Ion exchange-precipitation for nutrient recovery from dilute wastewater
Received
30th May 2015
, Accepted 22nd July 2015
First published on 23rd July 2015
Abstract
Regulated phosphorus (P) and nitrogen (N) discharges and the cost of fertilizer provide economic drivers for nutrient removal and recovery from wastewater. This study used ion exchange (IX) in dilute (domestic) wastewater to concentrate nutrients with subsequent recovery by struvite precipitation. This is the first tertiary wastewater treatment study directly comparing P removal using a range of Fe, Cu, and Al-based media followed by clinoptilolite IX columns for N removal and precipitation using the combined regenerants. Phosphate removal prior to breakthrough was 0.5–2.0 g P Lmedia−1, providing effluent concentrations <0.1 mg L−1 PO4-P and <0.2 mg L−1 NH4-N for ≥80 bed volumes. Dow-FeCu resin provided effective P removal, efficient neutral pH regeneration and 560 mg P L−1 in the regeneration eluate (≥100× concentration factor). Exchange capacity of clinoptilolite in column mode was 3.9–6.1 g N Lmedia−1 prior to breakthrough. Precipitation using the combined cation and anion regenerants resulted in a maximum of 74% P removal using Dow-FeCu. Precipitates contained impurities, including Al3+, Ca2+, and Fe. Overall, the IX-precipitation recovery process removed ≥98% P and 95% N and precipitates contained 13% P and 1.6% N. This sequential process can satisfy increasingly stringent wastewater standards and offers an effective alternative to traditional treatment technologies that simply remove nutrients. Approximately 84% of total P and 97% of total Kjeldahl N entering a treatment plant can be captured (accounting for primary clarifier removal), whereas most existing technologies target side streams that typically contain only 20–30% of influent P and 15–20% of influent N.
Water impact
Wastewater treatment targets removal of nitrogen (N) and phosphorus (P). Productive agriculture also depends on N and P, where P is a nonrenewable resource, diminishing reserves of which encourage not only removal from wastewater, but also recovery for fertilizer use. This research advances sustainable, economic approaches to domestic wastewater treatment through recovery of clean water and valuable nutrient fertilizers.
|
Introduction
The macronutrients nitrogen (N) and phosphorus (P) are commonly applied to improve agricultural yields.1 Currently, the Haber–Bosch process produces 500 million tons per year of N fertilizer, accounting for 1% of the world's energy consumption.2,3 Phosphate (PO43−) rock is mined to produce PO43− fertilizer, and depletion of available reserves is possible within the next 60–240 years.4 A substantial portion of P fertilizer demand (≈15%) could be satisfied by municipal wastewater facilities.1,5,6 To comply with effluent permits, municipalities commonly remove N through nitrification/denitrification,7 while P is removed in the biosolids through precipitation and biological uptake.6 Although these processes successfully remove nutrients from wastewater, agricultural reuse of biosolids is commonly regulated and P bioavailability can be inhibited by Fe and Al binding.6,8 A more sustainable approach is to recover the N and P removed to satisfy discharge permits and recycle it as a valuable fertilizer product.
Ion exchange (IX), or the exchange of ions of similar charge between liquid and solid phases, is a promising method of concentrating nutrients for subsequent recovery.9–11 Ammonium (NH4+) and PO43− are removed using cationic and anionic IX media, respectively. Clinoptilolite is a natural zeolite that preferentially exchanges NH4+.12 Synthetic PO43−-selective media have been developed, including: Dow-Cu, Dow-HFO-Cu, LayneRT™ and others.9,13–15 Yet, improved selectivity of PO43− remains a critical need to facilitate use in full-scale applications.11,16 Small-scale column tests of polymeric ligand exchangers indicate that improved selectivity of PO43− sorption and controlled release to recover the PO43− can be achieved using hydrated ferric oxide and/or Cu2+ loading.9,14,17–19 Upon exhaustion of surface exchange sites, the media is regenerated with brine solution, producing concentrated P or N eluate. Subsequent treatment processes, such as struvite precipitation, can recover concentrated P and N from the eluate in a readily reusable form.20,21
Clinoptilolite's NH4-N removal and recovery capacity is well-defined22,23 and previous work has determined that high pH regenerants improve desorption;24 however, excessive pH can negatively impact subsequent NH4-N capacity.25 In the current work, optimal regenerant NaCl concentration and pH were identified through multiple cycles of IX batch tests. Additionally, the concentration of cations and the pH of the regeneration eluate were analyzed as these have not previously been well-defined and these characteristics affect downstream processes (e.g., precipitation). This study also evaluated the mass of P exchanged (g Premoved Lmedia−1) and effluent PO4-P concentration using a range of Fe, Cu, or Al-based media, direct wastewater comparisons of which have not been reported. The pH and concentrations of PO4-P and metals of the regeneration eluate were analyzed.
