DOI:
10.1039/C4QO00287C
(Research Article)
Org. Chem. Front., 2015,
2, 55-59
Reaction of N-heterocyclic carbenes with 13-vertex closo-carboranes: synthesis and structural characterization of zwitterionic salts of 13-vertex nido-carboranes†
Received
3rd November 2014
, Accepted 10th December 2014
First published on 10th December 2014
Abstract
A series of 1
:
1 13-vertex nido carborane–carbene adducts were prepared from the reaction of 13-vertex closo-carboranes with sterically demanding N-heterocyclic carbenes (NHCs) in moderate to very good yields. Single-crystal X-ray analyses reveal that they are zwitterionic salts consisting of the 13-vertex nido-carborane cage and the imidazolium moiety that are connected via a C–B single bond. A mechanistic study shows that 13-vertex closo-carboranes and NHCs undergo a fast acid–base reaction to generate intermediates, i.e imidazolium salts of carborane monoanions, that are slowly converted to the final products. The stability of the intermediates is dependent upon the basicity of NHCs.
Introduction
The chemistry of carboranes has been dominated by 12-vertex carboranes over half a century.1 Only in recent years has significant progress been made in the synthesis and reactivity studies of supercarboranes (carboranes with more than 12 vertices).2 A number of 13- and 14-vertex carboranes as well as their metal complexes have been prepared and structurally characterized.3–13 They share some chemical properties with 12-vertex carboranes, and on the other hand they have their own unique features. For example, 13-vertex carborane 1,2-(CH2)3-1,2-C2B11H11 can undergo single-electron reduction to afford a stable carborane radical anion with [2n + 3] framework electrons,14 which accepts additional electrons to give 13-vertex nido-carborane dianions.5 1,2-(CH2)n-1,2-C2B11H11 (n = 3, 4) reacts also with various nucleophiles to give the cage boron and/or carbon extrusion products closo-CB11−, nido-CB10−, closo-CB10−, or closo-C2B10 depending on the nature of the nucleophiles.15–19 Under strong basic conditions, α-deprotonation of the methylene linkage in 13-vertex carboranes proceeds to generate the monoanion [1,2-CH(CH2)2-1,2-C2B11H11]− with exo C
C π bonding.9 It is noted that these reactions often couple with the rearrangement of the cage geometry, making the isolation of the first intermediate very difficult.2e
On the other hand, 12-vertex o-carboranes react with N-heterocyclic carbenes (NHCs) to give the imidazolium salts of carborane anions,20 or 1
:
2 adducts of nido-carborane–carbene,21 or zwitterionic salts of imidazolium nido-carboranyl22 depending upon the size of NHCs. The results show that a sterically demanding NHC, 1,3-bis(2,6-diisopropylphenyl)imidazol-2-ylidene, can stabilize the first intermediate in the deboration reaction of o-carboranes with nucleophiles.22 Intrigued by this work, we wondered whether such a NHC could react with 13-vertex carboranes, leading to the stabilization and isolation of the first intermediate in the reactions of 13-vertex carboranes with various nucleophiles, which would shed some light on the reaction mechanism.
Results and discussion
Synthesis
Treatment of 1,2-(CH2)3-1,2-C2B11H11 with 1 equiv. of 1,3-bis(2,6-diisopropylphenyl)-imidazol-2-ylidene in THF at room temperature for one day gave a zwitterionic salt of imidazolium nido-C2B11 anion 1 as a white solid in 82% isolated yield (Scheme 1). In a similar manner, a class of such zwitterionic salts 2–12 were prepared in 55–81% isolated yields. These results also indicate that the substituents at the cage B(3) position or at phenyl of the NHC unit have no obvious effects on the reactions. Both imidazolyl and imidazolinyl NHCs work equally well. However, the carbon-chain length between the two cage carbon atoms has an influence on both the reactivity and product yield. 13-Vertex carboranes with a –(CH2)4– linkage are less reactive than those having a –(CH2)3– linkage, leading to lower yields of the products.
