DOI:
10.1039/C5QI00008D
(Research Article)
Inorg. Chem. Front., 2015,
2, 713-719
Bimetallic layer-by-layer films and their application in catalytic hydrogenation of olefin†
Received
14th January 2015
, Accepted 19th June 2015
First published on 23rd June 2015
Abstract
Since bimetallic catalysts exhibit enhanced activity and stability over their monometallic counterparts, bimetallic film catalysts (Pd/bpy/Pt/bpy)n, were first prepared by alternating immersions of a substrate in a K2PdCl4 precursor (or K2PtCl4 precursor) and bpy (bpy = 4,4′-bipyridyl) solutions through a layer-by-layer (LbL) self-assembly method. The self-assembly of bimetallic films was characterized by UV-vis spectra. The absorbance increases with the number of bilayers and the film growth shows a good linear correlation between the optical absorption and the number of (Pd/bpy/Pt/bpy)n. Pd and Pt contents of (Pd/bpy/Pt/bpy)5 films were determined to be 2.0 × 10−7 and 8.4 × 10−8 mol using an inductively coupled plasma OES spectrometer (ICP-OES), respectively. The as-prepared (Pd/bpy/Pt/bpy)5 film demonstrates a remarkable catalytic activity toward hydrogenation of olefin bearing different characteristics under mild conditions. The relationship between the catalytic activity and the number of bilayers was investigated. The catalytic activity of the (Pd/bpy/Pt/bpy)n films increases with the number of bilayers below 4 bilayers for styrene hydrogenation and remains unchanged after 4 bilayers. X-ray photoelectron spectroscopy (XPS) and transmission electron microscopy (TEM) illustrate that Pd and Pt ions of (Pd/bpy/Pt/bpy)n films are in situ reduced into Pd and Pt nanoparticles (NPs) during the hydrogenation process, resulting in high catalytic activity. In addition, the control experiments show that the catalytic activity of our bimetallic catalysts is higher than that of the prereduced bimetallic catalyst and the physical mixtures of the Pd and Pt film catalysts. As compared to traditional heterogeneous catalysts, the film catalysts have superior advantages of easy separation and good recyclability, because they are easily removed from the reaction mixture without separation filtration.
1. Introduction
Research interest in bimetallic catalysts has drawn wide attention.1,2 Bimetallic catalysts, due to their tunable chemical/physical properties, exhibit unique properties which are distinct from those of their monometallic counterparts. Thus, bimetallic catalysts exhibit enhanced selectivity, activity, and stability over traditional monometallic ones.3,4 Synthetic strategies of bimetallic catalysts, including wet chemical approach, electrochemical synthesis, and thermal reduction, have been carried out in liquid mixtures or solutions. Besides, the layer-by-layer (LbL) technology, due to its simplicity, robustness, and versatility, offered an important method for bimetallic catalysts. The LbL technology has become a popular technique for thin film preparation.5–8 Compared to thin film deposition methods such as physical vapor deposition and chemical vapor deposition, the LbL method is extremely simple and cheap. As compared to traditional heterogeneous catalysts, film-based catalysts are easily removed from the reaction mixture without separation filtration.9 To our knowledge, there are many reports on monometallic Pd films using polyelectrolytes as building blocks.10–14 In general, polyelectrolyte multilayers have been explored as matrices for synthesis of zero-charge nanoparticles (NPs) in order to generate large surface area heterogeneous catalysts, such as LbL PAA/PEI-Pd(0) and Pd/PVP films (PAA = poly(acrylic acid), PEI = polyethylenimine, PVP = poly(4-vinylpyridine)). Monometallic Pd films were loaded within LbL multilayers by alternate adsorption of palladium ions