Struvite (MgNH4PO4) precipitation has previously been tested in anion exchange regeneration eluates9,26 and using select combinations of cation and anion regeneration eluates.16,21,27,28 The present study directly compared performance of cation eluate combined with a range of P-specific anion regeneration eluates to precipitate N and P from dilute wastewater. Nutrient recovery and purity of the fertilizer were evaluated as these parameters directly influence process economics. Results of this work advance practical application of nutrient recovery by improving upon existing treatment options to design economical nutrient recovery processes for dilute wastewaters. This recovery scheme can produce effluent which satisfies nutrient discharge limits while recovering a valuable fertilizer product. Furthermore, the IX-precipitation nutrient recovery process can facilitate implementation of more sustainable treatment trains such as those utilizing anaerobic membrane bioreactors, which avoid the costs of aerobic-driven nutrient removal.
Materials and methods
Anion exchange tests were conducted with PO43−-selective media. Three commercially available IX resins were purchased ready-to-use: LayneRT™ (Layne Christensen Co., The Woodlands, TX) and a neutral and basic activated alumina (AA, Sigma Aldrich, Milwaukee, WI). Four anionic media were prepared in the lab using the base resin Dowex™ M4195 (Dow Chemical Co., Midland, MI). Dow-Cu was prepared in accordance with Zhao and Sengupta (1996).14 Preparation of Dow-NAB-Cu followed the same process excluding the acid and base pretreatment steps. The hydrated ferric oxide (HFO) resin, Dow-HFO, was produced as described by Sengupta and Pandit (2011).9 The Dow-HFO and Dow-Cu preparation methods were conducted sequentially to produce a resin loaded with iron and copper, denoted as Dow-FeCu in this work, similar to Dow-HFO-Cu described by Sengupta and Pandit (2011).9
Clinoptilolite (St. Cloud Zeolite, Tucson, AZ) in the sodium aluminosilicate form was utilized for NH4-N exchange. The exchange sites were saturated with Na+ by adding 25 g of zeolite to a 0.5 L bottle and rinsing 3× with deionized (DI) water to remove fine particles, similar to the method described by Weatherley and Miladinovic (2004).29 Next, 0.5 L of 1% NaCl was added and the mixture was placed on a shaker table at 150 rpm for 2 d. The solution was discarded and the media was rinsed 2× with DI water and dried at 103 °C for 24 h.
Batch IX studies
To determine the effect of various regenerants on subsequent IX capacity, zeolite batch testing was conducted using a shaker table at room temperature. Two sets of zeolite experiments were conducted in triplicate: zeolite with NH4-N solution and zeolite with mixed cations. Bottles containing zeolite and 40 mg L−1 NH4-N solution were evaluated using varying regenerant solutions, as listed in Table 2. Initial kinetic tests demonstrated that sorption equilibrium was achieved within 4 days. Thus, all tests were conducted under the following conditions: 128 mL solution, 4 d IX duration, 2 h regeneration duration, and 150 rpm rotation speed. IX and regeneration were conducted for 3 cycles; zeolite was rinsed with DI water 2× after each regeneration cycle. The concentration of NH4-N in the final IX and regeneration solutions was analyzed to determine removal and desorption, respectively. A mixed cation solution (representative of a typical dilute wastewater) containing 40 mg NH4-N L−1, 35 mg Ca2+ L−1, 10 mg K+ L−1, 22 mg Mg2+ L−1, and 140 mg Na+ L−1 was used to determine competitive uptake and the optimal regenerant solution for zeolite.
To determine the capacity of each anion exchange media, PO4-P batch testing was conducted using 100 mg L−1 PO4-P in DI water. One IX cycle followed by one regeneration cycle was conducted for each media to determine percent P removal and desorption. All media/regenerant pairs tested are shown in Table 1.
Table 1 Phosphate removal and desorption in batch tests using anionic IX resin
Resin |
% removala |
Regenerantb |
% desorptiona |
Average results of triplicate measures ±1 standard deviation.
