of remodeling and density of a spheroid. As cells come into contact with each other, from the initial step of aggregation through spheroid formation, they can manipulate and secrete ECM proteins leading to reorganization and compaction. Here we simulate the effect of compaction by modeling the steady-state concentration of oxygen in two spheroids with an identical number of cells, and hence identical overall oxygen consumption rate. The first is a reference spheroid with uniform oxygen consumption rate, compared to a compact spheroid half the diameter (1/8 the volume). In other words, the compact spheroid would have a specific volumetric oxygen consumption rate that is eight times higher than the reference spheroid to account for the loss of volume. Assuming similar oxygen diffusion kinetics for both spheroids, the model predicted a steep hypoxic gradient from the surface to the core of the compact spheroid compared to the reference spheroid (Fig. 1B). These simulations indicate that the uniformity, strength and steepness of a solute gradient within a spheroid are directly proportional to the circularity and compactness, or morphology, of a spheroid. The simulations support the notion that spheroid responses to small molecules can be impacted significantly by spheroid morphology. Therefore any meaningful comparisons between pharmacokinetics of different drugs must be conducted using spheroid models with similar, reproducible physical characteristics.
Effect of additives on spheroid morphology in hanging drop cultures
Additives in hanging drops can affect the speed, quality, longevity and robustness of spheroid formation. Many of the advantages of spheroid culture, including transport limitation and establishment of a nutrient gradient, are based on the assumption that cells form a compact and symmetrical spheroid mass. Here, we assessed MethoCel, an additive that does not adhere to cells, in its ability to enhance spheroid formation. Unlike ECM proteins additives, such as collagen or fibronectin, MethoCel does not bias toward cell aggregation by acting as a scaffolding protein that binds multiple cells through surface receptors. However, given the widespread use of collagen as an aggregating/crosslinking agent in 3-D culture, we included it to serve as a comparison to MethoCell as well as assess the combined effects of collagen and MethoCel on spheroid formation using several cell lines.
One challenge in assessing spheroid morphology is to determine a standardized metric that correlates with spheroid function. As demonstrated with finite element models, circularity and compactness have a significant impact on transport gradients within spheroids. To this end, we developed a visual comparison-based, 5-point scale to assess circularity and compactness of spheroids. The uneven brightness of a spheroid and the background caused by the curvature of the hanging drop complicates the determination of the spheroid border necessary for computer image analysis. Circularity and compactness of each spheroid were scored by comparing phase-contrasted micrographs to the 5-stages of development for each metrics (Fig. S1†). The scores were generated blinded and independently by two researchers and the averages are presented as a gradient map (Fig. 2). For circularity measures, an automated image analysis system was also used where possible (Fig. S3†).
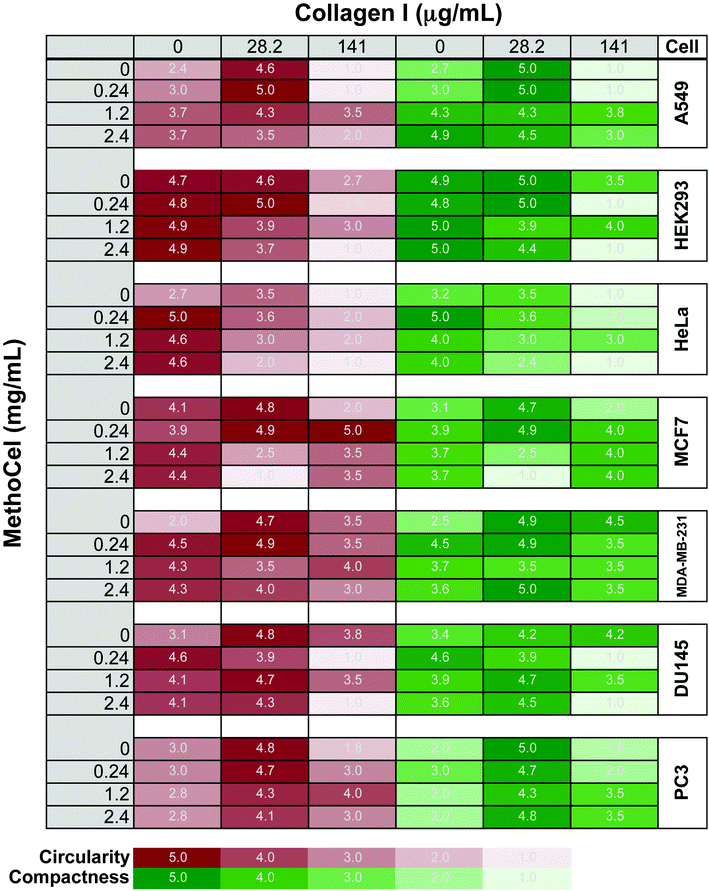 |
