DOI:
10.1039/C4QO00338A
(Research Article)
Org. Chem. Front., 2015,
2, 312-318
Palladium nanoparticles in glycerol: a clear-cut catalyst for one-pot multi-step processes applied in the synthesis of heterocyclic compounds†
Received
19th December 2014
, Accepted 29th January 2015
First published on 30th January 2015
Abstract
Palladium nanoparticles immobilised in a glycerol phase have been successfully applied in multi-step syntheses of heterocycles by a one-pot/one catalytic precursor approach. Actually, two- and three-component carbonylative couplings followed by an intramolecular cyclisation led to N-substituted (na)phthalimides, isoindole-1-ones and tetrahydroisoquinolin-1,3-diones in high isolated yields. 2-Benzofurans and dihydrobenzofurans could be likewise obtained by Cu-free Sonogashira coupling/hetero-cyclisation tandem processes. Drawing on the dual homogeneous/heterogeneous catalytic behaviour of PdNPs, sequential coupling/cyclisation/hydrogenation transformations were efficiently carried out, without isolation of intermediates. The Pd-based catalytic glycerol phase was recycled up to ten times preserving its activity and selectivity.
Introduction
Heterocycles are building blocks commonly present in a large variety of products, such as molecular materials, natural and biologically active compounds and also in regularly marketed drugs. For this reason, sustainable synthetic strategies have turned into a crucial current research. From this viewpoint, one-pot procedures involving metal-catalysed multi-step transformations represent powerful methodological means, which prevent the isolation (and purification) of intermediates leading to selective and advantageous processes.1 As very well-established, palladium is a potent tool in organic synthesis, mainly due to its high efficiency, selectivity and ability to perform a wide range of appealing transformations (C–C and C–heteroatom bond formation reactions, hydrogenations and carbonylations, among the most representative ones).2,3 In particular, Pd-catalysed reactions have found great success in the synthesis of heterocyclic compounds, mostly those containing nitrogen and oxygen as heteroatoms.4,5 In several reports, molecular-based palladium catalytic species have been found to be generated in situ from metallic salts in the presence of ligands (in most cases, phosphines),6 although ligand-free systems can also give the expected heterocycles, often using relatively high amounts of catalysts (higher than 2 mol%) and/or hazardous solvents.7
With the aim to recycle the catalytic phase, Pd-supported catalytic systems have led to the synthesis of heterocyclic compounds, mainly on carbon8 and carbon nanotubes.9 However, few studies using preformed palladium nanoparticles (PdNPs) have been applied in this frame.10,11
Looking for versatile Pd-based catalysts for the preparation of heterocycles, we decided to use the palladium catalytic system based on metal nanoparticles dispersed in neat glycerol (PdNP, stabilised by TPPTS, meaning Tris(3-sulfoPhenyl)Phosphine TriSodium salt), upon previously proving their efficiency and recyclability in C–C cross-couplings, C–heteroatom bond formations and C
C bond hydrogenations.12 Actually, we have recently reported the application of metal nanoparticles homogeneously dispersed in neat glycerol (based on Pd and Cu2O) in several organic transformations.12,13 This eco-friendly solvent offers, among others, the significant advantage to suitably immobilise the catalyst, allowing an easy recycling of the catalytic phase.14
To the best of our knowledge, preformed PdNPs immobilised in a liquid phase have never used before this present work in the synthesis of heterocycles. We then planned to synthesise N- and O-containing heterocycles (Fig. 1), by means of Pd-catalysed one-pot protocols in glycerol medium.