pH of all regenerants is that at which the media was prepared, with the exception of LayneRT™, for which regenerant pH was based on Blaney et al. (2007).35
|
DOW-Cu |
76 ± 0.1 |
6% NaCl, pH = 4.3 |
60 ± 5.4 |
DOW-FeCu |
64 ± 1.4 |
6% NaCl, pH = 8 |
65 ± 3.2 |
LayneRT™ |
60 ± 1.6 |
2% NaCl + 2% NaOH, pH > 14 |
49 ± 3.9 |
Dow-NAB-Cu |
53 ± 1.0 |
6% NaCl, pH = 4.3 |
38 ± 2.9 |
DOW-HFO |
52 ± 0.4 |
6% NaCl, pH = 8 |
8 ± 1.9 |
AA neutral |
32 ± 1.4 |
6% NaCl, pH = 7.5 |
31 ± 4.0 |
AA basic |
28 ± 1.1 |
6% NaCl, pH = 9.5 |
53 ± 14 |
Column IX studies
To determine exchange capacity under more realistic operating conditions, the top three performing anion resins and zeolite were tested using rapid small-scale column tests, as illustrated in Fig. 1. Secondary wastewater effluent was passed through 1.5 μm filters and used as column influent. This pre-filtration step was used to model realistic operating conditions for scenarios with low TSS due to tertiary filtration. As stricter regulatory compliance is required and water reuse becomes increasingly economical, membrane filtration, including membrane bioreactors, is being increasingly implemented, thereby providing excellent effluent for subsequent IX processes. Column effluent was collected every 2 h using a CF-1 Fraction Collector (Spectrum Chromatography, Houston, TX). Influent and effluent were analyzed as described in the Analytical Procedures section. Regression analysis was completed for the effluent PO4-P or NH4-N to determine P or N removal prior to breakthrough, defined as 0.1 mg L−1 PO4-P and 1.5 mg L−1 NH4-N based on Wisconsin's discharge limits.30,31
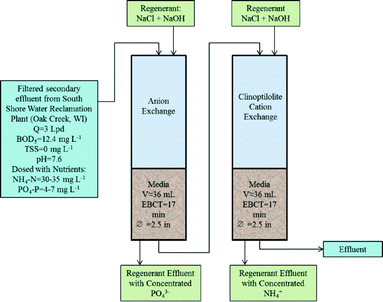 |
| Fig. 1 Schematic of rapid small-scale column testing system. | |
Columns were regenerated with brine solution (based on optimal batch-scale testing) following the IX cycle. Regenerant effluent samples were initially collected after every 5–20 mL of brine passed, and every 50–100 mL as testing progressed. Regression analysis was conducted to estimate PO4-P or NH4-N desorption over time. This enabled quantification of percent nutrient desorption and nutrient concentrations in regeneration eluate at a range of brine volumes. Adequate IX capacity during subsequent cycles (as a result of P or N desorption) and elevated nutrient concentrations improve cost effectiveness of the process.
Precipitation
Precipitation was conducted in Erlenmeyer flasks by combining one anion regeneration eluate, zeolite regeneration eluate, MgCl2 solution and NaOH for pH adjustment. Solutions were mixed with a target Mg
:
P
:
N ratio of 1
:
1
:
1 and a pH of 10. The mixture was stirred at 150 rpm for 10 min and then allowed to settle for 30 min. Supernatant was analyzed for PO4-P, NH4-N and cations. Percent removal was calculated and solids composition was estimated using a mass balance approach. For further evaluation, predicted precipitants were modelled using MINEQL+ v.4.62.3 and MINTEQ v.3.1 chemical equilibrium software. Struvite was added to the MINEQL+ custom thermodynamics database using pK = 13.26.32
Analytical procedures
Standard analytical methods33 were used, including: phenate method 4500-NH3-F for NH4-N; cadmium reduction method 4500-NO3-E for NO3-N; turbidimetric method for SO42−; and method 2320 B for alkalinity. Additional analyses included the ascorbic acid method34 for PO4-P and the Hach mercuric thiocyanate method for Cl−. Absorbance was measured using a Thermo Scientific (Waltham, MA) Genesys 20 spectrophotometer. An ICP-MS (7700 Series, Agilent Technologies, Santa Clara, CA) was used to measure Ca, Mg, K, Na, Al, Fe, Cu, Be, Cr, Mn, Co, Ni, Zn, Se, As, Mo, Pb, Hg, Ag, and Cd. A Thermo Scientific Orion 4 STAR meter was used for pH.
Results and discussion
Phosphate exchange capacity
Batch tests showed that ≥60% P removal was achieved using Dow-Cu, Dow-FeCu, and LayneRT™, as shown in Table 1. Elimination of the acid/base treatment step for Dow-Cu (Dow-NAB-Cu) decreased performance by almost 25%. Despite both Dow-HFO and LayneRT™ media utilizing HFO particles, which predominantly remove PO43− by ligand exchange,35 Dow-HFO underperformed LayneRT™. Both basic and neutral activated alumina performed poorly in comparison to other media. The three resins with the greatest P removal (Dow-Cu, Dow-FeCu, and LayneRT™) also provided the greatest P desorption (≥49%), suggesting that they offer the greatest potential for P recovery operations. These media were used for subsequent column testing. Greater phosphate removal potential of these media stems from their incorporation of transition metal cations Fe and Cu. Polyvalent metals such as these exhibit strong ligand adsorption of phosphate species through Lewis acid–base interactions, i.e., formation of inner-sphere complexes.17,36
Effluent PO4-P concentration during each column run is shown in Fig. 2a. All three media maintained effluent PO4-P <0.1 mg L−1 for ≥80 bed volumes (BV). Achieving this low concentration is a critical study outcome as it is sufficient to satisfy increasingly lower wastewater discharge limits which may not be feasible using conventional precipitation and biological treatment processes.18 These results suggest that LayneRT™ was the optimal media as it required no preparation and provided the longest bed life prior to breakthrough, thereby maximizing P removal.