| Fig. 2 Circularity and compactness of spheroids after two days in culture. Several common tumor cell lines were tested. Circularity and compactness were rated on a 5-point scale by blinded observers (n = 2). Final score represent the average of 5 spheroids cultured in identical conditions. | |
We tested several tumor cell lines since these would be of most interest to drug screening applications. In agreement with previously observed results, spheroids generally become more circular and compact when supplemented with low concentration of collagen compared to unsupplemented control conditions.12 Whereas high collagen concentrations had varied responses, primarily a negative impact on spheroid circularity and compactness and in some cell types (A549 and HeLa) completely abrogating single spheroid formation, similar to previously reported formation of small multi-spheroids within cultures containing higher concentrations of Matrigel17). In contrast, cells behave in a much more uniform fashion in the presence of MethoCel. For most cells tested (DU145, A549, HeLa, MDA-MB-231, MCF-7), the presence of MethoCel improved circularity and compactness of spheroids in a dose dependent manner with varying degrees of effectiveness. The exceptions were HEK293 cells, where circularity and compactness scores were high and favorable regardless of MethoCel addition, and PC3 cells, where MethoCel conferred almost no improvement. In MethoCel only culture, further addition of MethoCel combined with low concentration of collagen did not enhance spheroid morphology in a dose dependent manner, but instead caused no change or negatively impacted spheroid morphology. Finally, high collagen concentration combined with MethoCel lead to mixed results with no discernible trends.
Like in most tissue constructs, cells remodel and reshape the spheroid over time. MethoCel demonstrated its ability in enhancing spheroid morphology in the acute phase of spheroid culture (2 days), but it was unclear whether such enhancement would be sustained over a long culture period. To test this we chose three tumor cell lines (MDA-MB-231, DU145 and PC3) to further examine the effects of MethoCel on monoculture spheroid formation over a period of 4 days. Spheroids consisting of either MDA-MB-231 or DU145 became more circular and compact from day 1 to 4 post seeding (Fig. 3). The addition of 0.24 mg mL−1 of MethoCel led to further enhancement over time when compared to cultures with no additives. Interestingly, PC3 spheroid morphology was minimally affected by culture time and presence of MethoCel. This finding suggests that the effectiveness of MethoCel may be cell-type dependent.
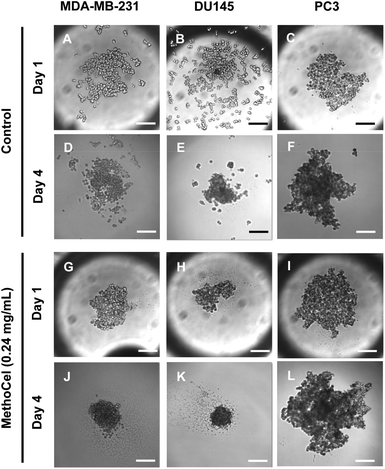 |
| Fig. 3 Changes in tumor spheroid morphologies over 3 days were monitored. The presence of MethoCel improved both acute and sustained spheroid circularity and compactness. Scale bar = 200 μm. | |
Spheroid formation generally improved using collagen concentrations within the range previously described in the literature.12,15,23 At higher concentrations, collagen led to formation of multiple spheroids instead of a single one as expected, reflecting previously reported results in increased Matrigel concentrations17 and in some cases, we observed significant adhesion to the hanging drop plate and formation of an elongated cell mass. The latter may be caused by non-specific absorption of protein, including collagen, onto the hanging drop plate that leads to subsequent cell adhesion and nucleation around the rim of the plate.