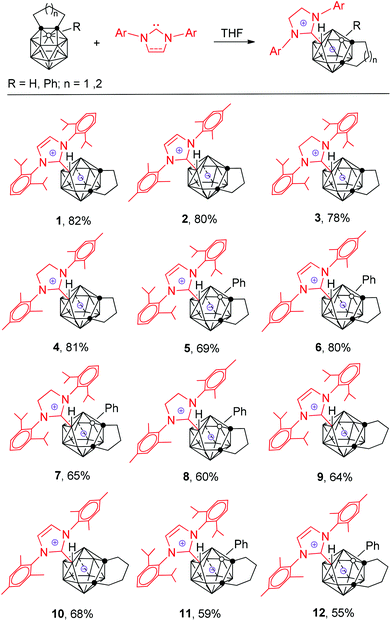 |
| Scheme 1 Reaction of 13-vertex closo-carboranes with NHCs. | |
Complexes 1–12 are stable toward excess NHCs probably due to steric reasons. These complexes are also air- and moisture-stable, as well as thermally stable, which is significantly different from their 12-vertex analogs.22 They can even be purified by chromatography on silica gel.
Complexes 1–12 were fully characterized from various spectroscopic data as well as elemental analyses. The 1H NMR spectra were in very good agreement with the proposed structures corresponding to 1
:
1 carborane–carbene adducts. The 11B NMR spectra exhibited a pattern of 1
:
1
:
2
:
1
:
2
:
2
:
1
:
1 for 1, 1
:
1
:
1
:
2
:
2
:
2
:
1
:
1 for 2 and 5, 1
:
1
:
2
:
1
:
1
:
2
:
1
:
1
:
1 for 3, 1
:
1
:
1
:
1
:
1
:
2
:
1
:
1
:
1
:
1 for 4, 1
:
1
:
1
:
2
:
3
:
1
:
1
:
1 for 6, 1
:
2
:
1
:
2
:
2
:
1
:
1
:
1 for 7, 1
:
1
:
1
:
1
:
1
:
3
:
1
:
1
:
1 for 8, 1
:
1
:
1
:
2
:
1
:
2
:
1
:
1
:
1 for 9–11, and 1
:
1
:
1
:
2
:
1
:
1
:
1
:
1
:
1
:
1 for 12. The chemical shifts of the unique B atoms bound to NHCs fell in the range −1.4–−3.8 ppm with JBH = 40–84 Hz, suggesting that this boron still binds to an exo-hydrogen atom. In addition, the proton coupled 11B NMR spectra showed one singlet at 13.9 ppm in 5, 13.5 ppm in 6, 13.2 ppm in 7, 13.6 ppm in 8, 13.0 ppm in 11, and 11.5 ppm in 12 corresponding to the BPh vertex in these complexes.
On the other hand, reaction of 1,2-(CH2)4-1,2-C2B11H11 with 1 equiv. of 1,3,4,5-tetramethylimidazol-2-ylidene in THF at room temperature afforded instantly an imidazolium salt of carborane anion [1,2-CH(CH2)3-1,2-C2B11H11][1′,3′,4′,5′-Me4-1′,3′-N2C3H] (13) as a white solid in 99% isolated yield (Scheme 2). Complex 13 was very stable and did not yield the desired zwitterionic salt even under prolonged heating, which is largely unexpected.
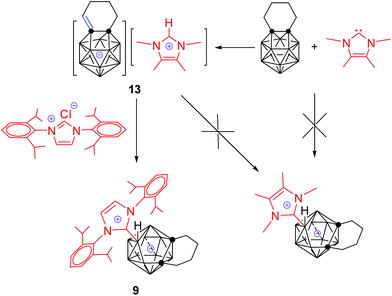 |
| Scheme 2 Reaction of 1,2-(CH2)4-1,2-C2B11H11 with 1,3,4,5-tetramethyl imidazol-2-ylidene. | |
The 1H NMR spectrum of 13 displayed a triplet of the unique exo α-CH at 5.82 ppm with J = 4.8 Hz, indicative of the α-CH being split by its neighboring methylene unit. Accordingly, the 13C chemical shift of the α-CH was observed at 152.0 ppm. Its 11B NMR spectrum exhibited a pattern of 1
:
5
:
5, ranging from 6.3 to −21.8 ppm. These characteristic spectroscopic data are consistent with those observed for [1,2-CH(CH2)3-1,2-C2B11H11][PPN].9 Elemental analyses also confirmed the composition of 13.
