DOI:
10.1039/C4FO00831F
(Paper)
Food Funct., 2015,
6, 256-263
The probiotic Escherichia coli Nissle 1917 inhibits propagating colonic contractions in the rat isolated large intestine
Received
17th September 2014
, Accepted 13th November 2014
First published on 14th November 2014
Abstract
The objective of this research was to test an in vitro motility model by investigating whether a probiotic that reduces diarrhea in humans would reduce motility in the rat colon in vitro. The probiotic Escherichia coli Nissle 1917 (EcN) the active ingredient in Mutaflor® was used as an example probiotic because it is effective for treating infectious diarrheal diseases. The effect of EcN on motility was compared in two colonic preparations. In distal colon segments EcN extract decreased the tension of spontaneous contractions by 74% and frequency by 46% compared with pre-treatment controls. In the whole large intestine the number of synchronized spontaneous propagating contractions decreased by 86% when EcN extract was applied externally and 69% when applied via the lumen compared with pre-treatment. From the inhibition produced by EcN extract in the distal colon segment a myogenic action was inferred and in the whole large intestine neural involvement was implicated. Both are consistent with its anti-diarrheal effect in humans.
Introduction
There is growing interest from food companies in developing functional ingredients for improving gastro-intestinal (GI) health, particularly GI motility. GI motility refers to contraction and relaxation of the longitudinal and circular layers of intestinal wall smooth muscle that are essential for normal orderly propulsion of content along the intestine. The peristaltic wave is a propagating contraction that can occur in the stomach,1 small intestine or colon. Typically each discrete peristaltic contraction is preceded by a relaxation of the smooth muscle that propels contents aborally along the GI tract. Accumulating evidence suggests that the generation and/or propagation of peristalsis is controlled by a complex interplay between smooth muscle cells (myogenic) and enteric nerve cells (neurogenic) of the intestinal wall, or may involve pacemaker cells known as interstitial cells of Cajal (ICC). Although contractions are generally considered myogenic or neurogenic in origin, the spontaneous phasic propagating contractions characteristic of the colon are not preceded by relaxation and are generally considered to be neurogenic under normal circumstances in guinea pig, mouse, rabbit, and rat.2 The enteric nerves include sensory, motor, and inter-neurons that form the interconnected neuronal network of the enteric nervous system. These neurons control secretion of water, electrolytes, and mucus, together with the propulsive motor patterns that occur in the colon to facilitate absorption and stool formation that underlie normal bowel function.1
A majority of the bacteria present in the GI tract reside in the human colon, estimated at 1011 to 1012 bacterial cells per gram of colonic contents.3,4 An interplay among food, bacteria and the host affect GI motility, which in turn influences propulsion of bacteria and therefore impacts the microbial community.5 The influence of bacteria on motility to improve GI health is a growing area of research. Probiotic bacteria are efficacious in improving GI comfort and relieving constipation or diarrhea in clinical studies.6,7 For example: Lactobacillus casei Shirota reduces colonic transit time in female adults with chronic constipation8 and Bifidobacterium lactis HN019 reduces colonic transit time in adults.9Lactobacillus reuteri (DSM 17938) reduces the duration of diarrhea in children.10 The reduced motility caused by L. reuteri in vivo correlates with a decrease in motility in mouse jejunum ex-vivo.11 Similarly Lactobacillus paracasei (NCC2461) was the only probiotic (of four tested) that decreased jejeunal hypercontractility in an irritable bowel syndrome mouse model.12
New methods to determine the effect of a potential probiotic on colonic dysmotility would be advantageous in early screening for functionality. To establish an in vitro model of intestinal motility to examine the effects of probiotics that are predictive of changes in colonic transit, we sought a well studied probiotic with known effects on GI transit. We therefore chose Escherichia coli Nissle 1917 (EcN) because its ability to alter GI motility is well known from human and animal studies, yet few mechanistic studies have been reported in isolated GI tissue and how EcN alters motility is unknown. EcN is the active component in Mutaflor® that human clinical studies have shown to be effective in treating constipation and diarrhea, including that associated with irritable bowel syndrome.13,14 It also shortens the duration of diarrhea in infants and young children.15,16 EcN is a Gram-negative enterobacterium that has been used as a probiotic for over 80 years. It was first identified by Alfred Nissle in 1917 who discovered that it provided protection against infectious diarrhea.17 Although EcN was isolated from humans, many commercialised probiotics have been isolated from dairy sources.