The final spheroid size in a hanging drop platform can be controlled by varying the initial cell seeding number in each droplet. The assumption is that higher initial cell numbers in a droplet will result in a larger spheroid, and this generally seems to hold true for different cell types10 and additive concentrations we have tested (data not shown). However, at high collagen concentration, cells may form clusters to create multiple nucleation sites as they are settling, which in turn increases the likelihood of multi-spheroid formation.17 Another aspect to consider is that some cell types have a natural tendency to form clusters of certain size irrespective of seeding density, especially in high ECM concentrations. This is seen in mammary epithelial cells, which form hollow acini with uniform size when seeded in Matrigel irrespective to cell quantity.24 These factors should be considered if the goal is to generate single spheroid within each hanging drop.
Viscosity mediated spheroid stability for improved imaging and analysis in hanging drop culture
Among the many techniques to assess anti-tumor drug efficacy, microscopic imaging remains a commonly used modality due to its simplicity and compatibility with online, automated high throughput screening. However, the motion blur associated with the vibration of the microscope or sample movement within the hanging drop can limit the quality of images, especially for long exposures necessary in fluorescence imaging. The free-floating nature of spheroids within the hanging drop culture results in the lack of steadfastness or spheroid stability, resulting in motion blur artifacts which can limit the ability to automate imaging of these high throughput spheroid arrays. We went on to confirm that MethoCel additionally reduces motion artifacts during microscopy of spheroids in hanging drops. By tracking spheroid movement and analyzing the center-of-mass path length we observed that as little as 0.024 mg mL−1 of MethoCel in medium is sufficient to reduce motion artifact in small or larger (25 and 500 cells, respectively) spheroids. In larger spheroids (500 cells), motion artifacts appeared to be slightly reduced as compared to smaller spheroids in the absence of MethoCel; however the stabilizing effect of MethoCel markedly reduced motion artifact in spheroids of both sizes tested (Fig. 4A). This secondary effect of MethoCel is particularly beneficial as it will improve the robustness to observe and image spheroids within the hanging drop culture. This is a significant finding as many of the clinically relevant hanging drop spheroid applications involve long-term culture of small (10 to 50 cells), patient-derived cell population in spheroid formats, which could benefit from a method to both improve spheroid formation and facilitate imaging of samples while not disrupting the longevity of culturing. Addition of MethoCel also did not change droplet contact angle (Fig. 4B) or evaporation rate (Fig. 4D) as compared to the control culture media, indicating that the motion dampening and subsequent spheroid stability was most likely conferred by viscosity as opposed to changes in Marangoni or thermo-convective flow profiles.
