DOI:
10.1039/C4FO00610K
(Paper)
Food Funct., 2015,
6, 192-202
Isolation and chemical identification of lipid derivatives from avocado (Persea americana) pulp with antiplatelet and antithrombotic activities
Received
13th July 2014
, Accepted 22nd September 2014
First published on 23rd September 2014
Abstract
Platelets play a pivotal role in physiological hemostasis. However, in coronary arteries damaged by atherosclerosis, enhanced platelet aggregation, with subsequent thrombus formation, is a precipitating factor in acute ischemic events. Avocado pulp (Persea americana) is a good source of bioactive compounds, and its inclusion in the diet as a source of fatty acid has been related to reduced platelet aggregability. Nevertheless, constituents of avocado pulp with antiplatelet activity remain unknown. The present study aims to characterize the chemical nature of avocado constituents with inhibitory effects on platelet aggregation. Centrifugal partition chromatography (CPC) was used as a fractionation and purification tool, guided by an in vitro adenosine diphosphate (ADP), arachidonic acid or collagen-platelet aggregation assay. Antiplatelet activity was initially linked to seven acetogenins that were further purified, and their dose-dependent effects in the presence of various agonists were contrasted. This process led to the identification of Persenone-C (3) as the most potent antiplatelet acetogenin (IC50 = 3.4 mM) among the evaluated compounds. In vivo evaluations with Persenone A (4) demonstrated potential protective effects against arterial thrombosis (25 mg kg−1 of body weight), as coagulation times increased (2-fold with respect to the vehicle) and thrombus formation was attenuated (71% versus vehicle). From these results, avocado may be referred to as a functional food containing acetogenin compounds that inhibit platelet aggregation with a potential preventive effect on thrombus formation, such as those that occur in ischaemic diseases.
Introduction
According to the World Health Organization, cardiovascular diseases (CVD) are the leading cause of mortality and morbidity, representing about 23% of the deaths worldwide.1 CVD, including acute myocardial infarctions, and cerebrovascular diseases are clinical manifestations of acute arterial thrombosis,2 after disruption of the hemostatic balance. Blood platelets, endothelial walls, and coagulation and fibrinolytic factors are key regulators of this delicate balance.
Platelets are essential in physiological hemostasis because they can be activated and mobilized rapidly to control bleeding through blood clot formation.3 However, under pathological conditions of the cardiovascular system, rupture or erosion of atherosclerotic plaques can occur, leading to vascular injury. Subsequent platelet interaction with the exposed sub-endothelial extracellular matrix at sites of injury triggers their activation, uncontrolled aggregation and thrombus formation. The thrombus blocks blood flow resulting in ischemic tissue injury and organ dysfunction.4
Hence, pharmacological agents with antiplatelet actions are considered to be fundamental therapies in the prevention of atherothrombotic events.5 However, antiplatelet agents, such as aspirin, are often associated with an increased risk of bleeding and frequently require gastric protection medications.6 Therefore, exploration of alternative sources of compounds that inhibit platelet aggregation continues to be necessary.
In this regard, avocado pulp (Persea americana) is a good source of bioactive compounds such as monounsaturated fatty acids and sterols, which may play a protective role against arterial thrombosis.7–9 Intervention trials in human subjects using avocado pulp as a source of monounsaturated fatty acids have demonstrated a significant decrease in ex vivo platelet aggregation induced by ADP.10 However, no information is available regarding the precise identity of the avocado molecules associated with antiplatelet action.
In the present study we describe the bioassay-guided isolation and identification of lipophilic constituents from avocado pulp, based on their in vitro ADP-induced platelet aggregation inhibitory properties. Initial stages of the isolation process involved the evaluation of antiplatelet activity of semi-crude fractions, obtained from partitioning of avocado pulp solids in a liquid–liquid biphasic system and in sub-fractions separated by CPC. Purification and chemical identifications were subsequently performed for compounds contained in the active fractions. Antiplatelet activity of purified compounds was then characterized, using different agonists to induce aggregation. Finally, the in vivo effects of one of the active molecules on blood clotting time and thrombosis were evaluated in CD1 mice.
Materials and methods
Plant material
Avocado fruits (Persea americana Mill, cv. var. Hass) were kindly provided by Avomex International (Sabinas, Coah., México) and were obtained from the region of Uruapan, Mich., México (19°25′0′′N 102°4′0′′O). Avocado pulp was manually separated from seeds, pureed, vacuum packed and stored at −80 °C prior to use, to avoid enzymatic activity, since it has been observed that enzyme lipoxygenase oxidizes acetogenins.
Isolation of semi-purified fractions for preliminary activity screening
An acetone extract E001 was obtained from freeze-dried avocado pulp, which was partitioned in a heptane–methanol biphasic system, and further fractionated by centrifuge partition chromatography (CPC). Bioactive fractions were grouped to finally obtain seven fractions (coded as GF01 to GF07), following the grouping procedures previously described.11 Subsequently, evaluation of ADP-induced platelet aggregation inhibitory properties of these seven fractions was performed as further described. Chromatographic profiles and the identity of the compounds present in fractions with higher antiplatelet activity were obtained using the HPLC-PDA/TOF method described in the next section.
Large scale isolation and purification of active constituents
Preparation of an acetogenin-enriched extract.
In order to further validate the potential antiplatelet activity of compounds present in fractions with higher antiplatelet activity, a large scale isolation and purification process was developed to recover them (Fig. 1). An acetone extract E001 from freeze-dried avocado pulp (240 g) was obtained and partitioned in a two non-miscible solvent system comprised of heptane–methanol (1
:
1 v/v), according to Rodriguez-Sanchez et al.11 Phase E002 (upper) and phase E003 (lower) were separated and washed with methanol saturated with heptane or heptane saturated with methanol (1
:
1 v/v), respectively. Phases were separated and concentrated under reduced pressure, yielding heptane- and methanol-soluble semicrude subfractions: E004 and E005 (derived from E002, respectively), and E006 and E007 (resulting from E003, respectively). Chromatographic profiles of sub-fractions E001 to E007 were obtained using the HPLC-PDA method further described in this section.
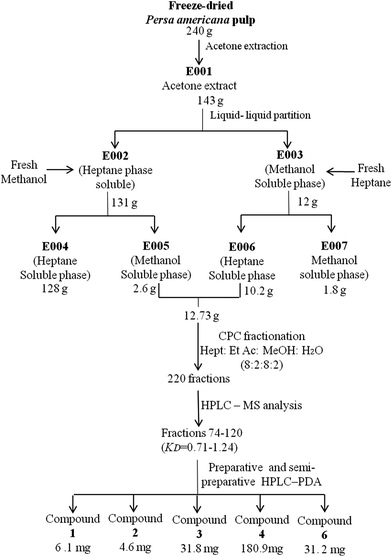 |
| Fig. 1 Flow chart of the process developed to isolate and purify the compounds identified as constituents of sub-fractions (KD = 0.71–1.24) that exhibited higher antiplatelet activity (GF04 to GF07) in the preliminary activity screening. | |
CPC fraction of an acetogenin-enriched extract.