To develop functional probiotic ingredients to treat conditions of altered intestinal motility, it is important to establish an in vitro assay to assist the selection process for candidate strains for in vivo studies. We used a rat model because motility patterns that propel contents are well described for this species.2,18 We tested the hypothesis that a probiotic that reduces diarrhea will also reduce propagating contractions in an isolated whole large intestine preparation in vitro. We chose EcN as the test probiotic because it is used for the treatment of infectious diarrheal diseases. Due to the many clinical studies demonstrating EcN effects in humans, data from our in vitro study will translate to humans. Furthermore, the mechanism(s) by which EcN alters GI motility to alter transit are unknown. Bacterial extract was used in this study as opposed to live bacteria because other in vitro studies have found effects on motility using bacterial extract from different sources. For example, the cell-free EcN bacterial extract,19 or the cell supernatant fraction from VSL#3 mixed bacterial cultures20 contain the active components that affect muscle contraction.
The aim of this research was to use EcN as an example probiotic and correlate its anti-diarrheal effect on motility with that observed in vitro. Since bacterial extracts have been reported to be the active component in affecting muscle contraction in vitro,19,20 we used EcN extract. We tested for effects of EcN on: (1) spontaneous muscle contractions using a short preparation consisting of a (1–2 cm length) section of rat distal colon, and (2) spontaneous propagating contractions in the isolated rat whole large intestine (22 cm length). The effect of EcN extract on contractile tension and frequency was measured for both colonic preparations. The pattern and velocity of propagating contractions were measured in the whole large intestine.
Materials and methods
Bacterial extract
E. coli Nissle 1917 bacterial strain (kindly supplied by the School of Biological Sciences, The University of Auckland) primary culture was inoculated from a secondary plate into 7 mL of Standard-I-Bouillon broth (Merck) and incubated at 37 °C in a shaking incubator with loose lid for 24 h. Two 150 mL secondary cultures were inoculated with 3 mL of primary broth and incubated for 16 h to an OD600 of 1.65, measured to ensure that they were in stationary phase. The bacterial cell culture was harvested and processed into extract, then used in the motility assays freshly each day. Bacterial cells were collected by centrifugation (10
845g for 15 min at 4 °C) and resuspended in 5 mL of de Jalon buffer (a non-phosphate buffered physiological salt solution, made up from analytical reagents). The bacterial cell pellet was washed twice in de Jalon buffer, resuspended in 5 mL and incubated on ice for 10 min. The mixture was then sonicated on ice using 20 s pulses with 30 s intervals, power level 2 at 40% duty for 10 min (Vibra-Cell, Sonics and Materials, Newtown, USA). The sonicated mixture was then centrifuged (10
845g for 30 min) to remove the cell debris and the resulting supernatant was ultra-centrifuged to remove any remaining cell membranes (300
000g for 2 h) and to prevent excessive frothing of the solution in the motility experiment phase. The final supernatant was a cell-free extract (EcN extract) that was adjusted to pH 7.4 (with 5 M sodium hydroxide) and diluted 1/10 dilution in Kreb's buffer (118 mM NaCl, 4.7 mM KCl, 1.2 mM KH2PO4, 1.2 mM MgSO4, 2.6 mM CaCl2, 25 mM NaHCO3, 11 mM glucose, pH 7.4) for use in the motility assays. Additionally, the EcN extract was tested for any remaining live bacteria by plating the supernatant onto the nutrient agar plates and incubated for 24 h at 37 °C in a 5% CO2 incubator.
Animals
This study was carried out in strict accordance with the recommendations of the New Zealand Animal Welfare Act 1999. The protocol was approved by the AgResearch Limited (Grasslands) Animal Ethics Committee (Ethics Approval no.: AE98). Male adult Sprague Dawley rats, 3–9 months of age, weighing 250–550 g were obtained from AgResearch Ruakura (Hamilton, NZ) for the purpose of tissue collection. The rats were housed under a 12 hour light/dark cycle, and fed Sharpes Diet 86 (Sharpes Stockfeeds Ltd, Carterton, New Zealand). Food and water were available ad libitum. Animals were euthanized by CO2 inhalation overdose.
Distal colon method
Methods were similar to those described previously for the distal colon.21,22 This study differs in that additional controls were tested to account for the buffer in which the extract was prepared and longer treatment exposure times were used. After euthanasia, a 4 cm piece of distal colon was removed from the rat, 1 cm distal to the striations of the mid colon. The tissue was placed in Kreb's buffer oxygenated with 95% O2/5% CO2. The preparation was divided into four pieces and each distal colon piece was mounted longitudinally on holders in an isolated tissue bath system (SI-MB4, SI-Heidelberg, World Precision Instruments, Sarasota, FL, USA), at 37 °C.