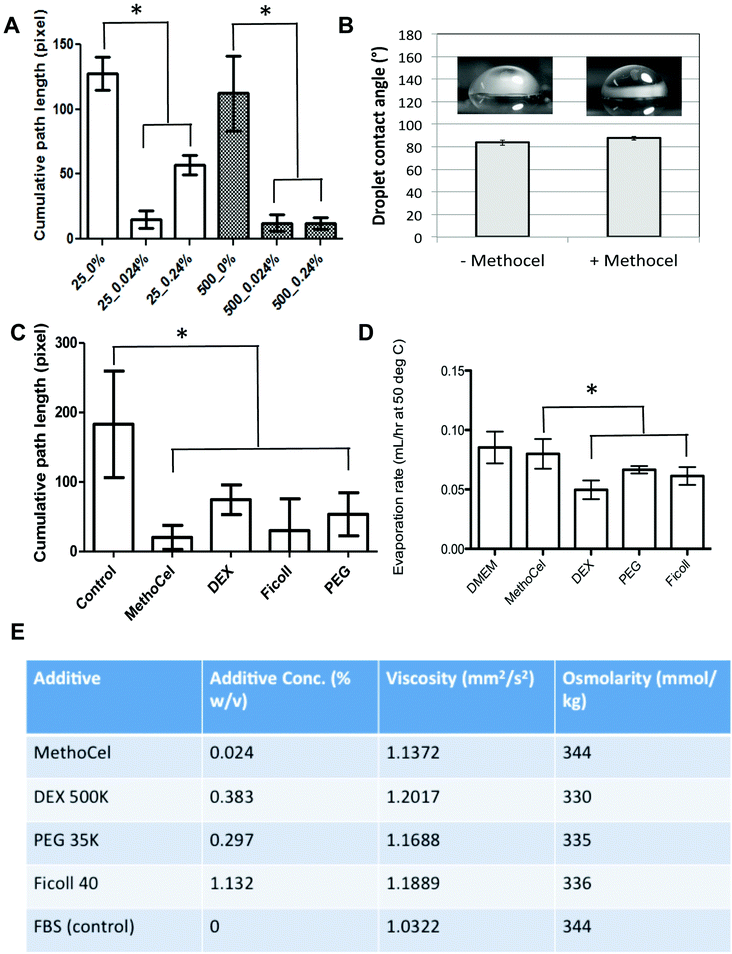 |
| Fig. 4 Physical characterization of spheroids in hanging drop culture. (A) Presence of MethoCel improved the stability of hanging drop culture (25 and 500 cells spheroids), evident by the reduction of cumulative path length compared to no additive controls (*P < 0.01). (B) The presence of MethoCel did not change the contact angle and evaporation rate of medium, suggesting minimal difference in thermo-convective flow within hanging drop culture. (C) Media containing DEX, PEG or Ficoll at comparable viscosity to medium with MethoCel led to similar level of motion artifact reduction compared to no additive control (25 cells spheroids, *P < 0.05). (D) The presence of DEX, Ficoll and PEG led to slight reduction in evaporation rates compared to MethoCel supplemented medium (*P < 0.05). (E) Comparison of viscosity and osmolarity between media with different additives and control (10% FBS in DMEM). | |
It is not surprising that the increase in viscosity imparted by the presence of MethoCel would dampen external vibration and reduce the amount of forces transmitted to the spheroid for improved stability. Due to MethoCel's viscosity mediated stabilization, and multiple descriptions eluding that its semi-solid, inert viscosity modulating properties maybe responsible for spheroid formation, we assessed other long chain macromolecules in their ability to achieve similar levels of spheroid stabilization, as well as their ability to promote spheroid formation. In fact we found other long chain macromolecules, including dextran (DEX), Ficoll and polyethylene glycol (PEG), supplemented to match 0.024% MethoCel viscosity (Fig. 4E) achieved similar level of motion artifact reduction for spheroid imaging (Fig. 4C) and slightly reduced evaporation rates (Fig. 4D).
Regardless of comparable viscosity, we identified that of the additives tested, MethoCel resulted in the most improved spheroid circularity and compactness in poor-spheroid forming cells, such as MDA-MB-231. Conversely, these macromolecule additives may interfere with spheroid formation, in a cell-type dependent manner, as noted when they are present in HEK293 spheroid culture. Specifically, even though all HEK293 cultures resulted in the formation of spheroids regardless of additives, each hanging drop culture with either PEG or DEX supplements resulted in the formation of multiple spheroids (Fig. 5). As previously discussed, multi-spheroids in a single droplet are non-ideal for screening applications, further indicating that regardless of viscosity modification there are secondary macromolecule effects necessary for robust promotion of spheroids in hanging drop cultures. Such factors may be crowding or “swelling” effects which could produce localization of ECM proteins25,26 or the aggregation of cells27 within the hanging drop cultures. These phenomena have been demonstrated to result in mechanisms that should induce particle and cellular aggregation when using certain macromolecules as additives,27 one such macromolecule being MethoCel.