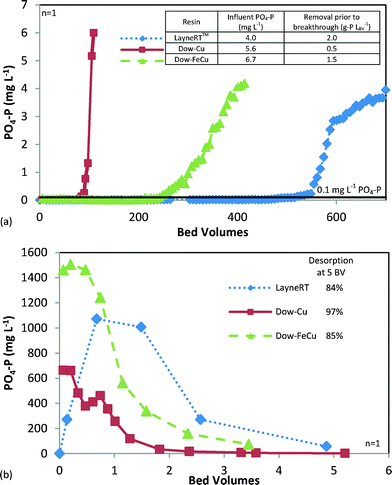 |
| Fig. 2 PO4-P concentrations from column testing of anionic media showing (a) phosphate breakthrough comparison of IX media and (b) desorption comparison showing regeneration eluate concentration. | |
Regeneration performance with anionic media
After exhaustion of the anion exchange columns, media was regenerated with brine solution, as identified in Table 1. Regeneration was conducted to determine total P desorption, volume of regenerant required, and characteristics of the regeneration eluate. Effluent PO4-P and percent desorption after 5 BV are shown in Fig. 2b. In order to achieve 80% P desorption, 3.5 BV of regenerant was needed for LayneRT™, 3 BV for Dow-FeCu and 1.1 BV for Dow-Cu. Based on regression analysis, the regeneration eluates contained approximately 400–550 mg PO4-P L−1 at 80% desorption, thereby concentrating PO4-P ≥100×. This was sufficient for effective precipitation as full-scale struvite precipitation reactors are typically applied to side streams with >150 mg L−1 PO4-P.37,38
The pH of Dow-Cu and Dow-FeCu regeneration eluates was approximately 9.6–9.9, whereas LayneRT™ eluate was 14. As optimal pH for struvite precipitation is 8–9,39 significant pH adjustment would be required when using LayneRT™ eluate to precipitate struvite. LayneRT™ eluate contained 155 mg L−1 Al3+, while 7 and 30 mg L−1 Cu2+ were measured in Dow-Cu and Dow-FeCu eluates, respectively. Due to the detection of high metal concentrations in the regeneration eluates, there is a significant risk for co-precipitation of metals with struvite, resulting in decreased fertilizer value. Based on the results, Dow-FeCu is the optimal media for P desorption as it provided the greatest concentration of P (550 mg L−1) and the regeneration eluate pH was closest to optimal precipitation conditions.
Optimization of zeolite regeneration
High pH improves N desorption from zeolite;24 however, excessive pH can cause attrition.25 Batch tests were conducted using NH4Cl solution to identify the optimal pH and the effect of increasing NaCl concentration on long-term NH4-N exchange capacity. As shown in Table 2, initial tests were performed using 7 alternative regenerants. Regeneration tests did not conclusively identify an optimal NaCl concentration with respect to NH4-N removal. Regeneration solutions with pH ≥ 13 negatively impacted NH4-N exchange after 3 cycles. Based on these results, zeolite was tested in a mixed cation solution using lower pH regenerants.
Table 2 Removal of NH4-N using varying zeolite regenerant solutions
|
NH4-N-only solution after 3 cycles |
Mixed ion solution after 5 cycles |
Regenerant solution: |
5% NaCl |
8% NaCl |
10% NaCl |
7.5% NaCl + 0.5% NaOH |
0.5% NaOH |
2% NaOH |
2% Ca(OH)2 |
0.1% Ca(OH)2 |
0.1% NaOH |
8% NaCl |
7.5% NaCl pH 12 |
Average results of triplicate data ±1 standard deviation.