Tissues were suspended under 1 g of tension and equilibrated for one hour in Kreb's buffer during which time the bath solution was exchanged every 15 min. Controls for each preparation recorded the spontaneous muscle contractions prior to any treatments. These were recorded during the last 15 min of a two hour exposure to Kreb's buffer and compared with the response to 30 min exposure to EcN extract applied following the control. Muscle contraction data were measured using BAM21-E amplifiers integrated using Lab-Trax 4/24T hardware and acquired and analysed using Labscribe 2 software (iWorx Inc., Dover, NH, USA). To allow for recovery from the solution exchange, data from the last 10 min of a 15 min recording period were analysed and the mean for each parameter determined from the contractile responses recorded. Contractile tension or amplitude (g) was measured from the baseline to the maximum peak of the contractile response. Contractile frequency was measured as the number of contractions counted per min. To ensure the viability of the preparation the maximum tension response was compared to that for 1 μM acetylcholine, which binds to acetylcholine receptors to induce muscle contraction, by adding it for 5 min at the end of the experiment. Preparations that gave little or no response to acetylcholine were excluded.
In previous studies the media in which the bacteria had been grown was subsequently used in a muscle contractility assay. However, our preliminary experiments in the rat distal colon in vitro testing whether bacterial media could be applied to the muscle preparation found that spontaneous contractions were partially inhibited by a 1/10 dilution of bacterial media in Kreb's buffer used to maintain the tissue preparation (data not shown). We therefore used only a physiological salt buffer (de Jalon or Kreb's buffer), without any media, to generate the bacterial extract (section 2.1) and diluted this 1/10 into Kreb's buffer in the tissue bath, which did not affect contractions in controls.
Whole large intestine
The method for measuring whole large intestine contraction was similar to that used previously.22 This study differs in that longer treatment exposure times were used. After euthanasia, the colon was removed from the rat (22 cm in length, to fit into the perfusion bath) approximately 3–5 cm below the caecum. The tissue was placed in oxygenated Kreb's buffer in a perfusion bath (210 mL volume) and externally perfused at 10 mL min−1 at 35 ± 1 °C. The colon was gently flushed with Kreb's solution using a syringe to expel faecal pellets. A stainless steel rod (approximately 21 cm long and 4 mm diameter) was inserted through the lumen of the colon and the entire tissue then mounted in an organ bath (approximately 200 mL capacity) perfused at 20 mL min−1 with Kreb's buffer at 35 ± 1 °C. The lumen was also perfused with Kreb's buffer at 0.5–1 mL min−1 which was pumped aborally using a constant flow pump because this provided the pressure required to record consistent propagating contractions. Changes in circular muscle tension were recorded from four sites simultaneously along the length of large intestine, using four custom-made metal hooks anchored 3 cm from each end of the preparation and evenly spaced at approximately 4 cm intervals. These hooks were connected via silk thread to force transducers and contractions measured as described for the distal colon segments.
A spontaneous propagating contraction was defined as a spontaneous contraction that occurred at four recording sites, and was generated in the proximal colon and migrated aborally to the distal recording site. The contractions that propagated from the proximal colon to the distal colon were analyzed and statistically compared. The interval between first and fourth contractions (taken from the time between 50% peak amplitude, on the rising phase, of four successive contractions) was measured. Propagation velocities were calculated by taking the distance between these recording hooks and dividing by the time taken for each spontaneous propagating contraction. Modulators were applied to the external side of the preparation via the perfusion tube supplying the bath.
Statistical analysis
Distal colon segment.
Results are expressed as the mean ± SEM from a minimum of 12 preparations (isolated from ≥7 animals). Ratios for each time point were calculated by dividing through by the value for tension or frequency for the initial time point in 10% de Jalon buffer (prior to the addition of the treatment compound). Analysis of variance was carried out on tension and frequency as response variables and time as predictor variable, with rat age as covariate. Statistical differences were assessed using Fisher's protected Least Significant Different test. The analyses were carried out using GenStat version 15.1 (VSN International Limited, Hemel Hempstead, UK).
Whole large intestine.
Results are expressed as the mean ± SEM from 5 animals. Repeated Measures Mixed Models were used to analyse differences in the number and proportion of propagating contractions between control and treatments. A square-root transformation was used to meet the assumptions of normality for data distribution to compare changes in the proportion of forward or synchronized contractions, between control and treatments. A Linear Mixed Model was used to compare the percent change in tension between control and treatments. The analyses were carried out using GenStat version 16.2 (VSN International Limited, Hemel Hempstead, UK).
Results
Distal colon segment
Spontaneous contractions were measured in the rat distal colon in vitro and effects of EcN extract on contractile tension and frequency measured (Fig. 1). During the 60 min control period, regular spontaneous contractions occurred at a mean frequency of 0.6 ± 0.1 contractions per minute, with an average tension of 0.92 ± 0.25 g (n = 5). There was no difference in muscle contractile parameters between Kreb's buffer and Kreb's containing 10% background buffer from the bacterial extract (de Jalon buffer). To determine the application time for maximal effect, the EcN extract was applied at a 1/10 dilution for 30 min and the response monitored. Spontaneous contractile activity recorded from the isolated distal colon before and after addition of extract is shown in Fig. 1. The effect of extract treatment on spontaneous muscle contractions was measured relative to that in the last 10 min of the control recording (Kreb's containing 10% de Jalon buffer). A decrease in tension and frequency occurred after several minutes of exposure to EcN extract, which further reduced rapidly over 15 min of exposure to the extract. Because the 1/10 dilution was effective this was used in subsequent experiments.