and pyridyl ligand layers onto solid slides.15–20 By comparison, the bimetallic films have been rarely reported. To date, only bimetallic gold–silver (Au–Ag) nanoparticles were synthesized by LbL polyelectrolyte multilayered (PEM) nanoreactors.21–24 No matter monometallic or bimetallic LbL films, polyelectrolytes are generally ulitized as building blocks forming NPs and small molecules are rarely used as building blocks. Moreover, an in situ synthesis strategy of bimetallic catalysts within the LbL films was also rarely reported. The in situ LbL strategy of a nanoreactor possesses several distinct advantages, such as a tunable structure and composition and good chemical stability. More importantly, the in situ LbL synthesis strategy combines the synthesis and immobilization within one step, for practical applications such as a sensor and in catalysis.23
The noble metals such as Pd and Pt are widely used in hydrogenation reaction that is significant in the petroleum refining industry. Based on metal ion–ligand interaction, small organic functional ligands can be used as building blocks to construct LbL Pd/Pt bimetallic self-assembly films. Each metal ion is separated and connected by organic ligands. Since bimetallic catalysts exhibit enhanced activity, the Pd/Pt bimetallic-pyridyl catalytic film for catalytic hydrogenation reactions was investigated (Scheme 1). The Pd/Pt bimetallic-pyridyl catalytic films exhibited a remarkable catalytic activity over monometallic ones. The catalytic films are not desorbed from slides and have good recyclability. The process of the metal ions in situ reduced into NPs was investigated by X-ray photoelectron spectroscopy (XPS) and transmission electron microscopy (TEM).
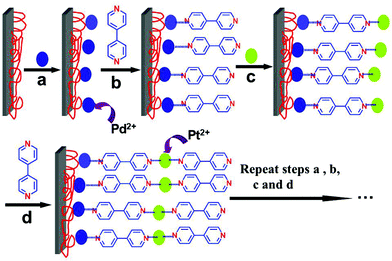 |
| Scheme 1 The self-assembly process of (Pd/bpy/Pt/bpy)n films. | |
2. Experimental section
2.1. Instrument
UV-vis absorption spectra were recorded on a quartz slide with a Lambda35 spectrophotometer (Perkin-Elmer, USA). Analysis of the noble metal content was measured by using an inductively coupled plasma OES spectrometer (ICP-OES) (Ultima2, France). Gas chromatography (GC) was performed on an Agilent 7890A (USA). High-resolution X-ray photoelectron spectra (XPS) were recorded at a takeoff angle of 45° using a PHI Quantum 2000 scanning ESCA microprobe (Physical Electronics, USA) with an Al Kα X-ray line (1486.6 eV). Transmission electron microscopy (TEM) was performed on a JEM-2010 operated at 120 kV. Small pieces of a multilayered film were scraped off the quartz substrate in ethanol and transferred to a copper grid.
2.2. Layer-by-layer assembly of (Pd/bpy/Pt/bpy)n multilayered films
The preparation of (Pd/bpy/Pt/bpy)n multilayered films are as follows: the quartz slides (size 25 mm × 12 mm × 1 mm) were cleaned with a “piranha solution” at 80 °C for 40 min, and thoroughly rinsed with water. Further purification was carried out by immersion in an H2O–H2O2–NH3OH (5
:
1
:
1) (v/v/v) bath for 30 min at 70 °C. The clean slides were first immersed in PEI solution for 20 min. The slides pre-coated with poly(ethylenimine) (PEI) were alternately immersed in K2PdCl4 aqueous solution (5 mM, 10 mL), bpy (5 mM, 10 mL) ethanol solution, K2PtCl4 aqueous solution (5 mM, 10 mL) and bpy (5 mM, 10 mL) ethanol solution for 30 min. The substrates were washed with water and dried with a nitrogen stream after each immersion. By repeating the above procedure, (Pd/bpy/Pt/bpy)n multilayered films were prepared and the self-assembly process is shown in Scheme 1.
2.3. Analysis of Pd and Pt contents in (Pd/bpy/Pt/bpy)5 multilayers
The quartz slide coated with (Pd/bpy/Pt/bpy)5 was dissolved in aqua regia (10 ml). The content of Pd and Pt was determined by using an inductively coupled plasma OES spectrometer (ICP-OES).