|
|
|
|
|
|
|
|
|
pH |
7.5 |
8 |
8.3 |
13 |
13 |
13.7 |
13.7 |
12 |
12 |
8 |
12 |
Total NH4-N removala (%) |
70 ± 6.3 |
85 ± 0.6 |
76 ± 4.2 |
55 ± 1.5 |
66 ± 8.2 |
44 ± 4.2 |
0 ± 0 |
40 ± 1.6 |
56 ± 3.8 |
70 ± 0.2 |
71 ± 4.6 |
As shown in Table 2, regeneration with 8% NaCl and 7.5% NaCl (pH = 12) resulted in similar NH4-N removal after 5 cycles. Regeneration with 0.1% NaOH or 0.1% Ca(OH)2 was ineffective, likely due to the lower concentration of cations driving desorption of NH4-N. A pH of 12 did not negatively impact IX, whereas pH of 13 did negatively impact removal after repeated IX cycles. Additionally, the data suggests that NaCl concentration can be reduced without diminishing capacity, with 2% NaCl being sufficient.24
Zeolite IX performance and regeneration efficiency
Zeolite was tested in columns to determine the media's NH4-N removal capacity when treating anion exchange effluent. Two IX cycles were conducted to determine the change in capacity following regeneration, as shown in Fig. 3a. Breakthrough was defined as 1.5 mg L−1 NH4-N, which falls within the waterbody nutrient criteria range of 0.2–5 mg L−1 total nitrogen (TN) developed by some states.31 The effluent remained below this concentration for ≥100 BVs, and was less than 0.2 mg L−1 throughout the first IX cycle. Total removal prior to breakthrough was 3.9 g N LBV−1 and 6.1 g N LBV−1 for the first and second cycles, respectively. The variability in zeolite capacity was likely due to differences in influent cations. Concentrations of Ca2+ and Mg2+ in the influent during cycle 2 were lower, enabling greater NH4-N exchange. The N removal capacities are comparable to zeolite tests performed using similar wastewater.23,40
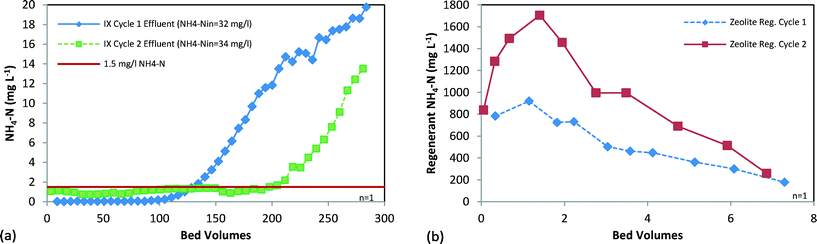 |
| Fig. 3 Two cycles of column testing of NH4-selective zeolite showing (a) ammonium IX breakthrough and (b) desorption showing regeneration eluate concentration. | |
Zeolite was regenerated to evaluate reuse of the media for IX and to determine the regeneration eluate characteristics. Based on batch testing, 6% NaCl (pH = 11) regenerant was used. As shown in Fig. 3b, 74% and 68% of N was desorbed during cycles 1 and 2, respectively. Regeneration may potentially be improved by raising the regenerant pH and reducing NaCl concentrations to improve N desorption.
The eluate characteristics of the first two BV of regenerant are shown in Table 3. The eluate contains substantial amounts of Ca2+ and K+, which may precipitate along with struvite, thereby decreasing the purity of the product. A reduction in fertilizer quality will reduce the value of the product and therefore the revenue.41
Table 3 Zeolite regeneration eluate characteristics for column tests
Regeneration cycle |
pH |
NH4-N |
K+ |
Ca2+ |
Mg2+ |
1 |
8.7 |
600 |
660 |
350 |
23 |
2 |
7.7 |
740 |
320 |
390 |
24 |
Precipitation efficiency and solids composition
The overarching process goal is recovery of struvite, a slow release fertilizer that performs comparably to standard fertilizers.6 Precipitation tests were conducted to determine P and N recovery as well as product purity. Precipitation was accomplished by combining cation and anion IX column regeneration eluates containing high concentrations of N and P, respectively, adding Mg2+ and adjusting the pH to 10. After settling the solids, the supernatant was analyzed to determine the P, N and metals concentrations, as shown in Table 4. Greater than 70% P removal was achieved with LayneRT™ and Dow-FeCu regeneration eluates. Precipitation ceased at P concentrations of approximately 60–80 mg L−1. This was likely due to limited multivalent cations such as Mg2+ and Ca2+; however, it has also been shown that greater Ca
:
Mg ratios reduce P removal efficiency.42 The majority of Al3+, Ca2+ and Fe present in the initial concentrated regeneration solutions precipitated, which impacts product quality, and would necessitate additional testing to determine the precipitated product's potential as a fertilizer. This is a substantial issue for the LayneRT™ regeneration eluate as the Al3+ concentration exceeds 100 mg L−1.
Table 4 Initial ion concentration and percent removal for precipitation of combined anion and zeolite regeneration eluates
Anion regeneration eluate source: |
LayneRT™ |
DOW-Cu |
DOW-FeCu |
Ion |
Initial (mg L−1) |
% removala |
Initial (mg L−1) |
% removala |
Initial (mg L−1) |
% removala |
Average results of triplicate data ±1 standard deviation.