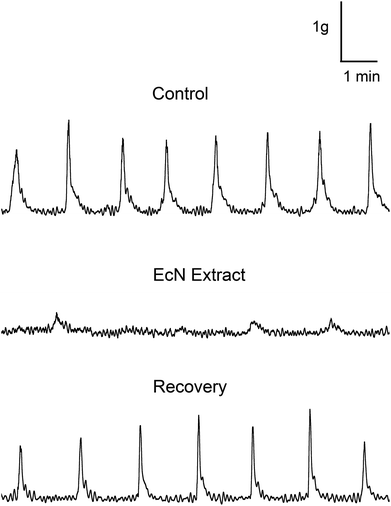 |
| Fig. 1 Effect of E. coli Nissle 1917 bacterial extract (EcN) extract on spontaneous muscle contraction in the short colon preparation. A representative example of a raw data recording shows changes in muscle tension over time for the control (last 10 min of 60 min Kreb's) and after addition of 1/10 EcN extract (last 10 min of 30 min exposure). | |
Data comparing the effect of the bacterial extract on contractile tension and frequency over time are shown in Fig. 2(a and b). Bacterial extract was applied to one preparation for 30 min, followed by 60 min in the control solution (de Jalon diluted 1/10 in Kreb's buffer). To account for variation in contractile activity among preparations for the pooled data, the contractile parameters were expressed as a proportion of the control measurement immediately prior to addition of bacterial extract. The bacterial extract reduced the peak tension of spontaneous muscle contractions by 74 ± 6% and frequency by 46 ± 16% (n = 12). Muscle contractions returned to levels not significantly different from pre-control levels after 60 min wash out in control buffer. Although the frequency appeared greater than the control, it was not significantly different. Furthermore, only preparations that gave robust contractile responses to the cholinergic activator acetylcholine chloride (1 μM) added at the end of the experiment were included in the analysis.
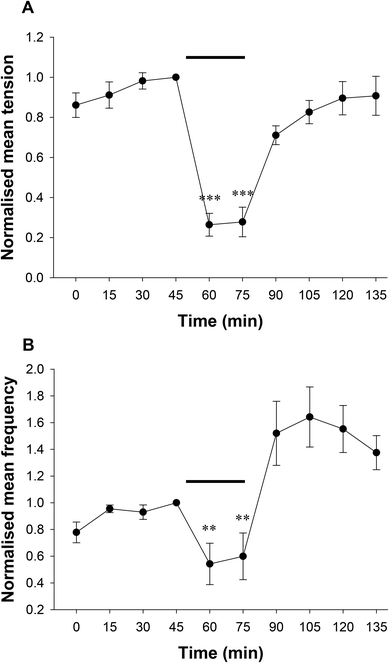 |
| Fig. 2 Changes in colonic motility over time are shown for spontaneous muscle contractions in Kreb's buffer. E. coli Nissle 1917 bacterial (EcN) extract in de Jalon buffer was applied at a 1/10 dilution to the bath for the period indicated by the bar. The control solution contained 1/10 de Jalon buffer. Contractile activity was normalised to the control recording immediately prior to addition of bacterial extract for (A) tension and (B) frequency; n = 12 preparations from 5 rats. Data show mean ± SEM. Asterisks indicate the significance of each treatment relative to the control at 45 min (*p < 0.05; **p < 0.01; ***p < 0.001). | |
Whole large intestine
Propagating contractions were recorded from the rat large intestine preparation in vitro before and after addition of EcN extract. A representative example is shown in Fig. 3. Effects on ability to propagate, direction of propagation, and contractile tension were measured. Control recordings had 4.2 ± 1.4 (n = 5) spontaneous forward propagating contractions from proximal colon to rectum during a 30 min period, after the preparation had been exposed to Kreb's buffer for 1–2 h. These forward propagating contractions had a velocity of 6.9 ± 0.9 mm s−1 (n = 5) and traversed a distance of 13.7 ± 1.1 cm (n = 5). Contractions that were temporally coordinated and were propagated either distally (forward), outward from the mid region of colon (mid), or orally (reverse) in direction were referred to as ‘synchronous contractions’. These were measured as a proportion of all contractions that were initiated at the proximal end. Because these synchronous contractions were more prevalent than forward contractions (8.6 ± 1.1 per 30 min, n = 5), and are all considered to be important as coordinated motor patterns for controlled propulsion and mixing, they were used to measure differences between control and EcN extract treatments. Synchronous propagating contractions had an interval between contractions of 3
:
51 ± 0
:
30 (min
:
s).