2.4. Typical procedures for the hydrogenation of olefin
Hydrogenation reactions were carried out under a hydrogen atmosphere (1.2 atm) at 34 °C. Typically, olefin (0.5 mmol), absolute ethanol (10 ml) and the quartz slide coated with the (Pd/bpy/Pt/bpy)5 film were added to the tube. The tube was sealed with a Teflon cap and evacuated and backfilled with H2 three times. The mixture was stirred at a constant stirring speed (500 rpm) in the tube under an H2 atmosphere. The conversion and yield were determined by GC analysis.
3. Results and discussion
3.1. Preparation and characterization
The self-assembly of bimetallic films is based on the coordination bond between metal ions and the two terminal pyridyl groups of bpy. The self-assembly process is shown in Scheme 1. As shown in Scheme 1, Pd2+ or Pt2+ ions were connected to bpy molecules. Palladium ions form the coordination bond with the terminal pyridyl group of bpy, whereas Pt ions form the coordination bond with the other pyridyl groups of bpy. The two coordination interactions constitute the films. In order to demonstrate the coordination interaction, UV-vis spectra of the solutions of metal precursors and bpy were recorded (shown in Fig. S1, see the ESI†). The band of the bpy molecules occurs at 240 nm (Fig. S1a†). The band at 233 nm is assigned to a ligand-to-metal charge transfer transition (LMCT) of the complex PdCl42− (Fig. S1b†), while the band at 227 nm is assigned to LMCT of the complex PtCl42− (Fig. S1d†). When the PdCl42− solution is mixed with the equimolar bpy molecules, two broad peaks at 244 nm and 273 nm occur (Fig. S1c†). Similarly, the PtCl42− solution is mixed with the equimolar bpy molecules, and the two peaks are at 235 nm and 265 nm, respectively (Fig. S1e†). Obviously, compared with an individual metal precursor, the peak shape and position of the mixture has been greatly changed and these peaks are red-shifted. Therefore, there exists coordination interaction between Pd and Pt ions and the terminal pyridyl group of bpy.
On this basis, the bimetallic-pyridyl (Pd/bpy/Pt/bpy)n films were prepared. UV-vis spectra also demonstrate the self-assembly process. The absorbance increases with the number of bilayers (Fig. 1). The absorbance is from bpy ligand layers. The relationship between absorbance and the number of bilayers is shown in Fig. 1b. The absorbance increases with the number of outer layers of Pd/bpy or Pt/bpy. The film growth shows a good linear correlation with R2 = 0.9965 (R2 is a statistical calculation used with linear regressions) between the optical absorption at λmax = 278 nm and the number of (Pd/bpy/Pt/bpy)n, suggesting that bpy is incorporated into the films with a regular growth.
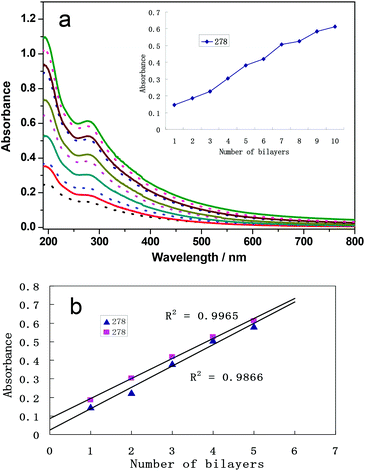 |
| Fig. 1 UV-vis spectra of (Pd/bpy/Pt/bpy)n films (a) and the relationship between absorbance and the number of bilayers (b). The dashed lines or triangle represent the outer layer of Pd/bpy; the solid lines or square represent the outer layer of Pt/bpy. | |
3.2. Catalytic performance of films for the olefin hydrogenation
The relationship between the substrate conversion and the number of (Pd/bpy/Pt/bpy)n bilayers was investigated. The catalytic activity of the films increases with the number of bilayers (as shown in Fig. 2). However, when the number of (Pd/bpy/Pt/bpy)n bilayers reaches 5, the catalytic activity is not greatly improved and remains similar to 4 bilayers. This indicates that the activity is also related to the NPs of films. After 4 bilayers, thicker LbL films produce NPs of lower quality.