No result due to analytical error.
|
PO4-P |
270 |
71 ± 1.6 |
120 |
28 ± 1.0 |
230 |
74 ± 1.7 |
NH4-N |
120 |
19 ± 1.8 |
53 |
16 ± 0.9 |
100 |
22 ± 1.6 |
Mg2+ |
170 |
96 ± 0.8 |
84 |
66 ± 2.5 |
270 |
62 ± 2.7 |
Ca2+ |
71 |
81 ± 4.0 |
31 |
45 ± 0.2 |
65 |
98 ± 0.6 |
Al3+ |
78 |
92 ± 0.2 |
0.28 |
58 ± 16 |
0.15 |
|
Fe |
5.8 |
76 ± 0.2 |
7.3 |
79 ± 0.3 |
5.2 |
100 ± 0.0 |
Cu2+ |
0.28 |
59 ± 3.5 |
22 |
9.5 ± 0.3 |
12 |
14 ± 0.5 |
K+ |
130 |
6.5 ± 1.5 |
58 |
12 ± 2.5 |
111 |
|
Removal of N was less than 25% in all precipitation tests, whereas 100% was targeted. P was likely being precipitated as calcium phosphates in addition to struvite and therefore N removal was lower than expected. Chemical equilibrium modeling confirmed that under these conditions Ca and Mg-based species such as hydroxyapatite, calcium phosphates, and magnesium phosphates were likely outcompeting struvite precipitation. Improved precipitate purity may be obtained by decreasing the pH to 8.743 (compared to 10, as in this study). Additionally, a reactor seeded with struvite pellets can be operated at a lower pH, such as 7.3, and achieve high P removal.44 Therefore, future testing should be conducted with struvite seeding at neutral pH to minimize chemical requirements and reduce Ca2+ precipitation while maximizing P and N removal.
A mass balance analysis was performed to estimate solids composition in order to assess fertilizer quality. The precipitates formed after combining LayneRT™ and zeolite regeneration eluate contained: 13% P, 1.6% N, 11% Mg, 3.9% Ca, 0.6% K, 0.3% Fe, and 5.3% Al. In comparison, pure struvite should contain 13% P, 5.7% N, and 10% Mg. The amount of P and Mg in the precipitate from the lab test is similar to pure struvite; however, the N content is low, as discussed previously. Additionally, there are significant amounts of Ca2+ and Al3+, which could negatively impact the fertilizer value of the precipitated product.
Conclusions
The ultimate goal of nutrient recovery is to produce a marketable nutrient-rich product in addition to meeting nutrient discharge limits for the treated water. The IX-precipitation process evaluated here concentrated and precipitated P and N to accomplish this objective using dilute wastewater. A range of Fe, Cu, or Al-based media were used for direct performance comparison in wastewater. Using the top-performing PO43−-selective resins, removal prior to column breakthrough was 0.5–2.0 g P Lmedia−1, which provided effluent concentrations <0.1 mg L−1 PO4-P for ≥80 BV and <0.2 mg L−1 NH4-N for ≥100 BV. Dow-FeCu provided the most effective P removal, efficient neutral pH regeneration and 560 mg L−1 P in the regeneration eluate (concentration factor ≥ 100). Greater than 98% PO4-P and 97% NH4-N were removed using combined anionic and cationic column regenerants for precipitation. The regenerant streams also contained substantial levels of Al, Cu, Ca, and/or K, which may diminish the value of the recovered product. Recommended future tests include continued testing of anion exchange media to determine the most economical media considering cost of preparation, P removal and selectivity, P desorption, chemical requirements and minimal metal leaching. Furthermore, the precipitation method can be adjusted to improve struvite purity and to evaluate the potential use of supernatant from precipitation as a recycled regenerant. Overall economics of the IX-precipitation process is of key interest for larger-scale implementation, and evaluation of chemical and energy costs, regenerant reuse, and price point of the recovered fertilizer product must be considered together in future assessments.
Acknowledgements
This work was supported by Xylem, Inc. The authors would like to thank Mike Dollhopf and Patrick Mullen for their assistance in sample analysis and Joe Heffron for performing chemical modelling. Additionally, the authors thank Milwaukee Metropolitan Sewerage District for providing wastewater samples.
References
- D. Cordell, J.-O. Drangert and S. White, The story of phosphorus: Global food security and food for thought, Glob. Environ. Change, 2009, 19(2), 292–305 CrossRef PubMed
.
-
A. Dinar and R. O. Mendelsohn, Handbook on Climate Change and Agriculture, MA: Edward Elgar Publishing, Inc., Northhampton, 2011 Search PubMed
.
- B. Smith, Nitrogenase reveals its inner secrets, Science, 2002, 297(5587), 1654–1655 CrossRef CAS PubMed
.
- I. Steen, Phosphorus availability in the 21st century: Management of a non-renewable resource, Phosphorus Potassium, 1998, 217, 25–31 Search PubMed
.