The above results clearly indicate that 1,3,4,5-tetramethylimidazol-2-ylidene serves as a base20,21 to deprotonate the exo α-methylene,9 leading to the formation of 13. We wondered whether such a salt is an intermediate for the formation of the final products 1–12. To this end, 11B NMR spectroscopy was used to monitor the reaction of 1,2-(CH2)3-1,2-C2B11H11 with 1,3-bis(2,6-diisopropylphenyl)-imidazol-2-ylidene at room temperature in THF, and the results are compiled in Fig. 1. Comparing these 11B NMR data with those of [1,2-CH(CH2)2-1,2-C2B11H11]−,9 it is suggested that the deprotonation of α-methylene in 13-vertex carboranes proceeds instantly after mixing with NHC to afford the imidazolium salt of carborane anion that is slowly converted to the final product 1.
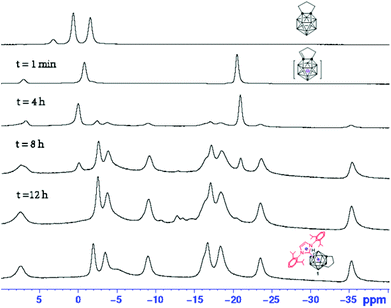 |
| Fig. 1
11B NMR spectra for the reaction of 1,2-(CH2)3-1,2-C2B11H11 with 1,3-bis(2,6-diisopropylphenyl)-imidazol-2-ylidene at room temperature in THF. | |
Subsequently, a question arises as to why 13 is stable and can not be converted to the corresponding zwitterionic salt. In view of the transformation from imidazolium salt of carborane anions to the final product, proton transfer from the imidazolium cation back to the exo α-CH unit is necessary. It has been documented that the N,N-dialkylimidazolium ion [NHCalkyl]H (pKa ∼ 23) is a weaker acid than the corresponding N,N-diarylimidazolium one [NHCaryl]H (pKa ∼ 21).23,24 We speculated that the relatively weak acidity of the 1,3,4,5-tetramethylimidazolium cation in 13 cannot protonate the carborane anion, resulting in the stability of 13. If this is true, addition of N,N-diarylimidazolium salt should promote the proton transfer, leading to the formation of the desired product. In fact, treatment of 13 with 1,3-bis(2,6-diisopropylphenyl)imidazolium chloride in THF at 80 °C gave 9 in 65% isolated yield (Scheme 2). These results show that the basicity of NHCs has a large impact on their reaction with 13-vertex carboranes.
Structure
The solid-state structures of 1, 3–5 and 9–11 were further confirmed by single-crystal X-ray analyses and the representative structures of 1, 5 and 10 are shown in Fig. 2–4, respectively. As illustrated in the figures, they have a very similar nido cage geometry with the NHC bound to the central boron atom of the C2B3 open face. The cluster framework can be viewed as a 14-vertex closo deltahedron with one vertex missing.4,6,10 As shown in Table 1, the B–C(carbene) bond distances (1.59–1.60 Å) found in all structures fall in the range 1.58–1.64 Å normally observed for the B–C(carbene) bond21,22,25,26 in carbene adducts, such as 1.59(1) Å in [1,3-bis(2,6-diisopropylphenyl)-imidazol-2-ylidene]BH3,25 1.62(1) Å in [1,3-bis(2,6-diisopropylphenyl)-imidazol-2-ylidene]BBr325 and 1.61(1) Å in μ-9,10,11-[2′-{1′,3′-[2′′,6′′-iPr2(C6H3)]2-1′,3′-N2C3H2}]BH-7,8-C2B9H11.22 The cage C–C distances of 1.54–1.57 Å are very close to those found in 13-vertex nido-carborane anions.5,10 These results suggest that complexes 1–12 are best viewed as zwitterionic salts with negative charge on the cage and positive charge on the imidazolium ring.