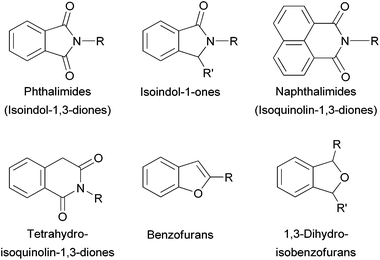 |
| Fig. 1 Heterocyclic compounds synthesised through Pd-catalysed one-pot procedures. | |
Results and discussion
Pd-catalysed carbonylation/intramolecular amination domino processes
Both N-substituted indol-1,3-diones and isoquinolin-1,3-diones find many interesting applications in different fields such as medicinal chemistry and materials (e.g. polymers, fluorosensors).4c Phthalimides and naphthalimides are conventionally prepared via condensation of the corresponding anhydrides with primary amines, often requiring harsh conditions especially when steric hindered alkyl amines are involved.15 Since the pioneering work of Heck concerning Pd-catalysed carbonylations,16 many groups have applied this strategy in particular for the synthesis of heterocyclic compounds.4c Some important studies were on homogeneous catalysts based on the assembly of Pd(II) salts and phosphines in usual organic solvents.17 More recently Alper had employed phosphonium-based ionic liquids as solvents, recycling only twice the catalytic phase.18 Bhanage found efficient supported catalytic systems based on Pd-NHC complexes on polymers19a and palladium salts/imidazolium ionic liquids on silica,19b for the synthesis of phthalimides, allowing catalyst recycling up to four times. The heterogeneous Pd/C catalyst was also efficient under harsh conditions, showing in addition the advantage of its recyclability (up to 8 times).19c However, preformed non-supported PdNPs dispersed in a liquid phase for the synthesis of heterocycles, have not previously reported.10
Benzylamine and carbon monoxide in the presence of DABCO using PdNP in glycerol as a catalyst were chosen as benchmark conditions in order to evaluate the nature of substrates in the synthesis of N-substituted phthalimides (Scheme 1 and Scheme S1 in the ESI† for the synthesis of PdNP). No important differences were found using the most typical starting materials, 2-iodobenzamide (b), methyl 2-iodobenzoate (c) and 1,2-diiodobenzene (d). Bromo-aryl derivatives, such as 2-bromoiodobenzene (e) and 1,2-dibromobenzene (f) were not active. But by using 2-iodobenzoic acid (a), a more convenient and cheaper substrate, the efficiency of the catalytic system was preserved. It is important to underline the low CO pressure (0.5 bar) and high activity of the catalytic system (full conversion was achieved in 0.5 h) to give 98% yield of a1. The catalytic phase was reused up to 10 times without loss of activity, isolating the phthalimide a1 with yields ranging between 94 and 98%, by extraction with dichloromethane from the glycerol phase (Fig. 2). The catalytic solution remained stable after recycling as evidenced by TEM analysis (see Fig. S1 in the ESI†). No palladium was detected by ICP-AES analyses for the isolated products obtained after each run.
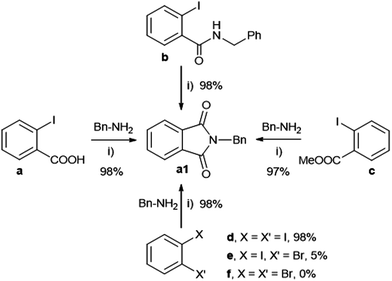 |
| Scheme 1 Pd-catalysed carbonylative cyclisation for the synthesis of a1. Reaction conditions: (i) 2.5 mol% PdNP, glycerol, 0.5 bar CO, DABCO, 120 °C, 0.5 h. | |
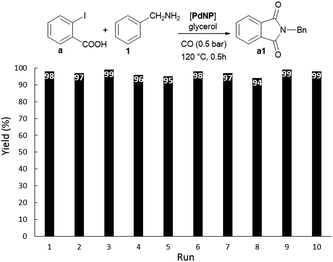 |
| Fig. 2 Pd-catalysed aminocarbonylation of 2-iodobenzoic acid and benzylamine. Diagram showing the recycling of the catalytic phase. Figures indicate the isolated yield of a1 after each run. | |
We then extended the scope of this protocol for the synthesis of N-substituted phthalimides using different primary amines (Table 1). Heterocycles with yields higher than 90% were obtained: benzyl- (a1–a8) and alkyl- (a9–a16) derivatives, including bulky primary amines (a11, a15–a16). The catalytic system was also compatible with different functions, such as alkynes (a17), alkenes (a18–a21), amino- (a22–a23) and oxygen-based (a8, a24–a28) functionalities. Some optically pure amines were also used as reagents (4, 6, 13, 16); no racemization was observed under the catalytic conditions employed (as shown by HPLC analysis of a4; see ESI†).