-
WERF, Nutrient Recovery State of the Knowledge, 2011, Available at: http://www.werf.org/c/2011Challenges/Nutrient_Recovery.aspx Search PubMed
.
- B. E. Rittmann, B. Mayer, P. Westerhoff and M. Edwards, Capturing the lost phosphorus, Chemosphere, 2011, 84(6), 846–853 CrossRef CAS PubMed
.
- Y.-H. Ahn, Sustainable nitrogen elimination biotechnologies: A review, Process Biochem., 2006, 41(8), 1709–1721 CrossRef CAS PubMed
.
-
G. O'Connor, H. Elliot, D. Graetz and S. Brinton, WERF Report Biosolids and Residuals: Characterizing Phosphorus in Biosolids, Commercial Fertilizers, and Manures (phase 1), 2002 Search PubMed.
- S. Sengupta and A. Pandit, Selective removal of phosphorus from wastewater combined with its recovery as a solid-phase fertilizer, Water Res., 2011, 45(11), 3318–3330 CrossRef CAS PubMed
.
- B. K. Mayer, D. Gerrity, D. Reisinger and S. Brandt-Williams, Innovative strategies to achieve low total phosphorus concentrations in high water flows, Crit. Rev. Environ. Sci. Technol., 2013, 43(4), 409–411 CrossRef CAS PubMed
.
- D. Petruzzelli, L. De Florio, A. Dell'Erba, L. Liberti, M. Notarnicola and A. K. Sengupta, A new phosphate-selective sorbent for the Rem Nut process. Laboratory investigation and field experience at a medium size wastewater treatment plant, Water Sci. Technol., 2003, 48(1), 179–184 CAS
.
- A. Hedstrom, Ion exchange of ammonium in zeolites: A literature review, J. Environ. Eng., 2001, 127(8), 673–681 CrossRef CAS
.
- R. S. S. Wu, K. H. Lam, J. M. N. Lee and T. C. Lau, Removal of phosphate from water by a highly selective La(III)-chelex resin, Chemosphere, 2007, 69(2), 289–294 CrossRef CAS PubMed
.
- D. Zhao and A. Sengupta, Selective removal and recovery of PO4 in a novel fixed bed process, Water Sci. Technol., 1996, 33(10–11), 139–147 CrossRef CAS
.
- X. Zhu and A. Jyo, Column-mode phosphate removal by a novel highly selective adsorbent, Water Res., 2005, 39(11), 2301–2308 CrossRef CAS PubMed
.
- L. Liberti, D. Petruzzelli and L. De Florio, REM NUT ion exchange plus struvite precipitation process, Environ. Technol., 2001, 22(11), 1313–1324 CrossRef CAS PubMed
.
- D. Zhao and A. K. Sengupta, Ultimate removal of phosphate from wastewater using a new class of polymeric ion exchangers, Water Res., 1998, 32(5), 1613–1625 CrossRef CAS
.
- B. Pan, J. Wu, B. Pan, L. Lv, W. Zhang, L. Xiao, X. Wang, X. Tao and S. Zheng, Development of polymer-based nanosized hydrated ferric oxides (HFOs) for enhanced phosphate removal from waste effluents, Water Res., 2009, 43(17), 4421–4429 CrossRef CAS PubMed
.
- T. Nur, M. A. H. Johir, P. Loganathan, T. Nguyen, S. Vigneswaran and J. Kandasamy, Phosphate removal from water using an iron oxide impregnated strong base anion exchange resin, J. Ind. Eng. Chem., 2014, 20(4), 1301–1307 CrossRef CAS PubMed
.
- A. L. Forrest, K. P. Fattah, D. S. Mavinic and F. A. Koch, Optimizing struvite production for phosphate recovery in WWTP, J. Environ. Eng., 2008, 134(5), 395–402 CrossRef CAS
.
- J. A. O'Neal and T. H. Boyer, Phosphorus recovery from urine and anaerobic digester filtrate: comparison of adsorption–precipitation with direct precipitation, Environ.
Sci.: Water Res. Technol., 2015, 9–12 Search PubMed
.
- S. Aiyuk, J. Amoako, L. Raskin, A. Van Haandel and W. Verstraete, Removal of carbon and nutrients from domestic wastewater using a low investment, integrated treatment concept, Water Res., 2004, 38(13), 3031–3042 CrossRef CAS PubMed
.
- E. Cooney, G. Stevens, N. Booker and D. Shallcross, Ammonia removal from wastewaters using natural Australian zeolite. II. Pilot-scale study using continuous packed column process, Sep. Sci. Technol., 1999, 34(14), 2741–2760 CrossRef CAS PubMed
.
- X. Guo, L. Zeng and X. Jin, Advanced regeneration and fixed-bed study of ammonium and potassium removal from anaerobic digested wastewater by natural zeolite, J. Environ. Sci., 2013, 25(5), 954–961 CrossRef CAS
.