After HPLC-PDA evaluation, E005 and E006 sub-fractions were mixed and further fractionated in a 1 L CPC system (Kromaton Technologies, Angers, France) using heptane–ethyl acetate–methanol–water (8
:
2
:
8
:
2) as a solvent system. The upper phase (UP) of the solvent system served as the stationary phase (SP), and after hydrodynamic equilibrium establishment, the lower phase (LP) accounted for 18% of the total column volume. Extracts E005 and E006 (12.73 ± 0.77 g), dissolved in 30 mL of UP and 80 mL of LP, were injected into the CPC column. Based on the elution–extrusion approach,12 LP was used to elute fractions for 170 min and then UP was pumped for 100 more minutes, both at a 10 mL min−1 flow rate. A total of 240 fractions (10 mL per fraction) were collected and their corresponding partition coefficients (KD) were calculated as described by Berthod et al. (2007).12 Aliquots (0.5 mL) of every 10 fractions were taken, evaporated under a stream of nitrogen and resuspended in isopropanol (0.5 mL) for further HPLC-PDA and HPLC-MS-TOF analysis as further described. At the end of the CPC run, the column was entirely filled with the SP, so it was ready to be reequilibrated again by pumping LP, and subsequent chromatographic fractionations (4) were carried out as previously described.
Acetogenin purification.
Consecutive HPLC separations were carried out in a preparative Phenomenex Prodigy C18 column (250 × 20 mm, 5 μm), using water 100% (A) and methanol 100% (B) as eluents, at a 20 mL min−1 flow rate. The solvent gradient was: 0–4 min, 75–85% B linear; 4–22 min, 85% B isocratic; 22–24 min, 85–95% B linear; 24–32 min, 95% B isocratic. A photodiode (PDA) detector was set at 220 nm. The final purification was conducted in a semi-preparative Phenomenex Synergi Hydro-RP column (250 × 4.6 mm, 4 μm), using 100% water and 100% methanol as mobile phases (A and B, respectively) at a flow rate of 1 mL min−1. Isocratic methods were optimized for each peak. The platelet aggregation (induced by ADP, collagen and arachidonic acid) inhibitory activity of purified molecules was evaluated as further described. One of the purified molecules exhibiting antiplatelet activity was selected for further evaluation of its performance as an antiplatelet agent in in vivo studies.
Identification of active compounds
For chemical identity the compounds were subjected to HPLC-PDA and HPLC-MS analysis as previously described by Rodriguez-Sanchez.11 Chemical identity was assigned by comparison of spectroscopic data with values reported in the literature and with data from standards isolated in our laboratory from avocado seeds.11,13
In vitro assays
Platelet aggregation.
This study was conducted in accordance with the Helsinki Declaration. All human donors from the blood bank of Instituto Nacional de Cardiología Ignacio Chávez previously signed informed consent. Blood samples were taken from healthy volunteers who had not taken any medications for at least 2 weeks, or ingested any alcohol for at least 24 h prior to sample collection. Blood was collected by venipuncture into Vacutainer (BD Diagnostics, Plymouth, UK) tubes containing 3.8% sodium citrate as an anticoagulant (at a 9
:
1 ratio, v/v). Platelet-rich plasma (PRP) and platelet-poor plasma (PPP) were obtained as earlier reported by De la Peña.14 The assays were carried out within 2 h after the blood had been drawn.
Evaluation of platelet aggregation was performed by turbidimetric measurements according to Falkenberg15 with some modifications. PRP adjusted with PPP to a platelet count of 2.5 × 108 mL−1 (215 μL) was pre-incubated at 37 °C for 10 min with the evaluated sample (10 μL) and at various concentrations. Platelet aggregation was initiated by the addition of 25 μL of the platelet agonist. The final concentrations of agonists in the reaction mixture were 20 μM ADP, 500 μM arachidonic acid or 5 μg mL−1 collagen, all obtained from Chrono-PAR Corporation (Havertown, PA, USA). Aggregation response was recorded for 10 min using a Chrono-log Model 700 Whole Blood/Optical Lumi-Aggregometer (Chrono-Log, Havertown, PA, USA). Maximal aggregation (MA) observed for samples containing avocado extracts, at different degrees of purification, was compared to those of vehicle controls (DMSO 2 M) evaluated under the same experimental conditions. Percent aggregation inhibitions, for all samples, were calculated using the following equation: %Inhibition = (MA vehicle control − MA extract)/MA vehicle control × 100%.
To verify the possible platelet cytotoxic effects of avocado extracts at different degrees of purification, cell viability was measured using CellTiter-Blue (Promega, Madison, WI, USA) and by trypan blue exclusion, counting live/dead platelets.
In vivo assays
Animal use and treatment.
Experiments were conducted in accordance with the Mexican National Protection Laws on Animal Protection and the General Health Law Related to Health Research (NOM-062-Z00-1999). All procedures were approved by an ethics committee. Male adult CD1 mice weighing 25–35 g were used (obtained from the Animal Care Unit from Facultad de Medicina, UNAM).
Animals were distributed among groups according to a balanced design based on body weight (3 animals per group in each experiment). Room temperature was kept constant (21–24 °C), and with light–dark cycles of 12 h. Food and water were given ad libitum. Persenone-A was dissolved in DMSO (vehicle) and administered intraperitoneally (i.p.). Control animals only received the vehicle (1.66 mL kg−1).
Blood clotting time.
Groups of animals received a single i.p. administration of Persenone A (1, 10 or 100 mg kg−1 of body weight, respectively) or vehicle. Blood clotting time was measured 24 h after administration, as previously described.16 The tail of the animal was warmed in a water bath at 37 °C for 3 min. The tail was dried and transected at 8 mm from the tip with a scalpel. Briefly, a 25 μL blood sample was collected from the bleeding tail tip into a microhematocrit glass capillary tube. The capillary tube was alternatively tilted to angles of +60° and −60° with respect to the horizontal plane, allowing blood to flow by gravity between two marks, separated by 45 mm. Time was counted from the instant that blood first made contact with the glass capillary tube and until the blood ceased to flow. The blood clotting time data were presented as the relative increase elicited by Persenone A as a percentage of that obtained in samples treated with the vehicle.
Experimental thrombosis model.