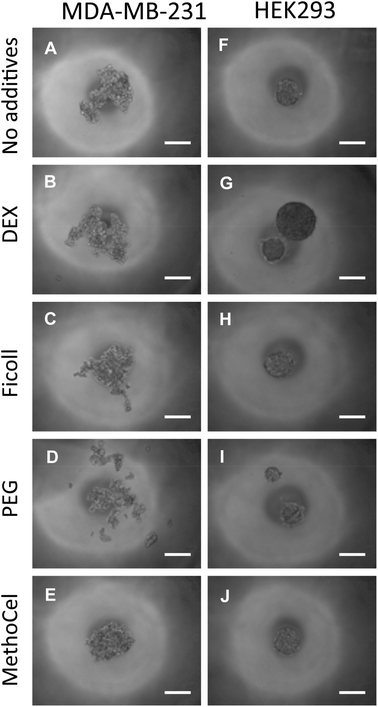 |
| Fig. 5 Effects of polymer additive in spheroid formation. Media containing polymer additives (formulation in Fig. 4E) were used to culture MDA-MB-231 and HEK293 cells. The presence of MethoCel (E) improved spheroid morphology in non-spheroid forming MDA-MB-231 cells. Other polymers (B, C and D) produced spheroids similar to control (A). In normally spheroid forming HEK293 cells (F), the presence of DEX (G) and PEG (I) led to the appearance of satellite bodies. MethoCel (J) and Ficoll (H) led to spheroids with normal morphologies. Scale bar = 200 μm. | |
Experimental procedures
Cell culture
Cells were cultured at 37 °C at 5% CO2 using a culture medium composed of Dulbecco's Modified Eagle's Medium (DMEM) (Invitrogen) supplemented with 10% Fetal Bovine Serum (FBS) and 1% antibiotic-antimycotic. Cells were cultured in 100 mm culture dishes until reaching ∼80% confluence and were then passaged using 0.25% Trypsin/EDTA (Gibco) to detach cells from plates prior to diluting and transferring them to the hanging drop plates. All cell lines were obtained from ATCC. Cell lines cultured for spheroid formation were human embryonic kidney HEK 293 (ATCC CRL-1573), breast cancer MDA-MB 231 (ATCC CRM-HTB-26) and MCF7 (ATCC HTB-22), lung cancer A549 (ATCC CCL-185), cervical cancer cells HeLa (ATCC CCL-2), prostate cancer PC3 (ATCC CRL-1435) and DU145 (ATCC HTB-81).
Spheroid culturing and assessment for methyl cellulose and collagen type I
Prior to usage, a hydrophilic coating (0.1%, Pluronic F108, BASF Co., Ludwigshafen, Germany) is applied onto the entire hanging drop plate surface. The plate is subsequently UV sterilized before cell seeding. To form hanging drops, cell suspension solution is pipetted from the top side through the access holes with the end of each pipette tip inserted into the access hole to guide the sample liquid to the bottom surface. Spheroid formation was tested for the different cell types across multiple concentrations of A4M MethoCel (Dow Chemical, MI) and bovine type I collagen (Corning, NY). The following concentrations for collagen 0, 0.3%, 1.5% were paired with the following concentrations of MethoCel 0, 2, 10, 20% of stock MethoCel (1.2% w/v). These different conditions were assessed to determine spheroid formation as a result of the additives in the media. These combinations were assessed and used to generate a gradient map of increased spheroid formation. Samples were assessed and compared to the spheroid formation scale seen in ESI (Fig. S1†).
Spheroid morphology scoring
Brightfield images of spheroids were collected at 10× magnification and assigned scores based on circularity and compactness. These images were randomized and scored by blinded observers. Observers were given a series of images describing the criteria for each metrics and were asked to score each image out of a 5-point scale. These scores were averaged and tabulated with gradient scale to allow visual comparison of the effects of medium additives across different cell lines.
Viscosity measurement of medium with additives
Solutions were prepared for viscosity testing using culture media composed of DMEM (LifeTechnologies, Carlsbad, CA) with 10% FBS and 1% antibiotic-antimycotic (Life Technologies, Carlsbad, CA) with the addition of the different additives. Viscosity testing was done on a total of five solution types, culture media as well as the four solutions with additives. Additives were placed into the culture media to match the viscosity of MethoCel 0.024% w/v in culture media. The following additives were used: Dextran 500 K (Pharmacosmo), PEG 35 K (Sigma), and Ficoll 40 (Sigma) to generate similar viscosity with the different additives. Viscosity testing was done using a 52501/0b ubbelohde viscometer, testing each sample 5 times to determine average viscosity. The viscosity was determined prior to using the solutions as a spheroid culturing solution. Upon using the different solutions, spheroid formation was assessed against each culture condition.