- J. H. Koon and W. J. Kaufman, Ammonia removal from municipal wastewaters by ion exchange, J. – Water Pollut. Control Fed., 2014, 47(3), 448–465 Search PubMed
.
- M. Kumar, M. Badruzzaman, S. Adham and J. Oppenheimer, Beneficial phosphate recovery from reverse osmosis (RO) concentrate of an integrated membrane system using polymeric ligand exchanger (PLE), Water Res., 2007, 41(10), 2211–2219 CrossRef CAS PubMed
.
- L. Liberti, N. Limoni, A. Lopez and R. Passino, The 10 m3h−1 RIM-NUT demonstration plant at West Bari for removing and recovering N and P from wastewater, Water Res., 1986, 20(6), 735–739 CrossRef CAS
.
- L. Liberti, G. Boari and R. Passino, Phosphates and ammonia recovery from secondary effluents by selective ion exchange with production of a slow-release fertilizer, Water Res., 1979, 13(1), 65–73 CrossRef CAS
.
- L. R. Weatherley and N. D. Miladinovic, Comparison of the ion exchange uptake of ammonium ion onto New Zealand clinoptilolite and mordenite, Water Res., 2004, 38(20), 4305–4312 CrossRef CAS PubMed
.
- Wisconsin Department of Natural Resources. Phosphorus Fact Sheet. Available at: http://www.fdl.wi.gov/cofuploads/FACT_SHEET.pdf
.
- Wisconsin Department of Natural Resources, Procedures for Calculating Water Quality Based Effluent Limitations for Toxic and Organoleptic Substances Discharged to Surface Waters, 2012 Search PubMed.
- M. Ronteltap, M. Maurer and W. Gujer, Struvite precipitation thermodynamics in source-separated urine, Water Res., 2007, 41(5), 977–984 CrossRef CAS PubMed
.
-
APHA, in Standard Methods for the Examination of Water and Wastewater, ed. A. D. Eaton, L. S. Clescen, E. W. Rice and A. E. Greenberg, 21st edn, D.C.: American Public Health Association, Washington, 2005 Search PubMed
.
-
USEPA, Methods for Chemical Analysis of Water and Wastes, OH: USEPA, Cincinnati, 1983 Search PubMed
.
- L. M. Blaney, S. Cinar and A. K. SenGupta, Hybrid anion exchanger for trace phosphate removal from water and wastewater, Water Res., 2007, 41(7), 1603–1613 CrossRef CAS PubMed
.
-
D. A. Dzombak and F. M. Morel, Surface Complexation Modeling: Hydrous Ferric Oxide, NJ: John Wiley & Sons, Hoboken, 1990 Search PubMed
.
- M. I. H. Bhuiyan, D. S. Mavinic and F. A. Koch, Phosphorus recovery from wastewater through struvite formation in fluidized bed reactors: A sustainable approach, Water Sci. Technol., 2008, 57(2), 175–181 CrossRef CAS PubMed
.
- D. S. Mavinic, F. A. Koch, H. Huang and K. V. Lo, Phosphorus recovery from anaerobic digester supernatants using a pilot-scale struvite crystallization process, J. Environ. Eng. Sci., 2007, 6(5), 561–571 CrossRef CAS
.
- Z. Bradford-Hartke, P. Lant and G. Leslie, Phosphorus recovery from centralised municipal water recycling plants, Chem. Eng. Res. Des., 2012, 90(1), 78–85 CrossRef CAS PubMed
.
-
USEPA, Manual: Nitrogen Control, OH: USEPA, Cincinnati, 1993 Search PubMed
.
- A. Uysal, Y. D. Yilmazel and G. N. Demirer, The determination of fertilizer quality of the formed struvite from effluent of a sewage sludge anaerobic digester, J. Hazard. Mater., 2010, 181(1–3), 248–254 CrossRef CAS PubMed
.
- H.-J. Hwang and E. Choi, Nutrient control with other sludges in anaerobic digestion of BPR sludge, Water Sci. Technol., 1998, 39(1), 295–302 CrossRef
.
- J. Wang, J. G. Burken, X. Zhang and R. Surampalli, Engineered struvite precipitation: lmpacts of component-ion molar ratios and pH, J. Environ. Eng., 2005, 131(10), 1433–1440 CrossRef CAS
.
- A. Adnan, F. Koch and D. Mavinic, Pilot-scale study of phosphorus recovery through struvite crystallization - II: Applying in-reactor supersaturation ratio as a process control parameter, J. Environ. Eng., 2003, 2(6), 473–483 CrossRef CAS
.
|
This journal is © The Royal Society of Chemistry 2015 |
Click here to see how this site uses Cookies. View our privacy policy here.