Groups of animals received a single i.p. administration of Persenone A (25 mg kg−1 of body weight) or vehicle. After 24 h of treatment, mice were anesthetized with phenobarbital (80 mg kg−1). Thrombosis was induced by a surgical model by tightening two sutures separated by 1 cm for 1 h to cause vascular occlusion in the right leg femoral vasculature. The vascular segments were then removed, fixed in formalin, dehydrated and embedded in paraffin. 4 μm thick sections were stained with Masson's trichrome and hematoxylin-eosin, and scored by a pathologist.
Statistical analysis
Data are representative of at least three independent experiments. Results were expressed as means ± SEM. Statistically significant differences among groups were analyzed employing one-way analysis of variance (ANOVA), and differences between the control and the treated group were estimated by Dunnett's or LSMean Student's tests as appropriate. Differences were considered significant at a level of P < 0.05. Half-maximal inhibitory concentrations (IC50, μM) of platelet aggregation and half-maximal effective concentrations (EC50, mg kg−1 body weight) of blood clotting times were determined by nonlinear regression analysis using a sigmoidal concentration–response equation. Statistical calculations were performed using the GraphPad Prism software, version 5.0 (GraphPad Software, San Diego, CA, USA).
Results and discussion
Preliminary activity screening
A previous study evaluated the inclusion of avocado pulp as a fat source (2.75%) in the diet of Type 2 diabetic patients (avocado cultivar was not reported). Diets were administered during a four week period, and were formulated to provide adequate energy supplies that came from carbohydrates (50%), fats (30%) and proteins (20%).10 Authors reported that patients consuming avocado showed significantly lower levels of platelet aggregation (∼30%) when compared with patients who did not consume avocado. Based on these prior observations, and preliminary tests with avocado pulp crude extracts, the present screening study was undertaken with the purpose of separating molecules present in crude extracts. Fractionation was conducted based on the hypothesis that compounds called acetogenins, present in avocado pulp and seeds, could potentially exhibit antiplatelet aggregation activities, based on their chemical structure. To test the hypothesis, acetone soluble compounds were extracted from avocado seeds, dried, and fractionated using CPC. Fractions with similar HPLC-PDA chromatographic profiles (at 220 nm) were grouped together, as previously described.11 Seven different avocado pulp sub-fractions, enriched in acetogenins, were obtained and designated as GF01 to GF07. Calculated KD values ranged from 0.14–0.40, 0.83–1.13, 1.59–1.72, 2.03–2.41, 4.12–5.82, 7.37–11.67 and 14–∞, for fractions GF01 to GF07, respectively. Potential inhibitory effects on platelet aggregation, for fractions GF01 to GF07 (at 500 μg solid dry-weight (dw) mL−1), were measured turbidimetrically on platelets induced by ADP. As shown in Fig. 2, fractions GF03 to GF07 significantly inhibited (P < 0.01) platelet aggregation in reference to the control vehicle. However, GF03 exhibited lower inhibition values (38 ± 4.15%) than the rest of the fractions, which resulted in levels of over 75% inhibition.
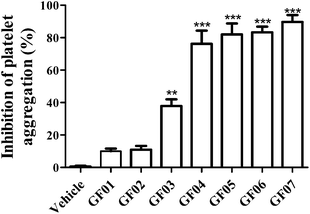 |
| Fig. 2 Percent inhibition of ADP-induced (20 μM) platelet aggregation exhibited by the groups of avocado pulp fractions GF01 to GF07 (500 μg solid dry weight mL−1), obtained after partition and centrifugal partition chromatography purification of acetone soluble solids from avocado pulp. The acetogenin content in each pulp fraction has been reported in Rodriguez-Sanchez et al. 2013.11 Each point represents the mean ± S.E.M. (n = 4). **P < 0.01, ***P < 0.001 compared with the vehicle control (one-way ANOVA with Dunnett-corrected post hoc analyses). | |
In the presence of equal concentrations of solids from each fraction (500 μg solid dw mL−1), platelet viability was found to be >80%, for fractions GF02 to GF07 (data not shown), suggesting that platelet integrity appeared to be not affected by compounds present in those fractions at concentrations evaluated in the platelet aggregation assay. However, compounds present in fraction GF01 appeared to have a negative effect on platelet function resulting in 62.5 ± 9.68% viability. Based on the higher activity and minimal effects on platelet viability, G004 to G007 were further characterized to determine the nature of the phytochemicals therein contained.
Mass spectrometry analysis of chromatographic peaks that were contained in the four fractions (GF04 to GF07), which presented the highest platelet aggregation inhibitory effects, consistently presented a similar ion pattern of [M + Na]+ and [M + H]+ molecular ions. In addition, fragment ions showing successive losses of H2O and/or acetic acid (C2H4O2) from the [M + H]+ ion were also present. This pattern was in accordance with the characteristic ion pattern reported for acetogenins,17 and more specifically for acetylated acetogenins derived from avocado fruit.18,19 With that information it was possible to assign the chemical identity to the chromatographic peaks by comparison of their mass spectra with values reported in the literature, and with data from standards isolated in our laboratory from avocado seeds.13 As shown in Table 1, compounds were identified as: 1-acetoxy-2,4-dihydroxy-n-heptadeca-16-ene (1);18 Persediene (2);13 Persenone-C (3);13 Persenone-A (4);18 Persenone-B (5),20 Persin (6)21 and 1-acetoxy-2,4-dihydroxy-heneicosa-12,15-diene (7).19 Data from the preliminary screening study suggest, for the first time, the inhibition of platelet aggregation in the presence of fractions containing acetogenins obtained from avocado pulp.
Table 1 Chemical identity of the common acetogenins present in the groups of fractions with the highest antiplatelet activity (GF04 to GF07)
Compound |
[M + H]+ (m/z)a |
Ions pattern (m/z)a |
Structure |
References |
MS/TOF detection using the electrospray ionization interface in positive-ion mode of analysis.
|
(2S,4S)-1-Acetoxy-2,4-di-hydroxy-n-heptadeca-16-ene (1) |
329 |
351, 311, 269, 251 |
|
18
|
Persediene (2) |
353 |
375, 335, 293 |
|
13
|
Persenone-C (3) |
353 |
375, 335, 293 |
|
13
|
Persenone-A (4) |
379 |
401, 361, 319, 301 |
|
18
|
Persenone-B (5) |
355 |
377, 337, 295 |
|
20
|
Persin (6) |
381 |
403, 363, 321, 303 |
|
21
|
(12Z,15Z)-1-Acetoxy-2,4-dihydroxy-heneicosa-12,15-diene (7) |
383 |
365, 323, 305 |
|
19
|
Isolation and purification of avocado pulp acetogenins
In order to further validate the potential antiplatelet activity observed for fractions that contained acetogenins, a large scale isolation and purification process was developed (Fig. 1). Through this process it was possible to recover larger amounts of purified compounds (4–130 mg, depending on their naturally occurring concentration) and to conduct the subsequent studies, which included the evaluation of the dose–response relationship of purified molecules in platelet aggregation induced by different agonist and in vivo studies.