Spheroid stability measurement
The stabilizing effects of polymer additives in hanging drop spheroid culture were assessed by video analysis. Spheroids with initial cell seeding numbers of 25 or 500 cells were cultured in medium with or without polymer additives (MethoCel, PEG, DEX, or Ficoll) for 2 days. Polymer-supplemented media were formulated to have comparable viscosities and osmolarities (Fig. 4E). Spheroids in hanging drop plates were imaged on a Nikon Eclipse Ti inverted microscope at 10× magnification for 10 seconds at a frame rate of 100 frame s−1. Using an open source cell tracking software,28 the recorded images were analyzed to determine the center of mass of each spheroid. The stability of spheroid was defined as the cumulative path length using a template-matching based tracking method. Cumulative path length was calculated by summing the total distance covered by the center of mass over 10 seconds (1000 frames total). In cases where cells formed more than one spheroid, the largest one out of the group was measured.
Oxygen depletion simulations
Finite element simulations were conducted in a commercially available finite element package (Comsol v. 4.2; Burlington, MA). To study the effect of spheroid shape on oxygen distributions, cells were assumed to consume oxygen equally throughout the cell aggregate. Equivalent-volume (33.5 μL) spherical and ellipsoidal geometries were generated using a 2D axisymmetric model, and the ‘transport of diluted species’ module was used to model oxygen consumption and transport within the spheroid. Appropriate parameters were selected based on values previously reported in the literature (diffusion coefficient D = 2 × 10−10 m2 s−1,20 oxygen consumption rate k = 3.09 × 10−4 mol m−3 s−1.21,22) To adjust for oxygen consumption differences in loose spheroids, the volumetric oxygen consumption rate was reduced by a factor equivalent to the expanded volume of the spheroid.
Conclusions
The multicellular spheroid model has found applications in many fields of biomedical research and will play a major role in next-generation drug-screening platforms. Using computational modeling we have demonstrated that the morphology of a spheroid expressed as circularity and compactness, are both important parameters that determine its transport characteristics. We found that the morphology of a spheroid is a function of cell type but can also be affected by the presence of additives in culture medium. We investigated the effect of collagen, an adhesive ECM protein, and MethoCel, a cell repelling polysaccharide, on spheroid morphologies. For all cell types tested, use of moderate amounts of an appropriate additive improved spheroid morphology. MethoCel was beneficial for all cells except PC3 cells. Collagen, at low concentration, was beneficial for all cells except HeLa cells. Combinations of MethoCel and collagen in medium yielded mixed results depending on the cell type, highlighting the importance of tailoring additive formulations for specific spheroid models. The additive formulation that was always better than no additive was a combination of a low concentration of Methocel (0.24 mg mL−1) and collagen (28.2 μg mL−1). To obtain the highest degree of morphology improvement, however, each cell type would have to be tested for a broader range of additive formulations. In addition, we tested other macromolecule – supplemented medium formulations with similar viscosity and osmolarity as MethoCel and saw no improvement in spheroid morphology. This finding implies that the spheroid enhancement property of MethoCel cannot be entirely attributed to medium thickening alone. Overall, our results are in agreement with others in the literature and suggest that additives may affect spheroid morphologies by other biophysical factors unrelated to medium viscosity, such as altering ECM availability in the peri-cellular space.
Acknowledgements
The authors would like to acknowledge funding support from NIH (CA165463) and the mCube grant from the University of Michigan. SCLP was supported by National Science Foundation Graduate Research Fellowship Program and the National Institutes of Health Cellular Biotechnology Training Program under grant no. DGE 1256260 (ID: 2011101670) and grant no. NIH GM008353 respectively. CM supported by a Banting postdoctoral fellowship from the Natural Sciences and Engineering Research Council of Canada.