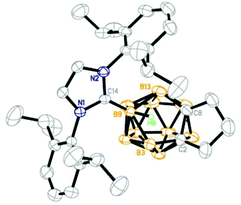 |
| Fig. 2 Molecular structure of 1. | |
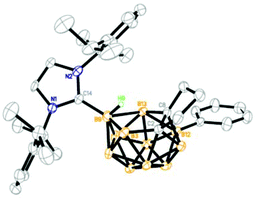 |
| Fig. 3 Molecular structure of 5. | |
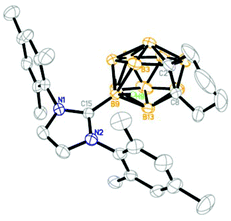 |
| Fig. 4 Molecular structure of 10. | |
Table 1 Selected bond lengths (Å) in 1, 3–5 and 9–11
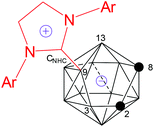
|
Complex |
CNHC–B(9) |
C(2)–C(8) |
C(8)–B(13) |
B(13)–B(9) |
B(9)–B(3) |
B(3)–C(2) |
1
|
1.605(6) |
1.540(6) |
1.668(8) |
2.124(7) |
1.825(8) |
1.577(7) |
3
|
1.602(4) |
1.541(4) |
1.661(5) |
2.135(4) |
1.836(4) |
1.578(4) |
4
|
1.601(8) |
1.557(8) |
1.687(8) |
2.180(9) |
1.835(9) |
1.586(9) |
5
|
1.600(7) |
1.537(7) |
1.671(8) |
2.076(7) |
1.817(9) |
1.583(7) |
9
|
1.599(4) |
1.560(4) |
1.689(5) |
2.082(4) |
1.823(4) |
1.585(4) |
10
|
1.592(3) |
1.555(4) |
1.657(4) |
2.044(4) |
1.810(3) |
1.580(4) |
11
|
1.586(5) |
1.567(4) |
1.653(5) |
2.098(5) |
1.823(6) |
1.594(5) |
Experimental
Preparation of 2,8-(CH2)3-9-(2′-{1′,3′-[2′′,6′′-iPr2(C6H3)]2-1′,3′-N2C3H2})-2,8-C2B11H11 (1)
A THF solution (5 mL) of 1,3-bis(2,6-diisopropylphenyl)imidazol-2-ylidene (78 mg, 0.2 mmol) was slowly added to a stirring solution of 1,2-(CH2)3-1,2-C2B11H11 (39 mg, 0.2 mmol) in THF (5 mL) at room temperature, and the mixture was stirred for 1 d. After removal of the solvent, the residue was subjected to chromatographic separation (SiO2, 300–400 mesh, n-hexane–CH2Cl2 3
:
1), giving 1 as a white solid (96 mg, 82%). X-ray-quality crystals were obtained by recrystallization from acetone. 1H NMR (acetone-d6): δ 8.02 (s, 2H, imidazolium NCH), 7.56 (t, J = 7.6 Hz, 2H, C6H3), 7.44 (d, J = 7.6 Hz, 2H, C6H3), 7.37 (d, J = 7.6 Hz, 2H, C6H3), 2.72 (m, 2H, CH(CH3)2), 2.58 (m, 2H, CH(CH3)2), 2.52 (m, 1H, CH2), 2.14 (m, 1H, CH2), 1.61 (m, 1H, CH2), 1.46 (d, J = 6.8 Hz, 6H, CH(CH3)2), 1.40 (m, 3H, CH2), 1.34 (d, J = 6.8 Hz, 6H, CH(CH3)2), 1.20 (d, J = 6.8 Hz, 6H, CH(CH3)2), 1.15 (d, J = 6.8 Hz, 6H, CH(CH3)2). 13C{1H} NMR (acetone-d6): δ 146.7, 146.3, 134.4, 132.0, 127.1, 125.1, 124.9 (C6H3 & imidazolium NCH), 76.0 (v1/2 = 40 Hz), 48.5 (v1/2 = 36 Hz) (cage C), 44.3, 35.6, 21.3 (CH2), 30.0, 29.9 (CH(CH3)2), 26.3, 26.2, 22.4, 22.2 (CH(CH3)2), the imidazolium NCN carbon was not observed. 11B NMR (acetone-d6): δ 7.5 (d, J = 142 Hz, 1B), −1.9 (d, J = 64 Hz, 1B), −3.5 (d, J = 151 Hz, 2B), −9.0 (d, J = 131 Hz, 1B), −16.7 (d, J = 148 Hz, 2B), −18.4 (d, J = 152 Hz, 2B), −23.5 (d, J = 132 Hz, 1B), −35.2 (d, J = 132 Hz, 1B). IR (KBr, cm−1): νBH 2545 (vs). HRMS: m/z calcd for C32H53B11N2 [M − 2H]+: 582.5160. Found: 582.5183. Anal. Calcd for C32H53B11N2: C, 65.73; H, 9.14; N, 4.79. Found: C, 65.77; H, 9.13; N, 4.78.