Primary extraction was then optimized to increase yields. For this task, a new acetone crude extract E001 was prepared and partitioned in a biphasic system comprised of heptane–methanol. Heptane and methanol phases were separated and washed with methanol or heptane, respectively. Phases were separated and concentrated, yielding heptane- and methanol-soluble semicrude subfractions: E004 and E005 (derived from E002), and E006 and E007 (resulting from E003), respectively. HPLC-PDA analysis of these subfractions indicated that acetogenins were preferentially concentrated in E005 and E006 (data not shown).
Consequently, they were mixed and subjected to sequential CPC-fractionation. For that purpose, based on CPC principles,12 an appropriate solvent system was selected to ensure correct separation of compounds; considering that extracts E005 and E006 contained less impurities than the sample chromatographed in a preliminary evaluation (Isolation of semi-purified fractions for preliminary activity screening in Materials and methods), the same system used back then would not perform the same for this new sample. Mass spectra from CPC fractions were obtained (every 10 fractions), and used as a tool to track the location of compounds 1 to 7 and selectively isolate them from the fractions in which they were preferably enriched (Fig. 3), which corresponded to fractions 74–120 with KD values ranging from 0.90 to 1.52. Based on their higher relative concentrations, compounds 1 to 4 and 6 were then selected for further purification by preparative and semi-preparative HPLC sequential runs.
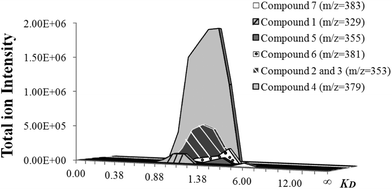 |
| Fig. 3 Total ion intensity values obtained by HPLC-MS analysis of different avocado pulp fractions isolated from an acetogenin-enriched extract. Information was used to track their location, and selectively isolate them from the fractions in which they were preferably enriched (KD = 0.90–1.52, corresponding to fractions 74–120). | |
Characterization of platelet aggregation inhibitory properties of purified acetogenins
Platelets normally circulate in a resting state and upon vascular injury they interact with components of the sub-endothelial matrix, particularly collagen and von Willebrand factor (vWF), via their respective receptors glycoprotein (GP) VI and GPIb/V/IX. Agonists, such as ADP, epinephrine, thromboxane A2 (arachidonic acid-derived) and thrombin, are then released or produced to further amplify platelet activation by interacting with their respective membrane receptors. Hence, more circulating platelets from the blood flow are recruited to sustain hemostatic plug growth. The final pathway for all agonists is the activation of the platelet membrane GPIIb/IIIa integrin, leading to thrombus formation through fibrinogen bridges.22
The most common antiplatelet agents currently used in clinical practice for the prevention of atherothrombosis are aspirin and clopidogrel.2 Their mechanisms of action are focused on inhibiting irreversibly amplification mechanisms of platelet activation by blocking the interaction of an agonist with its specific cell surface receptor.23 For instance, aspirin, as an irreversible inhibitor of cyclooxygenase (COX)-1, prevents arachidonic acid from being metabolized to prostaglandins G2/H2 and subsequently inhibits thromboxane A2 formation,24 whereas clopidogrel directly interferes with ADP binding to its P2Y12 receptor.25 Therefore, to confirm acetogenin's contribution to antiplatelet activity, their individual activity was evaluated at different concentrations (0.15–15 mM). Aggregation was induced by different agonists that included collagen (5 μg mL−1), ADP (20 μM) and arachidonic acid (500 μM) in an approximation to explore the potential interference of acetogenins with the binding of a particular agonist to its cell surface receptor.
As shown in Table 2, Persenone-C (3) presented a significantly lower (P < 0.05) IC50 than the other compounds for collagen, ADP and arachidonic acid (3.42 ± 1.56, 5.22 ± 1.19, 7.40 ± 1.20 mM, respectively). Compound 1 also inhibited platelet aggregation induced by all three agonists, but at >1.5-fold higher concentrations than Persenone-C (3). Whereas Persenone-A (4) exhibited a similar IC50 to compound 1 against collagen-induced aggregation, but even at the highest concentrations (15 mM) tested it was unable to reach 50% inhibition when aggregation was induced by arachidonic acid. Isolated Persediene (2) and Persin (6) showed significantly lower platelet aggregation inhibition levels. Persediene (2) inhibited collagen-induced aggregation; however, Persin (6) did not inhibit platelet aggregation at any of the evaluated concentrations. Incubation of the platelets with purified compounds at 15 mM had no effect on the platelet viability (data not shown).
Table 2 Half-maximal inhibitory concentrations (IC50) presented by purified acetogenins in a platelet aggregation assay, induced by different agonists
Evaluated sample |
Half-maximal inhibition concentrations (IC50 (mM))a,b |
Collagen (5 μg mL−1) |
ADP (20 μM) |
Arachidonic acid (500 μM) |
Values represent mean ± standard deviation (n = 4).
“>15” means that the achieved inhibition was lower that 50%, at 15 mM.
Different letters in the same column indicate that values are significantly different (P < 0.05) by the LSMean Student's t-test.
|
(2S,4S)-1-Acetoxy-2,4-dihydroxy-n-heptadeca-16-ene (1) |
8.18 ± 1.48bcc |
7.29 ± 1.21b |
13.42 ± 1.26c |
Persediene (2) |
11.99 ± 1.24c |
>15 |
>15 |
Persenone-C (3) |
5.23 ± 1.19b |
3.42 ± 1.56a |
7.40 ± 1.20b |
Persenone-A (4) |
8.73 ± 1.25bc |
13.48 ± 1.41c |
>15 |
Persin (6) |
>15 |
>15 |
>15 |
Aspirin (acetylsalicylic acid) |
0.38 ± 0.07a |
3.65 ± 0.07a |
0.07 ± 0.01a |
In preliminary screening studies, described in Isolation and purification of avocado pulp acetogenins, fractions containing mixtures of compounds, evaluated at concentrations of 500 μg solid dw mL−1, presented inhibitory concentrations of greater than 80% (Fig. 2). However purified compounds presented higher IC50 values (3.42 mM = 1207 μg mL−1) than semi-pure fractions (Table 2). This suggests that isolation of compounds has a detrimental effect on antiplatelet activity, probably due to synergistic interactions between compounds in mixture,26 or that acetogenins are not the only ones responsible for the activity. However, this is the first evidence linking antiplatelet action, previously observed for avocado pulp, to acetogenins, of which Persenone-C (3) was the most potent.