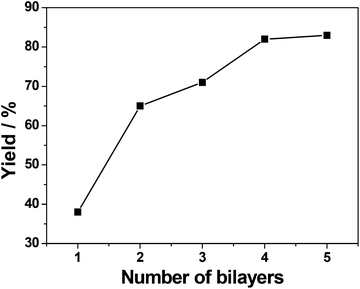 |
| Fig. 2 Relationship between styrene conversion and the number of (Pd/bpy/Pt/bpy)n bilayers during the 30 min reaction time. | |
To explore the generality of the catalytic system, olefins bearing different characteristics were tested. The results are summarized in Table 1. For example, nitrobenzene is reduced into aniline (Table 1, entry 2), 3,7-dimethyl-1,6-octadien-3-ol is selectively hydrogenated to 3,7-dimethyl-6-octen-3-ol (Table 1, entry 4), and benzalacetone is selectively hydrogenated to benzylacetone (Table 1, entry 5). Moreover, for cyclohexene hydrogenation, the bimetallic-pyridyl film (Pd/bpy/Pt/bpy)5 gave good yield in 3.6 h (Table 1, entry 8), while monometallic (Pd/bpy)10 film catalysts gave good yield in 8.0 h.25 Other olefin substrates such as 3,7-dimethyl-1,6-octadien-3-ol and ethyl cinnamate, (Pd/bpy/Pt/bpy)5 have high catalytic activity than the corresponding monometallic (Pd/bpy)10 film catalysts.25 Although Pt has catalytic activity in olefin hydrogenation, monometallic (Pt/bpy)10 film catalysts exhibited lower catalytic activity for styrene or no activity for other olefin hydrogenations. Pd and Pt contents of (Pd/bpy/Pt/bpy)5 films were determined to be 2.0 × 10−7 and 8.4 × 10−8 mol, respectively. Turnover number (TON) (moles of substrate converted per mole of catalyst) is calculated to be 1760, which is higher than that of the monometallic film (Pd/bpy)10 in a previous report (TON: 1190).24 Therefore, the bimetallic-pyridyl film (Pd/bpy/Pt/bpy)5 exhibits much better catalytic efficiency than monometallic (Pd/bpy)10 film catalysts in a previous report.25
Table 1 Hydrogenation of olefin and nitrobenzene over film catalysts
Entry |
Substrate |
Product |
Time |
Yielda (%) |
Reaction conditions: substrate (0.5 mmol), absolute ethanol (10 ml). Catalysts: (Pd/bpy/Pt/bpy)5, stirring, H2 (1.2 atm), 34 °C. Determined by GC methods. |
1 |
|
|
0.6 h |
>99 |
2 |
|
|
2.6 h |
>99 |
3 |
|
|
6 h |
86 |
4 |
|
|
2 h |
96 |
5 |
|
|
4.4 h |
94 |
6 |
|
|
4.3 h |
>99 |
7 |
|
|
1.5 h |
>99 |
8 |
|
|
3.6 h |
>99 |
To ensure that the immobilized NPs in the films represent the catalytically active species rather than soluble species leaching from the film during the reaction process, a hot filtration test was performed. The (Pd/bpy/Pt/bpy)5 catalysts were removed after 1 h for the nitrobenzene hydrogenation reaction and the reaction mixtures were kept to react for an additional 2 h under identical reaction conditions, no changes in conversion were observed. The ICP analysis of the final reaction mixture shows that Pd and Pt concentrations are 0.056 ppm and 0.075 ppm, respectively, indicating that metal leaching is negligible during the catalytic reaction. These results demonstrate that the reaction proceeds in a heterogeneous fashion.