References
- F. Hirschhaeuser, H. Menne, C. Dittfeld, J. West, W. Mueller-Klieser and L. A. Kunz-Schughart, Multicellular tumor spheroids: an underestimated tool is catching up again, J. Biotechnol., 2010, 148(1), 3–15 CrossRef CAS PubMed.
- E. Fennema, N. Rivron, J. Rouwkema, C. van Blitterswijk and J. de Boer, Spheroid culture as a tool for creating 3D complex tissues, Trends Biotechnol., 2013, 31(2), 108–115 CrossRef CAS PubMed.
- W. Wirz, M. Antoine, C. G. Tag, A. M. Gressner, T. Korff, C. Hellerbrand and P. Kiefer, Hepatic stellate cells display a functional vascular smooth muscle cell phenotype in a three-dimensional co-culture model with endothelial cells, Differentiation, 2008, 76(7), 784–794 CrossRef CAS PubMed.
- R. Z. Lin and H. Y. Chang, Recent advances in three-dimensional multicellular spheroid culture for biomedical research, Biotechnol. J., 2008, 3(9–10), 1172–1184 CrossRef CAS PubMed.
- H. Kurosawa, Methods for inducing embryoid body formation: in vitro differentiation system of embryonic stem cells, J. Biosci. Bioeng., 2007, 103(5), 389–398 CrossRef CAS PubMed.
- Y. C. Tung, A. Y. Hsiao, S. G. Allen, Y. S. Torisawa, M. Ho and S. Takayama, High-throughput 3D spheroid culture and drug testing using a 384 hanging drop array, Analyst, 2011, 136(3), 473–478 RSC.
- N. E. Timmins, S. Dietmair and L. K. Nielsen, Hanging-drop multicellular spheroids as a model of tumour angiogenesis, Angiogenesis, 2004, 7(2), 97–103 CrossRef PubMed.
- J. M. Kelm, E. Ehler, L. K. Nielsen, S. Schlatter, J. C. Perriard and M. Fussenegger, Design of artificial myocardial microtissues, Tissue Eng., 2004, 10(1–2), 201–214 CrossRef CAS PubMed.
- I. Wagner, E. M. Materne, S. Brincker, U. Sussbier, C. Fradrich, M. Busek, F. Sonntag, D. A. Sakharov, E. V. Trushkin, A. G. Tonevitsky, R. Lauster and U. Marx, A dynamic multi-organ-chip for long-term cultivation and substance testing proven by 3D human liver and skin tissue co-culture, Lab Chip, 2013, 13(18), 3538–3547 RSC.
- A. Y. Hsiao, Y. C. Tung, X. Qu, L. R. Patel, K. J. Pienta and S. Takayama, 384 hanging drop arrays give excellent Z-factors and allow versatile formation of co-culture spheroids, Biotechnol. Bioeng., 2012, 109(5), 1293–1304 CrossRef CAS PubMed.
- A. Y. Hsiao, Y. C. Tung, C. H. Kuo, B. Mosadegh, R. Bedenis, K. J. Pienta and S. Takayama, Micro-ring structures stabilize microdroplets to enable long term spheroid culture in 384 hanging drop array plates, Biomed. Microdevices, 2012, 14(2), 313–323 CrossRef CAS PubMed.
- A. Ivascu and M. Kubbies, Diversity of cell-mediated adhesions in breast cancer spheroids, Int. J. Oncol., 2007, 31(6), 1403–1413 CAS.
- S. J. Gwak, D. Choi, S. S. Paik, S. W. Cho, S. S. Kim, C. Y. Choi and B. S. Kim, A method for the effective formation of hepatocyte spheroids using a biodegradable polymer nanosphere, J. Biomed. Mater Res., Part A, 2006, 78(2), 268–275 CrossRef PubMed.
- P. A. De Bank, Q. Hou, R. M. Warner, I. V. Wood, B. E. Ali, S. Macneil, D. A. Kendall, B. Kellam, K. M. Shakesheff and L. D. Buttery, Accelerated formation of multicellular 3-D structures by cell-to-cell cross-linking, Biotechnol. Bioeng., 2007, 97(6), 1617–1625 CrossRef CAS PubMed.