As expected,23 aspirin markedly inhibited (IC50 = 0.07 ± 0.01 μM) arachidonic acid-induced platelet aggregation with IC50 values 5- and 50-fold lower than that observed for collagen- and ADP-induced aggregation, respectively. In contrast, purified acetogenins did not exhibit such an evident selective inhibition for any of the evaluated agonists. This observation suggests that acetogenins do not interfere with the binding of the evaluated agonists to their specific receptors on the platelet's surface.
The precise mechanism by which acetogenins trigger antiplatelet activity is not clear. However, they may contribute to an increase in the negativity of the platelet surface charge. In this context, it has been demonstrated that under normal conditions, when human platelets circulate in a resting state, they carry a net negative surface charge, as determined by their electrophoretic mobility.27 This charge is mainly attributed to the presence of sialic acid, a negatively charged nine-carbon sugar,28 attached to proteins and lipids in their outer membranes.29 The repulsive force of the same negative charged platelets keeps them apart, inhibiting their aggregation.30 Studies have reported that platelets from patients with coronary heart disease present a significantly lower (P < 0.05) sialic acid content compared to controls, resulting in a lower negative surface charge and higher propensity to aggregate.29
Upon activation, platelets undergo drastic morphological changes, becoming flatter. Their granules are gathered into the center and the cytoskeleton is rearranged to develop filopods.22 Additionally, flippase and scramblase are activated, and the location of negatively charged phospholipids, mainly phosphatidylserine, changes from the inner to the outer membrane surface, inducing coagulation.31 Besides structural changes, agonist compounds of dense and α-granules are secreted facilitating the recruitment of additional platelets and thrombus stabilization.3 Cytosolic and surface calcium concentration increases as a result of its influx from the extracellular milieu, and its release from the dense tubular, becoming an important second messenger in the platelet activation cascade.32 Ultimately a net positive charge is developed.27,30 The mechanism of the surface charge reversal is not known.27 However it has been demonstrated that platelet activation occurs when the negative surface charge of resting platelets is neutralized by different means, for example, by the addition of cationic molecules33,34 or when they come in contact with positively charged biomaterials’ surfaces.30
A recent report showed that increasing oral doses of omega-3 polyunsaturated fatty acids (PUFAS, from 1 to 8 g daily over 24 weeks) in human subjects produced a significant increase (P < 0.05) in the negative surface charge of resting platelets, compared to the baseline.27 Additionally, an attenuated response to arachidonic acid-induced platelet aggregation was observed. Based on their findings, researchers suggest that the increased negativity of the platelet surface charge produced by PUFAS may create an additional hurdle that must be overcome before platelets can become fully activated. Thus, the structural similarities between PUFAS and avocado acetogenins18,35 suggest a similar mechanism, since acetogenins are lipophilic compounds able to accumulate in cell membranes36 and it has been proposed that they can exist as anionic species, due to their activity as mitochondrial uncoupling agents.36,37 Delocalization of the charge over its structure, facilitated by the presence of a keto group at C-4,37 conjugated to an unsaturation at C5–C6,36 enables the loss of protons.
In addition, it has been observed that diets with a high content of omega-3 PUFAS, especially eicosapentaenoic acid (EPA) and docosahexaenoic acid (DHA), are associated with a lower rate of cardiovascular events.38 These fatty acids have shown positive effects on platelet function,39 which have been associated with the metabolic end products derived from them.40 For instance, arachidonic acid (omega-6 PUFA), hydrolyzed from inner membrane phospholipids by phospholipase A2, is further metabolized to thromboxane A2, a potent platelet agonist and vasoconstrictor.41,42 In contrast, omega-3 PUFA are metabolized to thromboxane A3, considered as a poor platelet agonist and vasoconstrictor.40 Furthermore, there is a novel family of lipid local mediators (lipoxins, resolvins, protectins, and maresins) generated from omega-3 PUFAS by the action of lipoxygenases and cyclooxygenases.43 These lipid mediators have recently been associated with resolution of vascular inflammation43,44 and inhibition of platelet aggregation,45 both relevant in pathological cardiovascular events.
To our knowledge, no information is available regarding acetogenin metabolism in humans that may be used for comparison to PUFAS-derived metabolic products with a protective effect on platelet aggregation. However, it has been reported that Persin (6) can be metabolized by lipoxygenases, causing a loss of its antifungal activity.46 In our results, it can be observed that among the evaluated acetogenins (compounds 1–4 and 6), Persediene (2) and Persenone-C (3) present an unsaturation at C3–C4 from the methyl end, similarly to omega-3 PUFA (Table 1), but compound 2 additionally presents a terminal vinyl group. Interestingly, Persenone-C (3) exhibited the highest antiplatelet activity (Table 2), suggesting that omega-3 unsaturation may be another relevant structural feature for this effect. For instance, compounds Persenone-C (3) and Persenone-A (4) contain the enone group, previously proposed as an important feature to form stabilized anions that increase the negativity of the platelet surface, but the lack of omega-3 unsaturation in Persenone-A seems to reduce its antiplatelet activity compared to compound 3.
The case of compound 1 is unique, because it does not present any of the mentioned structural features, although it performs somewhat better than Persenone-A. Prior reports confirmed that compound 1, among other acetogenins extracted from avocado, reduced inflammation in skin cells and UV-induced damage, as it increased cell viability, decreased the secretion of interleukin-6 and prostaglandin E2 (PGE2), and enhanced DNA repair.47 Furthermore, it was suggested that reduced secretion of PGE2 in keratinocytes was associated with inhibition of phospholipase A2 activity in skin cells.48 This may suggest that the mechanism through which compound 1 attenuates platelet aggregation can be related to lower hydrolysis of arachidonic acid from the inner membrane by inhibiting phospholipase A2 action. However the proposed mechanisms need to be proved.
In vivo assay
Blood clotting time and thrombosis model.