The recycling of (Pd/bpy/Pt/bpy)5 films was investigated. After the completion of a catalytic reaction, solid glass slides modified with (Pd/bpy/Pt/bpy)5 films were simply removed from the product, washed with ethanol, dried, and then reused in another catalytic cycle. This process was repeated for at least 10 cycles for the hydrogenation of styrene for 40 min and the yield of the product remains over 95% (Fig. 3). For nitrobenzene hydrogenation (Fig. 4), the yield of aniline is 76% for 2 h in the first catalytic cycle and the yield is decreased to 67% in the second cycle. The higher activity is due to the in situ reduced (Pd/bpy/Pt/bpy)5 film catalyst in the first cycle. However, in another catalytic cycle, the obvious decrease of the catalytic activity did not occur and the yield remained stable. The number of cycles also reaches 10. Therefore, the results show that our film catalysts have good recyclability. Moreover, the recycling is more convenient as compared to the traditional heterogeneous catalysts, because they are easily removed from the reaction mixture without separation filtration.
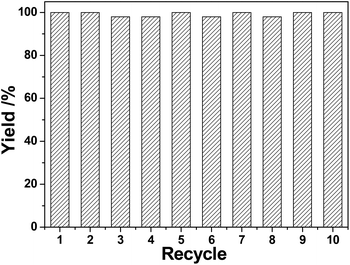 |
| Fig. 3 Recycling of (Pd/bpy/Pt/bpy)5 films for 10 runs of styrene hydrogenation for 40 min. | |
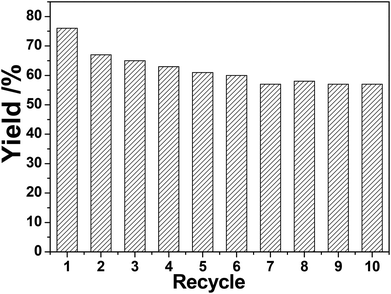 |
| Fig. 4 Recycling of (Pd/bpy/Pt/bpy)5 films for 10 runs of nitrobenzene hydrogenation for 2 h. | |
The catalytic process was investigated by TEM and XPS. During the process of olefin hydrogenation, hydrogen gas was used as a reductant for metal ions besides a hydrogen source for the hydrogenation reactions. XPS is recorded for the catalytic film after catalytic reduction. As shown in Fig. 5, the peak position and intensity for the metal elements largely changes before and after the hydrogen reduction. Firstly, before the hydrogenation reaction, Pd ions are Pd(II) ions with the binding energy 337.4/342.4 eV, whereas the binding energy 72.8/76.0 eV corresponds to Pt(II) ions. After the 10th cycle of nitrobenzene hydrogenation, a couple of new peaks at 335.3/340.5 eV with high intensity are ascribed to Pd(0) (Fig. 5a), which indicates that Pd(II) ions are reduced to Pd(0).26 Meanwhile, a couple of new peaks at 71.5/74.7 eV occur with high intensity (Fig. 5b), which corresponds to Pt(0).27 During the process of styrene reduction, XPS also demonstrates similar behaviors (Fig. 6). Most of the Pd(II) ions with the binding energy 337.4/342.4 eV are reduced into Pd(0) states (335.3/340.5 eV), while less than half of Pt(II) ions (72.8/76.0 eV) are reduced into Pt(0) states (71.5/74.7 eV) (Fig. 6). As seen from the peak intensity, Pd(II) ions are more easily reduced than Pt(II) ions under the same conditions, because the peak intensity of Pt(II) ions is stronger than that of Pd(II) ions after reduction. These results indicate that metal ions are bivalent metal ions before olefin hydrogenation, but metal ions are in the presence of mixed valences metal(II) and metal(0) with the hydrogenation carried through or increasing the number of cycles. Finally, all metal ions are reduced into NPs.