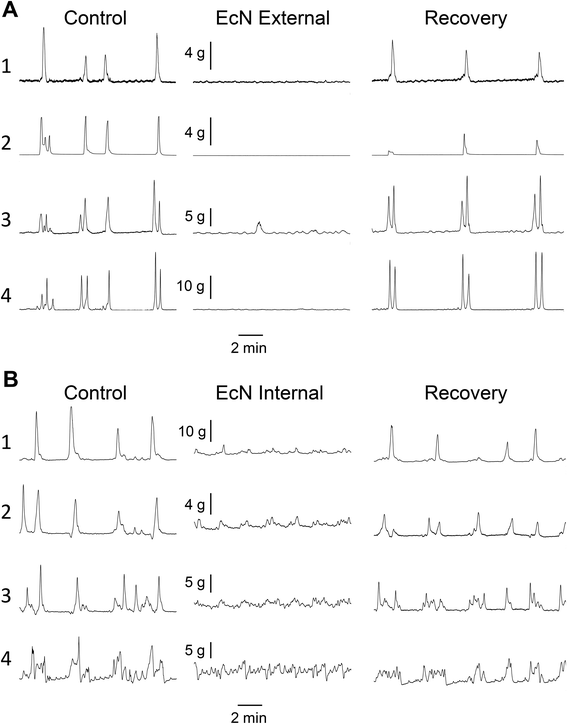 |
| Fig. 3 Effect of EcN extract on spontaneous propagating muscle contractions in the whole large intestine preparation. Representative examples from two experiments show changes in muscle tension at each of the four recording locations over time for the control and after the first application of 1/10 EcN extract either externally via the bath (a) or internally via the lumen (b). | |
When a 1/10 dilution of EcN extract was applied to the whole large intestine preparation either via perfusion through the lumen or through perfusion into the bath it inhibited motility by reducing the number of propagating synchronous contractions compared with the control by 69 ± 14% (internal) and 86 ± 8% (external), n = 5 (Fig. 4). The total number of contractions that occurred in the proximal colon (including those that propagated and those that did not) was also reduced (Fig. 4). Because aborally propagated contractions are considered to underlie the movement of contents along the large intestine we compared the effect of EcN extract on this subset of synchronized contractions with that for all synchronized contractions (forward, mid and reverse propagated). Following addition of EcN extract via the bath (external) the proportion of contractions that were aborally propagated was also decreased (Fig. 5). Synchronous contractions were completely inhibited in two preparations (and non-synchronous in one of these two) upon external application of EcN extract. Exposure to externally applied EcN extract decreased the tension of the synchronized contractions by 58–88% along the length of the large intestine (Fig. 6). Tension was decreased by 55–64% in the proximal half of the large intestine when EcN extract was internally applied (Fig. 6). In preparations with propagating contractions these were inhibited by 1 μM tetrodotoxin (TTX), n = 2.
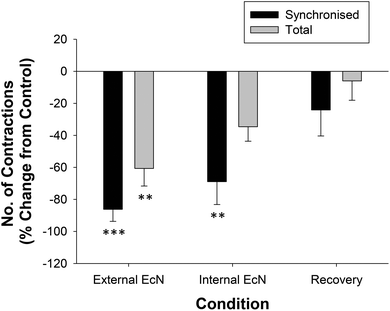 |
| Fig. 4 Summary of the effects of EcN extract on contractions in the whole large intestine preparation. The number of contractions propagated between the proximal colon and the rectum were measured during a 30 min control recording and compared with that after 30 min of exposure to 1/10 EcN (n = 5). Synchronized contractions that were temporally coordinated in forward, mid, or reverse in direction (dark bars) and all contractions (propagated and non-propagated) recorded in the proximal colon only (light bars) were calculated as a percent change from the control for each treatment condition. Asterisks indicate the significance of each treatment relative to controls (*p < 0.05; **p < 0.01; ***p < 0.001). Data show mean ± SEM. | |
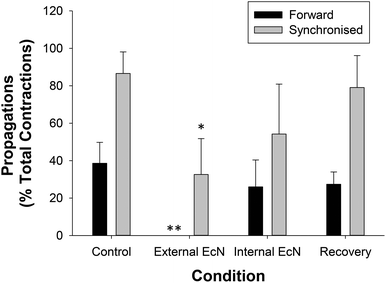 |
| Fig. 5 Summary of the effects of EcN extract on the proportion of forward or synchronized propagating contractions in the whole large intestine preparation. The proportion of contractions propagated between the proximal colon and the rectum were measured during a 30 min control recording and compared with that after 15 min of exposure to 1/10 EcN extract (n = 5). Forward propagations (dark bars) and those that were synchronized, temporally coordinated in forward, mid, or reverse direction (light bars), were calculated as a percent of the total number of contractions recorded in the proximal colon during each treatment condition. Note that externally applied EcN extract completely inhibited contractions in all 5 preparations. Asterisks indicate the significance of each treatment relative to controls (*p < 0.05; **p < 0.01; ***p < 0.001). Data show mean ± SEM. | |
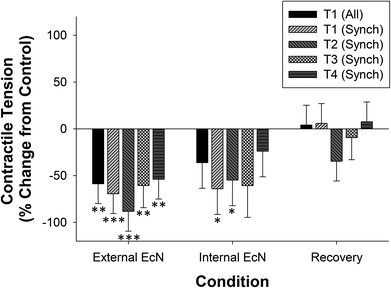 |
| Fig. 6 Summary of the effects of EcN extract on contractile tension in the whole large intestine preparation. The effect of 30 min exposure to EcN extract (n = 5) on contractile tension for synchronous contractions and those occurring only in the proximal colon (T1) were compared with a control recording at all four locations (T1; proximal, T2; mid, T3; distal, T4; rectal) and data calculated as a percent of the control in each experiment. Data show mean change in tension ± SEM. Asterisks indicate the significance of each treatment relative to controls (*p < 0.05; **p < 0.01; ***p < 0.001). | |