In vitro methods used to measure platelet function detect a simultaneous aggregation of most of the platelets in the sample under low shear conditions.23 Although they represent very valuable tools to measure specific platelet functions and their modification by antiplatelet drugs, these methods do not reflect the physiological processes involved in the formation of a platelet-rich thrombus.23
Then, trying to confirm if the observed antiplatelet activity in vitro was reflected in vivo, blood clotting times of male mice were evaluated 24 h after i.p. administration of Persenone-A (4) (1, 10 and 100 mg kg−1 of body weight). Persenone-A was not the most potent antiplatelet acetogenin, but it was selected for in vivo evaluation because of its higher recovered yields. Considerations to determine the maximum explored dose were based on the few reports available evaluating the in vivo effect of acetogenins. There is one study that documented the protective effect of Persenone-A (4) and Persin (6), at doses of 100 mg kg−1, on rat liver injury induced by D-galactosamine.21 Another study suggested that Persin (6) produced necrosis of the secretory epithelium of the mammary gland in lactating mice at 60–100 mg kg−1, whereas greater doses additionally produced necrosis of myocardial fibers.49
Our findings indicate that at 100 mg kg−1, Persenone-A (4) produced a 2-fold increase in blood clotting time. The calculated EC50 value for Persenone-A (4) was 24.8 ± 1.7 mg kg−1 of body weight (Fig. 4).
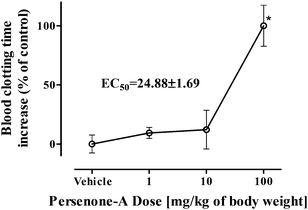 |
| Fig. 4 Dose–response relationships of Persenone-A (1–100 mg kg−1 of body weight) on the blood clotting time of male mice, 24 h after single i.p. administration. Data were expressed as percentage increase from that obtained with the vehicle. Each point represents the mean ± S.E.M. (n = 4). *P < 0.05 compared with the vehicle control (one-way ANOVA with Dunnett-corrected post hoc analyses). N = 3 for each treatment. | |
Blood coagulation is not only the result of interactions between platelets, but also it is a complex process; it is a highly regulated process involving plasma coagulation factors and the vessel wall.50 It was confirmed that the antiplatelet activity of acetogenins initially observed in vitro was successfully reflected in in vivo models.
Finally, mice were subjected to an acute ischemic challenge, 24 h after i.p. administration of Persenone-A (4), at a dose of 25 mg kg−1 of body weight, based on its EC50 value on the blood clotting time increase. With respect to vehicle (Fig. 5), mice treated with compound 4 (Fig. 5C) decrease by 71% in the presence of a thrombus. This provides more evidence that the antiplatelet activity of acetogenins observed in in vitro assays was positively reflected in animals, ultimately strongly suggesting an efficient antithrombotic effect. However, these interesting results should be explored in more detail in a further report.
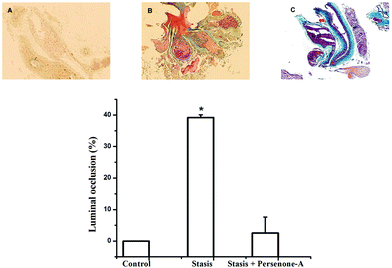 |
| Fig. 5 Persenone A (compound 4) decreases thrombus induction in male mice. Upper panel: a transverse section of the femoral vasculonervous package stained with Masson's trichrome. (A) The femoral artery does not present thrombi. Numerous unorganized erythrocytes can be observed in the light. (B) Surgical induction of the thrombus. A recent, unorganized thrombus can be observed in the artery. Fibrin accompanies the first step in thrombus organization, which occludes 40% of the vascular light. (C) A single i.p. administration of Persenone-A (25 mg kg−1) dissolved in the DMSO vehicle 24 h before the surgical induction reduces the thrombus formation. In this representative image 10% of the vascular light is occluded. Bottom panel: percentage analysis of thrombus induction. *P < 0.05 compared with the vehicle control. N = 4 for each treatment. | |
No studies have been performed on the bioavailability of Persenones (in vitro or in vivo), which if available could provide some information on the amounts of avocado pulp that a human would need to consume in order to obtain specific levels of these bioactive lipids in the bloodstream. However, studies on the concentrations of Persenones in avocado pulp (cv. Hass) have indicated that it contains approx. 2600 ppm of Persenones (A, B and C) and a concentration of total acetogenins of approx. 2800 ppm (data not shown), values that are ∼110-fold higher than the concentrations found to be active in the vascular occlusion study. Although acetogenins are lipid derivatives and can possibly be absorbed in a similar manner to other fatty acids such as EPA and DHA, further work needs to be done on their absorption and metabolism in order to extrapolate the bioactivities observed herein to the human metabolism.
Conclusion
In conclusion, a bioassay-guided isolation and purification process, based on in vitro antiplatelet properties, led to the identification of seven acetogenins as constituents of the active fractions. Characterization of antiplatelet activities on purified compounds showed the inhibitory properties of Persenone-C (3) (IC50 = 3.42–7.40 mM). Acetogenins did not interfere with the binding of ADP, arachidonic acid or collagen to their specific receptors on the platelet's surface. Therefore their contribution to increase the negativity of the platelet surface is suggested as a possible mechanism of action that needs to be explored. Additionally it was observed that the most potent acetogenin exhibited an omega-3 unsaturation, similarly to omega-3 PUFAS which are recognized by their cardioprotective effect. The performance of Persenone-A (4), another active acetogenin, was evaluated in vivo, suggesting that it may produce protective effects against arterial thrombosis.
Acknowledgements
The authors thank the Hospital San José Department of Pathology for thrombus description, especially thanks are due to Dr Irma Eraña. This research was possible thanks to the research funds from the CONACYT-TAMU Research Initiative, the Tecnológico de Monterrey-Research Chair Initiatives on Cardiology (CAT-131) and Micronutrientes (CAT-198), as well as CONACYT-México grants 133591 (G. García-Rivas), Xignus Research grant (CIE122) PROEZA Research Grant (CIE151) and CONACYT doctoral scholarship number 228072 (Dariana G. Rodriguez-Sanchez). We would also like to thank Avomex Intl., S.A. de C.V. (Sabinas, Coahuila, México) for providing avocado fruits.
Notes and references
- [OMS], O.M.d.1.S. Cardiovascular diseases, 2011 [cited 2011 May]; Available from: http://www.who.int/cardiovascular_diseases/en/.
- A. D. Tselepis, G. Gerotziafas, G. Andrikopoulos, H. Anninos and P. Vardas, Mechanisms of platelet activation and modification of response to antiplatelet agents, Hellenic J. Cardiol., 2011, 52(2), 28–40 Search PubMed.
- L. K. Jennings, Mechanisms of platelet activation: need for new strategies to protect against platelet-mediated atherothrombosis, Thromb. Haemost., 2009, 102(2), 248–257 CAS.
- S. P. Jackson, The growing complexity of platelet aggregation, Blood, 2007, 109(12), 5087–5095 CrossRef CAS PubMed.
- J. M. Siller-Matula, J. Krumphuber and B. Jilma, Pharmacokinetic, pharmacodynamic and clinical profile of novel antiplatelet drugs targeting vascular diseases, Br. J. Pharmacol., 2010, 159(3), 502–517 CrossRef CAS PubMed.