- R. Z. Lin, L. F. Chou, C. C. Chien and H. Y. Chang, Dynamic analysis of hepatoma spheroid formation: roles of E-cadherin and beta1-integrin, Cell Tissue Res., 2006, 324(3), 411–422 CrossRef CAS PubMed.
- P. Longati, X. Jia, J. Eimer, A. Wagman, M. R. Witt, S. Rehnmark, C. Verbeke, R. Toftgard, M. Lohr and R. L. Heuchel, 3D pancreatic carcinoma spheroids induce a matrix-rich, chemoresistant phenotype offering a better model for drug testing, BMC Cancer, 2013, 13, 95 CrossRef CAS PubMed.
- J. D. Haeger, N. Hambruch, M. Dilly, R. Froehlich and C. Pfarrer, Formation of bovine placental trophoblast spheroids, Cells Tissues Organs, 2011, 193(4), 274–284 CrossRef CAS PubMed.
- E. Urich, C. Patsch, S. Aigner, M. Graf, R. Iacone and P. O. Freskgard, Multicellular self-assembled spheroidal model of the blood brain barrier, Sci. Rep., 2013, 3, 1500 Search PubMed.
- S. M. Dang, M. Kyba, R. Perlingeiro, G. Q. Daley and P. W. Zandstra, Efficiency of embryoid body formation and hematopoietic development from embryonic stem cells in different culture systems, Biotechnol. Bioeng., 2002, 78(4), 442–453 CrossRef CAS PubMed.
- N. Sasaki, H. Horinouchi, A. Ushiyama and H. Minamitani, A new method for measuring the oxygen diffusion constant and oxygen consumption rate of arteriolar walls, Keio J. Med., 2012, 61(2), 57–65 CrossRef.
- W. Mueller-Klieser, Method for the determination of oxygen consumption rates and diffusion coefficients in multicellular spheroids, Biophys. J., 1984, 46(3), 343–348 CrossRef CAS.
- D. R. Grimes, C. Kelly, K. Bloch and M. Partridge, A method for estimating the oxygen consumption rate in multicellular tumour spheroids, J. R. Soc., Interface, 2014, 11(92), 20131124 CrossRef PubMed.
- A. Ivascu and M. Kubbies, Rapid generation of single-tumor spheroids for high-throughput cell function and toxicity analysis, J. Biomol. Screening, 2006, 11(8), 922–932 CrossRef CAS PubMed.
- J. Debnath and J. S. Brugge, Modelling glandular epithelial cancers in three-dimensional cultures, Nat. Rev. Cancer, 2005, 5(9), 675–688 CrossRef CAS PubMed.
- C. Chen, F. Loe, A. Blocki, Y. Peng and M. Raghunath, Applying macromolecular crowding to enhance extracellular matrix deposition and its remodeling in vitro for tissue engineering and cell-based therapies, Adv. Drug Delivery Rev., 2011, 63(4–5), 277–290 CrossRef CAS PubMed.
- A. Satyam, P. Kumar, X. Fan, A. Gorelov, Y. Rochev, L. Joshi, H. Peinado, D. Lyden, B. Thomas, B. Rodriguez, M. Raghunath, A. Pandit and D. Zeugolis, Macromolecular crowding meets tissue engineering by self-assembly: a paradigm shift in regenerative medicine, Adv. Mater., 2014, 26(19), 3024–3034 CrossRef CAS PubMed.
- N. Kojima, S. Takeuchi and Y. Sakai, Rapid aggregation of heterogeneous cells and multiple-sized microspheres in methylcellulose medium, Biomaterials, 2012, 33(18), 4508–4514 CrossRef CAS PubMed.
- A. Sacan, H. Ferhatosmanoglu and H. Coskun, CellTrack: an open-source software for cell tracking and motility analysis, Bioinformatics, 2008, 24(14), 1647–1649 CrossRef CAS PubMed.
Footnotes |
† Electronic supplementary information (ESI) available. See DOI: 10.1039/c4bm00319e |
‡ Equal contribution. |
|
This journal is © The Royal Society of Chemistry 2015 |
Click here to see how this site uses Cookies. View our privacy policy here.