Discussion
The finding that EcN extract disrupted motility patterns of spontaneous muscle contractions in both isolated colonic preparations, inhibiting propagating contractions in the whole large intestine, support the hypothesis that a probiotic known to reduce diarrhea in vivo reduced motility in isolated tissue in vitro.
Distal colon segment
The inhibitory effect of the EcN bacterial extract on the tension and frequency of spontaneous contractions in the rat distal colon suggests that it would likely reduce smooth muscle motility. The spontaneous phasic contractions in the distal colon segment were not initiated by enteric neurons as we demonstrated previously that these are not inhibited by TTX21 which inhibits enteric neurons by inhibiting the voltage-gated sodium ion channel. This argues against a neural mode of action for the inhibitory effect of the EcN extract in this segment of distal colon, suggesting a direct effect on the smooth muscle or the ICC.
The reduced motility observed in the distal colon segment preparation in response to EcN extract is consistent with anti-diarrheal effects in animals. In an in vivo pig model of secretory diarrhea, pretreatment with EcN bacteria followed by challenge with a pathogenic enterotoxigenic intestinal bacteria, the pathogens were detected but clinical signs of diarrhea were absent.23 EcN also reduces the incidence of diarrhea in calves.24
The EcN extract inhibitory effect in the distal colon was different from that previously reported that EcN secreted products in bacterial media increase muscle contraction in human muscle strips from circular distal colon muscle strips (1 cm) in vitro.19 The study reported by Bar et al. differed from the current study in that it applied secreted products rather than cell-free extract and used human circular muscle strips. Furthermore, the effects of EcN secreted products were measured in bacterial media, which alone increased motility in the negative control,19 indicating that this methodology was not ideal and complicating interpretation of the apparent EcN stimulatory effect. The current study avoided the use of bacterial media because initial experiments showed that it inhibited spontaneous phasic contractions in the shorter length segments of distal colon studied. Nevertheless both increased and decreased motility have been reported for EcN in vivo13–16 indicating that multiple mechanisms and active components are likely to be involved in modulation of GI motility by EcN bacterial components.
Bacteria are known to produce short chain fatty acids (SCFA) by fermentation of carbohydrates that alter motility in the rat distal colon in vitro.25,26 EcN produces acetic acid as the main metabolic end product of its carbohydrate metabolism17 and 5 mM acetic acid decreases the frequency of spontaneous contractions in longitudinal muscle in the rat distal colon.26 However, SCFAs given by intra-colonic infusion do not alter colonic motility in healthy humans (measured by intra-colonic pressure), nor does acidic saline solution, thus additional active components are considered to be important in modulating GI motility.27
Motility in the isolated whole large intestine
The aborally propagating contractions recorded were similar to those reported previously using this method22 and their velocity was also in a similar range.2,18 In the whole large intestine preparation we found that the predominant effect of EcN extract was to inhibit propagation of the spontaneous coordinated contractions initiated in the proximal colon from reaching the rectum and to reduce the proportion of synchronized contractions compared with total contractions. Contractile tension was also reduced. The inhibition of propagating contractions measured in response to TTX has been reported previously for this preparation and confirms them as being neurally mediated.2 Our finding that EcN reduces the proportion of forward propagating contractions compared with total contractions in the whole large intestine implicates enteric neurons and therefore a neurogenic action of EcN extract.