- S. Johnson, Known knowns and know unknowns: risk associated with combination antithrombotic therapy, Thromb. Res., 2008, 123(Suppl 1), S7–11 CrossRef CAS PubMed.
- Z. Pieterse, J. C. Jerling, W. Oosthuizen, H. S. Kruger, S. M. Hanekom, C. M. Smuts and A. E. Schutte, Substitution of high monounsatura fatty acid avocado for mixed dietary fats during and energy-restricted diet: effects on weight loss, serum lipids, fibrinogen, and vascular function, Nutrition, 2005, 21(1), 67–75 CrossRef CAS PubMed.
- C. L. Pelkman, V. K. Fishell, D. H. Maddox, T. A. Pearson, D. T. Mauger and P. M. Kris-Etherton, Effects of moderate-fat (from monounsaturated fat) and low-fat weight-loss diets on the serum lipid profile in overweight and obese men and women, Am. J. Clin. Nutr., 2004, 79(2), 204–212 CAS.
- K. Duester, Avocado fruits is a rich source of beta-sitosterol, The food consult, J. Am. Diet. Assoc., 2001, 101(4), 404–405 CrossRef CAS.
- M. J. Carranza, M. M. Alvizouri, J. E. Herrera and F. Chávez, Efectos del aguacate como fuente de ácidos grasos monoinsaturados en lípidos séricos, metabolismo de la glucosa y reología en pacientes con diabetes tipo 2, Med. Int. Mex., 2008, 24(4), 267–272 Search PubMed.
- D. G. Rodriguez-Sanchez, C. Silva-Platas, R. P. Rojo, N. García, L. Cisneros-Zevallos, G. García-Rivas and C. Hernández-Brenes, Activity-guided identification of acetogenins as novel lipophilic antioxidants present in avocado pulp (Persea americana), J. Chromatogr., B: Biomed. Appl., 2013, 942–943, 37–45 CrossRef CAS PubMed.
- A. Berthod, J. B. Friesen, T. Inui and G. F. Pauli, Elution-extrusion countercurrent chromatography: theory and concepts in metabolic analysis, Anal. Chem., 2007, 79(9), 3371–3382 CrossRef CAS PubMed.
- D. G. Rodriguez-Sanchez, A. Pacheco, M. I. García-Cruz, J. A. Gutiérrez-Uribe, J. A. Benavides-Lozano and C. Hernández-Brenes, Isolation and structure elucidation of avocado seed (Persea americana) lipid derivatives that inhibit Clostridium sporogenes endospore germination, J. Agric. Food Chem., 2013, 61(30), 7403–7411 CrossRef CAS PubMed.
- A. De la Peña, G. Baños, R. Izaguirre, J. J. Mandoki and J. M. Fernández, Comparative effect of synthetic aminoestrogens with estradiol on platelet aggregation, Steroids, 1993, 58(9), 407–409 CrossRef.
- S. S. Falkenberg, I. Tarnow, A. Guzmán, P. Molgaard and H. T. Simonsen, Mapuche herbal medicine inhibits blood platelet aggregation, Evid. Based Complement. Alternat. Med., 2012, 2012, 647620 Search PubMed.
- R. Jaimez, A. Cooney, K. Jackson, A. E. Lemus, C. Lemini, M. Cárdenas, R. García, G. Silva and F. Larrea, In vivo estrogen bioactivities and in vitro estrogen receptor binding and transcriptional activities of anticoagulant synthetic 17beta-aminoestrogens, J. Steroid Biochem. Mol. Biol., 2000, 73(1–2), 59–66 CrossRef CAS.
- Z. M. Gu, D. Zhou, J. Wu, G. Shi, L. Zeng and J. L. McLaughlin, Screening for Annonaceous
acetogenins in bioactive plant extracts by liquid chromatography/mass spectrometry, J. Nat. Prod., 1997, 60(3), 242–248 CrossRef CAS PubMed.
- F. Domergue, G. L. Helms, D. Prusky and J. Browse, Antifungal compounds from idioblast cells isolated from avocado fruits, Phytochemistry, 2000, 54(2), 183–189 CrossRef CAS.
- A. G. Degenhardt and T. Hofmann, Britter-tasting and kokumi-enchancing molecules in thermally processed avocado (Persea americana Mill.), J. Agric. Food Chem., 2010, 58(24), 12906–12915 CrossRef CAS PubMed.
- O. K. Kim, A. Murakami, Y. Nakamura, N. Takeda, H. Yoshizumi and H. Ohigashi, Novel nitric oxide and superoxide generation inhibitors, persenone A and B, from avocado fruit, J. Agric. Food Chem., 2000, 48(5), 1557–1563 CrossRef CAS PubMed.
- H. Kawagishi, Y. Fukumoto, M. Hatakeyama, P. He, H. Arimoto, T. Matsuzawa, Y. Arimoto, H. Suganuma, T. Inakuma and K. Sugiyama, Liver injury suppressing compounds from avocado (Persea americana), J. Agric. Food Chem., 2001, 49(5), 2215–2221 CrossRef CAS PubMed.
- Z. Li, M. K. Delaney, K. A. O'Brien and X. Du, Signaling during platelet adhesion and activation, Arterioscler. Thromb. Vasc. Biol., 2010, 30(12), 2341–2349 CrossRef CAS PubMed.
- A. A. Weber, M. Adamzik, H. S. Bachmann, K. Gorlinger, M. Grandoch, K. Leineweber, H. Muller-Beissenhirtz, F. Wenzel and C. Naber, Methods to evaluate the pharmacology of oral antiplatelet drugs, Herz, 2008, 33(4), 287–296 CrossRef PubMed.
- E. H. Awtry and J. Loscalzo, Aspirin, Circulation, 2000, 101(10), 1206–1218 CrossRef CAS.
- D. J. Angiolillo and J. L. Ferreiro, Platelet adenosine diphosphate P2Y12 receptor antagonism: benefits and limitations of current treatment strategies and future directions, Rev. Esp. Cardiol., 2010, 63(1), 60–76 CrossRef.
- I. Raskin, D. M. Ribnicky, S. Komarnytsky, N. Ilic, A. Poulev, N. Borisjuk, A. Brinker, D. A. Moreno, C. Ripoll, N. Yakoby, J. M. O'Neal, T. Cornwell, I. Pastor and B. Fridlender, Plants and human health in the twenty-first century, Trends Biotechnol., 2002, 20(12), 522–531 CrossRef CAS.