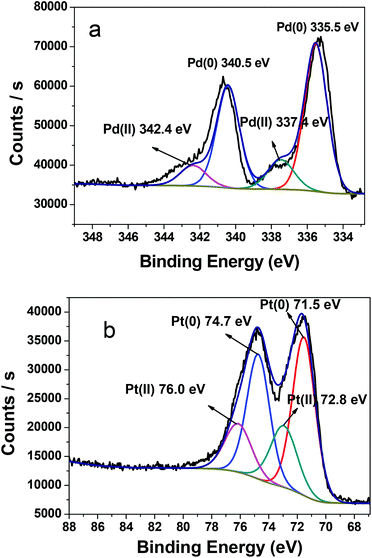 |
| Fig. 5 XPS spectra of the Pd3d (a) and Pt4f (b) region for the (Pd/bpy/Pt/bpy)5 film deposited on the solid glass substrate after the 10th cycle of nitrobenzene hydrogenation. | |
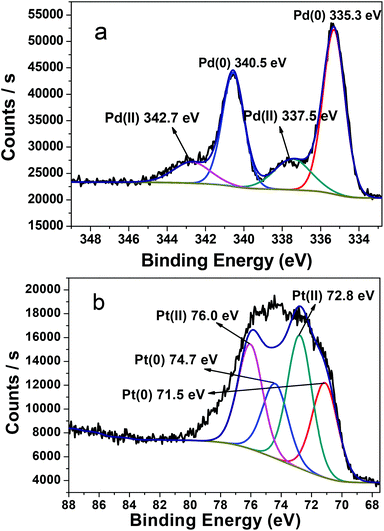 |
| Fig. 6 XPS spectra of the Pd3d (a) and Pt4f (b) region for the (Pd/bpy/Pt/bpy)5 film deposited on the solid glass substrate after the 10th cycle of styrene hydrogenation. | |
As shown in TEM images, the dispersion of particles in (Pd/bpy/Pt/bpy)5 films is uniform and the mean size of NPs is 3.79 nm after the 10th cycle of styrene hydrogenation (Fig. 7). When the reaction is performed for a long time, NPs tend to aggregate after the 10th cycle of nitrobenzene hydrogenation (Fig. 8). However, the mean size of NPs still remains small (5.46 nm). Small sizes of NPs result in high catalytic activity after recycling for many times.
 |
| Fig. 7 TEM images of (Pd/bpy/Pt/bpy)5 films after the 10th cycle of styrene hydrogenation. | |
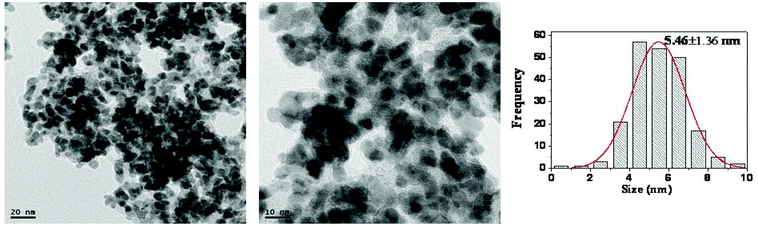 |
| Fig. 8 TEM images of (Pd/bpy/Pt/bpy)5 films after the 10th cycle of nitrobenzene hydrogenation. | |
In order to confirm the high catalytic activity of the catalyst, the control experiments using the prereduced (Pd/bpy/Pt/bpy)5 bimetallic catalyst (H2 reduction at 150 °C) and the physical mixtures of the Pd and Pt film catalysts were performed. The results are shown in Table 2. TOF (moles of substrate converted per mole of catalyst per h) of (Pd/bpy)5 and (Pt/bpy)5 mixed film catalysts is 295 h−1 (Table 2, entry 1), while TOF of the prereduced (Pd/bpy/Pt/bpy)5 bimetallic catalyst is 378 h−1 (Table 2, entry 2). The in situ reduced (Pd/bpy/Pt/bpy)5 film catalyst has the highest TOF of 669 h−1 (Table 2, entry 3). This may be explained as the following: metal ions are gradually reduced into NPs for bimetallic films, but Pt(II) ions are more difficult to reduce than Pd(II) ions under the same conditions. Therefore, the catalytic activity of bimetallic (Pd/bpy/Pt/bpy)5 films comes from Pd NPs firstly. With the reaction going on, the reduced Pt NPs appeared and the synergistic action of bimetallic Pd/Pt might enhance the catalytic activity.28,29 Moreover, the internal structure of the bimetallic complex films may have an effect on the catalytic activtiy. The terminal pyridyl group of bpy is coordinated to Pd ions, while the other pyridyl group is connected to the Pt ion. Thus, Pd NPs of bimetallic films cannot easily aggregate compared with monometallic films, resulting in high catalytic activity.