Conclusions
Reaction of 13-vertex closo-carboranes with NHCs gave instantly intermediates, imidazolium salts of carborane monoanions with exo-π-bonding to the cage carbon atom,9 that were slowly converted to the zwitterionic salts with the nido-carborane unit. The stability of the intermediates is highly dependent upon the basicity of NHCs. Strong bases such as N,N-dialkyl NHCs lead to the isolation and characterization of the intermediates, whereas relatively weak bases such as N,N-diaryl NHCs result in the formation of final products 1–12. These results suggest that in the reactions of 13-vertex closo-carboranes with nucleophiles reported in the literature,15–19 the nucleophiles may attack the most electron deficient cage B (connected to both cage C atoms) to form the first intermediates with structures similar to 1–12.
Acknowledgements
The work described in this paper was supported by grants from the Research Grants Council of the Hong Kong Special Administration Region (project no. CUHK 7/CRF/12G and 14306114).
Notes and references
-
(a)
R. N. Grimes, Carboranes, Elsevier, Oxford, 2nd edn, 2011 Search PubMed;
(b)
N. S. Hosmane, Boron Science: New Technologies and Applications, CRC Press, Boca Raton, FL, 2011 Search PubMed;
(c)
M. A. Fox, in Comprehensive Organometallic Chemistry III, ed. R. H. Crabtree and D. M. P. Mingos, Elsevier, Oxford, 2007, vol. 3, p. 49 Search PubMed;
(d) Z. Xie, Coord. Chem. Rev., 2006, 250, 259 CrossRef CAS PubMed;
(e) H. Shen and Z. Xie, Chem. Commun., 2009, 2431 RSC;
(f) Z. Qiu, S. Ren and Z. Xie, Acc. Chem. Res., 2011, 44, 299 CrossRef CAS PubMed.
-
(a) L. Deng and Z. Xie, Coord. Chem. Rev., 2007, 251, 2452 CrossRef CAS PubMed;
(b) L. Deng and Z. Xie, Organometallics, 2007, 26, 1832 CrossRef CAS;
(c) J. Zhang and Z. Xie, Chem. – Asian J., 2010, 5, 1742 CrossRef CAS PubMed;
(d) J. Zhang and Z. Xie, Pure Appl. Chem., 2013, 85, 661 CAS;
(e) J. Zhang and Z. Xie, Acc. Chem. Res., 2014, 47, 1623 CrossRef CAS PubMed.
- A. Burke, D. Ellis, B. T. Giles, B. E. Hodson, S. A. Macgregor, G. M. Rosair and A. J. Welch, Angew. Chem., Int. Ed., 2003, 42, 225 CrossRef CAS PubMed.
- L. Deng, H.-S. Chan and Z. Xie, Angew. Chem., Int. Ed., 2005, 44, 2128 CrossRef CAS PubMed.