Comparison of isolated colon preparations
By comparing two methods used to study the effect of EcN extract on intestinal motility in vitro we have demonstrated that both were useful methods that produced results consistent with the anti-diarrheal effect reported for EcN live bacteria on intestinal motility in humans.13–16 Our findings suggest that EcN acts via more than one site of action, since data from the distal colon segments inferred a myogenic action and data from the whole large intestine implicated an action on the enteric nervous system.
Furthermore, the nature of muscle contractions varied with preparation length in isolated colon consistent with previous studies. Contractions in the distal colon segments do not propagate and are predominately of non-neural origin.21 Contractions occur in the isolated whole large intestine that propagate along its length are neurally initiated.2,22 Those that do not propagate were not inhibited by TTX, suggesting a non-neural origin.22 It should be noted however that the criteria for “propagating contractions” were those recorded at all four recording force transducers sites (4–5 cm apart). Partial propagations between fewer sites were not included and would be at the resolution limit. Measurement of GI motility in humans using high resolution pressure sensors (1 cm apart) has detected partially propagating contractions in the presence of TTX, thought to be myogenic with neural influence.28 Use of this higher resolution method to study the effects of probiotics on motility in future studies will determine the changes in contractile patterns and further reveal underlying mechanisms.
These two independent preparations provide an insight into the mechanisms by which EcN can alter GI motility in an isolated rat model. The spontaneous contractile activity measured in both the distal colon segment and the whole large intestine was from a non-fasted rat in order to model normal intestinal function, rather than that in the fasted state which can alter motility.
It should be noted that diarrhea also involves secretion and absorption imbalance in the intestine that may also be affected by EcN whereas our study has focussed its impacts on motility. While other studies have shown that the whole EcN bacterium is effective at treating constipation and diarrhea in humans,13,14 our study using extract suggests that the cytoplasmic fraction is sufficient to slow hypermotility. The use of bacterial extract would also be advantageous in future studies to narrow down the likely active fraction of the bacteria and to provide a substance that could be prepared in a consistent manner across experiments (as opposed to live bacteria which replicate rapidly and would therefore vary in concentration and viability with time).
Conclusion
The main finding of this study was that EcN extract inhibited propagating contractions in the whole isolated large intestine and inhibited spontaneous contractile activity of the isolated rat distal colon, causing a disruption in motility patterns in segments of colon. The data support our hypothesis that a probiotic known to reduce diarrhea in vivo reduced motility in the colon in vitro, using EcN extract.
Because the whole large intestine preparation produces coordinated propagating contractions that mix and propel contents along, this method enables identification of bacterial substances likely to alter GI transit time in humans. This could facilitate the identification of potential probiotics that may improve intestinal comfort, for example: paediatric related problems such as colic and constipation in infants, cramps associated with diarrhea/constipation sensitivity, and constipation in adults and the elderly.
Acknowledgements
We thank L. Ryan, S. Burton, and A. Lynch for technical assistance, R. Broadhurst and B. Smith for animal breeding and H. Gillespie for animal care, C. Lloyd-West for statistical analysis, and N. Spencer for helpful discussions.
This work was supported by AgResearch Core funding (A18043).
Notes and references
-
S. K. Sarna and X. Z. Shi, in Physiology of the Gastrointestinal Tract, ed. L. R. Johnson, Elsevier Academic Press, USA, Edition edn., 2006, vol. 1, p. 1031 Search PubMed.
- M. Costa, K. N. Dodds, L. Wiklendt, N. J. Spencer, S. J. H. Brookes and P. G. Dinning, Am. J. Physiol.: Gastrointest. Liver Physiol, 2013, 305, G749–G759 CrossRef CAS PubMed.
- A. M. O'Hara and F. Shanahan, EMBO Rep., 2006, 7, 688–693 CrossRef PubMed.
- W. B. Whitman, D. C. Coleman and W. J. Wiebe, Proc. Natl. Acad. Sci. U. S. A., 1998, 95, 6578–6583 CrossRef CAS.
- M. Simrén, G. Barbara, H. J. Flint, B. M. R. Spiegel, R. C. Spiller, S. Vanner, E. F. Verdu, P. J. Whorwell and E. G. Zoetendal, Gut, 2013, 62, 159–176 CrossRef PubMed.
- Y. Ringel, E. M. M. Quigley and H. C. Lin, Am. J. Gastroenterol., 2012,(Suppl. 1), 34–40 CrossRef CAS.
- M. E. Sanders, F. Guarner, R. Guerrant, P. R. Holt, E. M. M. Quigley, R. B. Sartor, P. M. Sherman and E. A. Mayer, Gut, 2013, 62, 787–796 CrossRef CAS PubMed.
- H. J. Krammer, H. von Seggern, J. Schaumburg and F. Neumer, Coloproctology, 2011, 33, 109–113 CrossRef.