Table 2 Hydrogenation of nitrobenzene over different film catalysts

|
Entry |
Catalysts |
Pd (mol) ×10−7 |
Pt (mol) ×10−8 |
Yielda (%) |
TOF (h−1) |
Reaction conditions: 0.5 mmol of nitrobenzene, absolute ethanol (10 ml), stirring, H2 (1.2 atm), 34 °C, time (2.0 h), TOF (moles of substrate converted per mole of catalyst per h). Determined by GC methods. |
1 |
(Pd/bpy)5 film and (Pt/bpy)5 film |
1.9 |
1.3 |
24 |
295 |
2 |
(Pd/bpy/Pt/bpy)5 film (prereduced) |
2.0 |
8.4 |
43 |
378 |
3 |
(Pd/bpy/Pt/bpy)5 film |
2.0 |
8.4 |
76 |
669 |
4. Conclusions
In the present work, bimetallic layer-by-layer catalytic (Pd/bpy/Pt/bpy)n films were first successfully prepared. The bimetallic-pyridyl catalytic films exhibit a superior catalytic activity toward hydrogenation of olefin under mild conditions. Regarding activity, (Pd/bpy/Pt/bpy)5 films exhibit better performance than that of monometallic film counterparts.25 The results of XPS and TEM also further demonstrated that Pd(II) and Pt(II) ions of bimetallic films were gradually in situ converted to NPs, resulting in higher activity. The control experiments further indicate that Pd and Pt NPs in situ reduced and the synergistic action of bimetallic Pd/Pt result in higher catalytic activity. Moreover, our bimetallic catalytic films have the superiority compared with traditional heterogeneous catalysts, due to high efficiency, ease of catalyst separation and good recyclability.
Acknowledgements
This work was financially supported by the 973 Program (2011CB932504 and 2012CB821705), NSFC (21331006, 21173222 and 21221001), the “Strategic Priority Research Program” of the Chinese Academy of Sciences (XDA09030102), and the Key Project from CAS.
Notes and references
- S. Hirasawa, H. Watanabe, T. Kizuka, Y. Nakagawa and K. Tomishige, J. Catal., 2013, 300, 205 CrossRef CAS PubMed.
- W. G. Menezes, L. Altmann, V. Zielasek, K. Thiel and M. Bäumer, J. Catal., 2013, 300, 125 CrossRef CAS PubMed.
- Z. Wei, J. Sun, Y. Li, A. K. Datyec and Y. Wang, Chem. Soc. Rev., 2012, 41, 7994 RSC.
- W. Yu, M. D. Porosoff and J. G. Chen, Chem. Rev., 2012, 112, 5780 CrossRef CAS PubMed.
- Y. Xiang, S. Lu and S. P. Jiang, Chem. Soc. Rev., 2012, 41, 7291 RSC.
- H. Xu, W. Cao and X. Zhang, Acc. Chem. Res., 2013, 46, 1647 CrossRef CAS PubMed.
- M. Yang, S. Lu, J. Lu, S. P. Jiang and Y. Xiang, Chem. Commun., 2010, 1434 RSC.