- L. Deng, H.-S. Chan and Z. Xie, J. Am. Chem. Soc., 2006, 128, 5219 CrossRef CAS PubMed.
- L. Deng, J. Zhang, H.-S. Chan and Z. Xie, Angew. Chem., Int. Ed., 2006, 45, 4309 CrossRef CAS PubMed.
- R. D. McIntosh, D. Ellis, G. M. Rosair and A. J. Welch, Angew. Chem., Int. Ed., 2006, 45, 4313 CrossRef CAS PubMed.
- J. Zhang, L. Deng, H.-S. Chan and Z. Xie, J. Am. Chem. Soc., 2007, 129, 18 CrossRef CAS PubMed.
- F. Zheng, J. Zhang, X. Fu and Z. Xie, Chem. – Asian J., 2013, 8, 1886 CrossRef CAS PubMed.
- J. Zhang, F. Zheng and Z. Xie, Organometallics, 2013, 32, 7399 CrossRef CAS.
- F. Zheng and Z. Xie, Dalton Trans., 2014, 43, 4986 RSC.
- J. Zhang and Z. Xie, Chin. J. Chem., 2014, 32, 777 CrossRef CAS.
- For the recent synthesis of a 16-vertex metallaborane, see: D. K. Roy, S. K. Bose, R. S. Anju, B. Mondal, V. Ramkumar and S. Ghosh, Angew. Chem., Int. Ed., 2013, 52, 3222 CrossRef CAS PubMed.
- X. Fu, H.-S. Chan and Z. Xie, J. Am. Chem. Soc., 2007, 129, 8964 CrossRef CAS PubMed.
- J. Zhang, H.-S. Chan and Z. Xie, Angew. Chem., Int. Ed., 2008, 47, 9447 CrossRef CAS PubMed.
- J. Zhang, F. Zheng, H.-S. Chan and Z. Xie, Inorg. Chem., 2009, 48, 9786 CrossRef CAS PubMed.
- J. Zhang, H.-S. Chan and Z. Xie, Chem. Commun., 2011, 47, 8082 RSC.
- J. Zhang and Z. Xie, Inorg. Chem., 2012, 51, 12976 CrossRef CAS PubMed.
- J. Zhang and Z. Xie, Inorg. Chem., 2013, 52, 10677 CrossRef CAS PubMed.
- N. Matsumi, M. Miyamoto and K. Aoi, J. Organomet. Chem., 2009, 694, 1612 CrossRef CAS PubMed.
- C. E. Willans, C. A. Kilner and M. A. Fox, Chem. – Eur. J., 2010, 16, 10644 CrossRef CAS PubMed.
- F. Zheng and Z. Xie, Dalton Trans., 2012, 41, 12907 RSC.
- Y. Chu, H. Deng and J. Cheng, J. Org. Chem., 2007, 72, 7790 CrossRef CAS PubMed.
- E. M. Higgins, J. A. Sherwood, A. G. Lindsay, J. Armstrong, R. S. Massey, R. W. Alder and A. C. O'Donoghue, Chem. Commun., 2011, 47, 1559 RSC.
- Y. Wang, B. Quillian, P. Wei, C. S. Wannere, Y. Xie, R. B. King, H. F. Schaefer, P. v. R. Schleyer and G. H. Robinson, J. Am. Chem. Soc., 2007, 129, 12412 CrossRef CAS PubMed.
- K. Fujita, S. Hikichi, M. Akita and Y. Moro-oka, J. Chem. Soc., Dalton Trans., 2000, 1255 RSC.
Footnote |
† Electronic supplementary information (ESI) available: Detailed experimental procedures, complete characterization data of 1–13, and crystallographic data in the CIF format for 1, 3–5, 9–11. CCDC 1032166–1032172. For ESI and crystallographic data in CIF or other electronic format see DOI: 10.1039/c4qo00287c |
|
This journal is © the Partner Organisations 2015 |
Click here to see how this site uses Cookies. View our privacy policy here.