- P. A. Waller, P. K. Gopal, G. J. Leyer, A. C. Ouwehand, C. Reifer, M. E. Stewart and L. E. Miller, Scand. J. Gastroenterol., 2011, 46, 1057–1064 CrossRef CAS PubMed.
- R. Francavilla, E. Lionetti, S. Castellaneta, F. Ciruzzi, F. Indrio, A. Masciale, C. Fontana, M. M. La Rosa, L. Cavallo and A. Francavilla, Aliment. Pharmacol. Ther., 2012, 36, 363–369 CrossRef CAS PubMed.
- B. Wang, Y. K. Mao, C. Diorio, M. Pasyk, R. Y. Wu, J. Bienenstock and W. A. Kunze, FASEB J., 2010, 24, 4078–4088 CrossRef CAS PubMed.
- E. F. Verdu, P. Bercik, G. E. Bergonzelli, X. X. Huang, P. Blennerhasset, F. Rochat, M. Fiaux, R. Mansourian, I. Corthesy-Theulaz and S. M. Collins, Gastroenterology, 2004, 127, 826–837 CrossRef CAS PubMed.
- M. Mollenbrink and E. Bruckschen, Med. Klin., 1994, 89, 587–593 CAS.
- D. Plaßmann and H. Schulte-Witte, Med. Klin., 2007, 102, 888–892 CrossRef PubMed.
- J. Henker, M. Laass, B. M. Blokhin, Y. K. Bolbot, V. G. Maydannik, M. Elze, C. Wolff and J. Schulze, Eur. J. Pediatr., 2007, 166, 311–318 CrossRef PubMed.
- J. Henker, M. W. Laass, B. M. Blokhin, V. G. Maydannik, Y. K. Bolbot, M. Elze, C. Wolff, A. Schreiner and J. Schulze, Pediatr. Infect. Dis. J., 2008, 27, 494–499 CrossRef PubMed.
- U. Sonnenborn and J. Schulze, Microb. Ecol. Health Dis., 2009, 21, 122–158 CrossRef CAS.
- J. H. Chen, Q. Zhang, Y. Yu, K. Li, H. Liao, L. Jiang, L. Hong, X. Du, X. Hu, S. Chen, S. Yin, Q. Gao, X. Yin, H. Luo and J. D. Huizinga, PLoS One, 2013, 8 Search PubMed.
- F. Bar, H. Von Koschitzky, U. Roblick, H. P. Bruch, L. Schulze, U. Sonnenborn, M. Bottner and T. Wedel, Neurogastroenterol. Motil., 2009, 21, 559–566 CrossRef CAS PubMed.
- M. Massi, P. Ioan, R. Budriesi, A. Chiarini, B. Vitali, K. M. Lammers, P. Gionchetti, M. Campieri, A. Lembo and P. Brigidi, World J. Gastroenterol., 2006, 12, 5987–5994 CAS.
- J. E. Dalziel, K. E. Dunstan and S. C. Finch, J. Anim. Sci., 2013, 91, 5177–5182 CrossRef CAS PubMed.
- J. E. Dalziel, N. J. Spencer, K. E. Dunstan, A. T. Lynch, N. W. Haggarty, P. K. Gopal and N. C. Roy, Food Funct., 2014, 5, 2768–2774 CAS.
- B. Schroeder, S. Duncker, S. Barth, R. Bauerfeind, A. D. Gruber, S. Deppenmeier and G. Breves, Dig. Dis. Sci., 2006, 51, 724–731 CrossRef CAS PubMed.
- R. Von Buenau, L. Jaekel, E. Schubotz, S. Schwarz, T. Stroff and M. Krueger, J. Dairy Sci., 2005, 88, 317–323 CrossRef CAS.
- B. H. Bajka, J. M. Clarke, D. L. Topping, L. Cobiac, M. Y. Abeywardena and G. S. Patten, Nutr. Res., 2010, 30, 427–434 CrossRef CAS PubMed.
- S. Ono, S. I. Karaki and A. Kuwahara, Jpn. J. Physiol., 2004, 54, 483–493 CrossRef CAS PubMed.
- P. Jouët, D. Moussata, H. Duboc, G. Boschetti, A. Attar, C. Gorbatchef, J. M. Sabaté, B. Coffin and B. Flourié, Neurogastroenterol. Motil., 2013, 25, 943–949 CrossRef PubMed.
- P. G. Dinning, L. Wiklendt, L. Maslen, I. Gibbins, V. Patton, J. W. Arkwright, D. Z. Lubowski, G. O'Grady, P. A. Bampton, S. J. Brookes and M. Costa, Neurogastroenterol. Motil., 2014, 26, 1443–1457 CAS.
|
This journal is © The Royal Society of Chemistry 2015 |
Click here to see how this site uses Cookies. View our privacy policy here.