- A. Zhuk and S. A. Sukhishvili, Soft Matter, 2013, 9, 5149 RSC.
- A. Mentbayeva, A. Ospanova, Z. Tashmuhambetova, V. Sokolova and S. Sukhishvili, Langmuir, 2012, 28, 11948 CrossRef CAS PubMed.
- S. Kidambi, J. Dai, J. Li and M. L. Bruening, J. Am. Chem. Soc., 2004, 126, 2658 CrossRef CAS PubMed.
- S. Kidambi and M. L. Bruening, Chem. Mater., 2005, 17, 301 CrossRef CAS.
- S. Bhattacharjee and M. L. Bruening, Langmuir, 2008, 24, 2916 CrossRef CAS PubMed.
- Y. Wang and J.-K. Lee, J. Mol. Catal. A: Chem., 2007, 263, 163 CrossRef CAS PubMed.
- Z. Ren, H.-L. Wang, Y.-Q. Cai, M. Chen and D.-J. Qian, Mater. Chem. Phys., 2011, 127, 310 CrossRef CAS PubMed.
- M. Altman, A. D. Shukla, T. Zubkov, G. Evmenenko, P. Dutta and M. E. van der Boom, J. Am. Chem. Soc., 2006, 128, 7374 CrossRef CAS PubMed.
- M. Altman, O. V. Zenkina, T. Ichiki, M. A. Iron, G. Evmenenko, P. Dutta and M. E. van der Boom, Chem. Mater., 2009, 21, 4676 CrossRef CAS.
- J. Choudhury, R. Kaminker, L. Motiei, G. de Ruiter, M. Morozov, F. Lupo, A. Guilino and M. E. van der Boom, J. Am. Chem. Soc., 2010, 132, 9295 CrossRef CAS PubMed.
- T. N. Milie, N. Chi, D. G. Yablon, G. W. Flynn, J. D. Batteas and C. M. Drain, Angew. Chem., Int. Ed., 2002, 41, 2117 CrossRef.
- T. Milic, J. C. Garno, J. D. Batteas, G. Smeureanu and C. M. Drain, Langmuir, 2004, 20, 3974 CrossRef CAS.
- S. Gao, Z. Zheng, J. Lü and R. Cao, Chem. Commun., 2010, 46, 7584 RSC.
- L. Zhang, C. Wang and Y. Zhang, Appl. Surf. Sci., 2012, 258, 5312 CrossRef CAS PubMed.
- L. Shang, L. Jin, S. Guo, J. Zhai and S. Dong, Langmuir, 2010, 26, 6713 CrossRef CAS PubMed.
- X. Liu, A. Wang, X. Yang, T. Zhang, C.-Y. Mou, D.-S. Su and J. Li, Chem. Mater., 2009, 21, 410 CrossRef CAS.
- X. Zhang and Z. Su, Adv. Mater., 2012, 24, 4574 CrossRef CAS PubMed.
- S. Gao, M. Cao, W. Li and R. Cao, J. Mater. Chem. A, 2014, 2, 12185 CAS.
- S. Kidambi and M. L. Bruening, Chem. Mater., 2005, 17, 301 CrossRef CAS.
- C. Dablemont, P. Lang, C. Mangeney, J.-Y. Piquemal, V. Petkov, F. Herbst and G. Viau, Langmuir, 2008, 24, 5832 CrossRef CAS PubMed.
- R. W. J. Scott, A. K. Datye and R. M. Crooks, J. Am. Chem. Soc., 2003, 125, 3708 CrossRef CAS PubMed.
- B. A. Kakade, T. Tamaki, H. Ohashi and T. Yamaguchi, J. Phys. Chem. C, 2012, 116, 7464 CAS.
Footnote |
† Electronic supplementary information (ESI) available. See DOI: 10.1039/c5qi00008d |
|
This journal is © the Partner Organisations 2015 |
Click here to see how this site uses Cookies. View our privacy policy here.