Table 1 Pd-catalysed three-component carbonylative cyclisations for the synthesis of N-substituted isoindol-1,3-diones (a1–a28) and isoquinolin-1,3-diones (g derivatives)a
Reaction conditions: 1 mL of glycerol using a iodo-based substrate/amine/palladium ratio = 40/40/1. Yields refer to isolated products. See Table S1 for reagents and product structures.
|
|
This Pd-catalysed three-component reaction was also successfully applied in the synthesis of 1,8-naphthalimides (g6, g8–9, g12, g16 and g24, Table 1), using 1,8-diiodonaphthalene as a substrate, commonly employed in the literature.20
Unfortunately, the PdNP catalytic system was not active when anilines were involved as nucleophiles.
Polyheterocyclic compounds could be also synthesised from bis(amines) 29–31 and tris-benzylic amine 32 by reaction with 2-iodobenzoic acid, giving the corresponding bis- (a29–a31) and tris-phthalimides (a32) in high yields (90–98%) (see Fig. 3 and Scheme S2† for their syntheses).
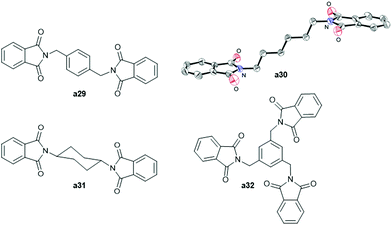 |
| Fig. 3 Poly-phthalimides a29–a32 synthesised by one-pot Pd-catalysed aminocarbonylation. For a30, a molecular view (ellipsoids drawn at 50% probability) of its X-ray diffraction structure is given; hydrogen atoms are omitted for clarity. | |
Preformed substrates (h–k) allowed the synthesis of isoindole-1-ones (h8, i9) and tetrahydroisoquinoline-1,3-diones (j9, k24) through a Pd-catalysed two-component carbonylative cyclisation process under the same catalytic conditions described above for the preparation of (na)phthalimides (Scheme 2).
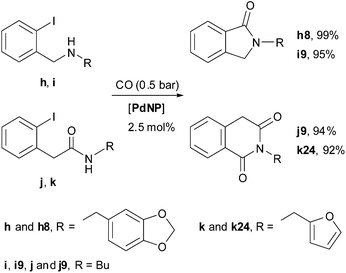 |
| Scheme 2 Pd-catalysed two-component carbonylative cyclisations for the synthesis of N-substituted isoindole-1-ones (h8, i9) and tetrahydroisoquinolin-1,3-diones (j9, k24). Reaction conditions: glycerol, DABCO, 120 °C, 0.5 h. | |
Pd-catalysed C–C Sonogashira cross-coupling/intramolecular cyclisation domino processes
The synthesis of highly substituted heterocyclic compounds represents an intense research axis due to their presence in commonly used natural compounds and drugs.21 Several metal-catalysed methodologies have been developed including domino Cu-catalysed tandem reactions,22 Sonogashira carbonylative couplings in ionic liquids23 or Heck/Suzuki–Miyaura domino processes,24 also under microwave activations.25
In this study, we planned the synthesis of N-substituted 3-(arylmethylene)isoindolin-1-ones through Pd-catalysed Cu-free Sonogashira coupling followed by intramolecular cyclisation, under CO-free conditions starting from 2-halobenzamides and the corresponding terminal alkynes (Scheme 3).26
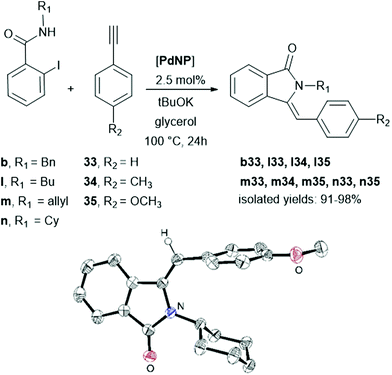 |
| Scheme 3 Pd-catalysed Sonogashira coupling followed by intramolecular amination for the synthesis of (Z)-3-(arylmethylene)isoindolin-1-ones. For n35, a molecular view (ellipsoids drawn at 50% probability) of its X-ray diffraction structure is given; hydrogen atoms are omitted for clarity, except that involved in the exocyclic C C bond. | |
The heterocyclic compounds were isolated in yields higher than 90%, exclusively giving the Z isomer, proved by the X-ray diffraction structure of n35. These results are in agreement with those reported using Pd/Cu-catalysed C–C coupling/heteroannulation domino reactions, demonstrating that after the C–C coupling, the alkynyl compound triggers an anti-addition leading to the corresponding heterocycle.27,28
Following the same domino strategy but using 2-iodo benzyl alcohols (o–r) and phenols (s, t) instead as substrates, PdNP in glycerol efficiently gave the regio- and stereo-selective synthesis of (Z)-1-methylene-1,3-dihydroisobenzofurans (Scheme 4) and 2-substituted benzofurans (Table 2), respectively. The diiodo-benzyl alcohol r led to the bifunctional compound r33 using the same catalyst; its X-ray diffraction structure also confirmed the (Z)-stereochemistry (Scheme 4).