- M. G. Cohen, J. S. Rossi, J. Garbarino, R. Bowling, A. A. Motsinger-Reif, C. Schuler, A. G. Dupont and D. Gabriel, Insights into the inhibition of platelet activation by omega-3 polyunsaturated fatty acids: beyond aspirin and clopidogrel, Thromb. Res., 2011, 128(4), 335–340 CrossRef CAS PubMed.
- X. Chen and A. Varki, Advances in the biology and chemistry of sialic acids, ACS Chem. Biol., 2010, 5(2), 163–176 CrossRef CAS PubMed.
- R. Mandic, C. Opper, J. Krappe and W. Wesemann, Platelet sialic acid as a potential pathogenic factor in coronary heart disease, Thromb. Res., 2002, 106(2), 137–141 CrossRef CAS.
- V. Karagkiozaki, S. Logothetidis, S. Lousinian and G. Giannoglou, Impact of surface electric properties of carbon-based thin films on platelets activation for nano-medical and nano-sensing applications, Int. J. Nanomedicine, 2008, 3(4), 461–469 CAS.
- J. W. Heemskerk, W. M. Vuist, M. A. Feijge, C. P. Reutelingsperger and T. Lindhout, Collagen but not fibrinogen surfaces induce bleb formation, exposure of phosphatidylserine, and procoagulant activity of adherent platelets: evidence for regulation by protein tyrosine kinase-dependent Ca2+ responses, Blood, 1997, 90(7), 2615–2625 CAS.
- D. E. Roberts, A. McNicol and R. Bose, Mechanism of collagen activation in human platelets, J. Biol. Chem., 2004, 279(19), 19421–19430 CrossRef CAS PubMed.
- H. Yamazaki, H. Suzuki, N. Yamamoto and K. Tanoue, Electron microscopic observations on platelet aggregation induced by cationized ferritin, Blood, 1984, 63(2), 439–447 CAS.
- B. S. Coller, The effects of ristocetin and von Willebrand factor on platelet electrophoretic mobility, J. Clin. Invest., 1978, 61(5), 1168–1175 CrossRef CAS PubMed.
- C. Rodriguez-Saona and J. T. Trumble, Biologically active aliphatic acetogenins from specialized idioblast oil cells, Curr. Org. Chem., 2000, 4(12), 1249–1260 CrossRef CAS.
- C. Silva-Platas, N. García, E. Fernández-Sada, D. Dávila, C. Hernández-Brenes, D. Rodríguez and G. García-Rivas, Cardiotoxicity of acetogenins from Persea americana occurs through the mitochondrial permeability transition pore and caspase-dependent apoptosis pathways, J. Bioenerg. Biomembr., 2012, 44(4), 461–471 CrossRef CAS PubMed.
- A. M. Mendoza-Wilson, Computational study of the molecular structure and reactive sites of the R and S isomers of persin diene, J. Mol. Struct., 2008, 869, 67–74 CrossRef CAS PubMed.
- M. de Lorgeril and P. Salen, Dietary prevention of coronary heart disease: focus on omega-6/omega-3 essential fatty acid balance, World Rev. Nutr. Diet., 2003, 92, 57–73 CAS.
- S. D. Kristensen, E. B. Schmidt and J. Dyerberg, Dietary supplementation with n-3 polyunsaturated fatty acids and human platelet function: a review with particular emphasis on implications for cardiovascular disease, J. Intern. Med. Suppl., 1989, 731, 141–150 CrossRef CAS PubMed.
- H. J. Kramer, J. Stevens, F. Grimminger and W. Seeger, Fish oil fatty acids and human platelets: dose-dependent decrease in dienoic and increase in trienoic thromboxane generation, Biochem. Pharmacol., 1996, 52(8), 1211–1217 CrossRef CAS.
- K. Yuhki, F. Kojima, H. Kashiwagi, J. Kawabe, T. Fujino, S. Narumiya and F. Ushikubi, Roles of prostanoids in the pathogenesis of cardiovascular diseases, Int. Angiol., 2010, 29(2 Suppl), 19–27 CAS.
- W. Auch-Schwelk, Z. S. Katusic and P. M. Vanhoutte, Thromboxane A2 receptor antagonists inhibit endothelium-dependent contractions, Hypertension, 1990, 15(6 Pt 2), 699–703 CrossRef CAS.
- G. Bannenberg and C. N. Serhan, Specialized pro-resolving lipid mediators in the inflammatory response: An update, Biochim. Biophys. Acta, 2010, 1801(12), 1260–1273 CrossRef CAS PubMed.
- S. Hong and Y. Lu, Omega-3 fatty acid-derived resolvins and protectins in inflammation resolution and leukocyte functions: targeting novel lipid mediator pathways in mitigation of acute kidney injury, Front Immunol., 2013, 4, 13 Search PubMed.
- G. Fredman, T. E. Van Dyke and C. N. Serhan, Resolvin E1 regulates adenosine diphosphate activation of human platelets, Arterioscler. Thromb. Vasc. Biol., 2010, 30(10), 2005–2013 CrossRef CAS PubMed.
- D. Prusky, N. T. Keen and I. Eaks, Further evidence for the involvement of preformed antifungal compounds in the latency of Colletotrichum gloeosporioides on unripe avocado fruits, Physiol. Plant. Pathol., 1983, 22, 189–198 CrossRef CAS.
- G. Rosenblat, S. Meretski, J. Segal, M. Tarshis, A. Schroeder, A. Zanin-Zhorov, G. Lion, A. Ingber and M. Hochberg, Polyhydroxylated fatty alcohols derived from avocado suppress inflammatory response and provide non-sunscreen protection against UV-induced damage in skin cells, Arch. Dermatol. Res., 2011, 303(4), 239–246 CrossRef CAS PubMed.
- M. F. Otuki, O. Schwob, P. A. Silveira, I. Zaltsman, S. Meretski, J. Segal, S. Yedgar and G. Rosenblat, Attenuation of experimental TPA-induced dermatitis by acetylenic acetogenins is associated with inhibition of PLA(2) activity, Eur. J. Pharmacol., 2011, 672(1–3), 175–179 CrossRef CAS PubMed.
- P. B. Oelrichs, J. C. Ng, A. A. Seawright, L. Schaffeler and J. K. MacLeod, Isolation and identification of a compound from avocado (Persea americana) leaves which causes necrosis of the acinar epithelium of the lactating mammary gland and the myocardium, Nat. Toxins, 1995, 3(5), 344–349 CrossRef CAS.
- D. J. Angiolillo, M. Ueno and S. Goto, Basic
principles of platelet biology and clinical implications, Circ. J., 2010, 74(4), 597–607 CrossRef CAS PubMed.
|
This journal is © The Royal Society of Chemistry 2015 |
Click here to see how this site uses Cookies. View our privacy policy here.