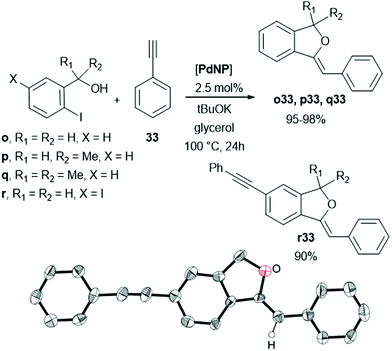 |
| Scheme 4 Pd-catalysed Sonogashira coupling followed by intramolecular amination for the synthesis of (Z)-1-methylene-1,3-dihydroisobenzofurans. For r33, a molecular view (ellipsoids drawn at 50% probability) of its X-ray diffraction structure is given; hydrogen atoms are omitted for clarity, except that involved in the exocyclic C C bond. | |
Table 2 Synthesis of 2-substituted benzofurans (s33, s35–s40, t33–t36 and t39–t40) by Pd-catalysed Sonogashira coupling followed by intramolecular cyclisationa
Reaction conditions: 1 mL of glycerol using a iodo-based substrate/alkyne/palladium ratio = 40/40/1. Yields refer to isolated products.
Iodo-based substrate/alkyne/palladium ratio = 80/40/1. See Table S2 for reagents and product structures.
|
|
In relation to benzofurans (Table 2), it is important to note the behaviour observed using 1,7-octadiyne (40); the relative ratio of the phenol s and dialkyne 40 (s/40 = 1/1 or s/40 = 2/1), gave the alkyne-benzofuran s40 or the bis(benzofuran) s40s, respectively (Table 2). This selectivity permitted to isolate s40 in high yield, which in turn could be involved in the synthesis of polyheterocyclic compounds such as s40u (Scheme 6).
Drawing on the versatility and robustness exhibited by PdNP in glycerol together with its compatibility vis-à-vis different kinds of reagents, we carried out a one-pot methodology for the synthesis of a polyfunctional molecule containing two different heterocycles by one-pot sequential processes: carbonylation/intramolecular amination followed by a Sonogashira coupling (Scheme 5). Actually, the bis(heterocyclic) a17s was selectively obtained, without isolation of the phthalimide intermediate (a17).
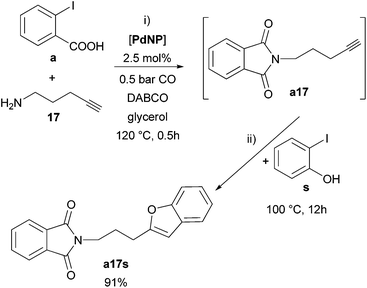 |
| Scheme 5 Synthesis of the bis(heterocyclic) phthalimide/benzofuran compound a17s by a one-pot sequential process catalysed by PdNP in glycerol. | |
With the same aim on mind, we coupled two different catalysts in the same medium, PdNP and Cu2ONP,12,13 which gave bis(heterocyclic) compounds bearing a benzofuran or phthalimide moiety and a triazole group, without the isolation of the corresponding intermediates a17 and s40 (Scheme 6). Both compounds were obtained in excellent yields and fully characterised, including their X-ray diffraction structures.
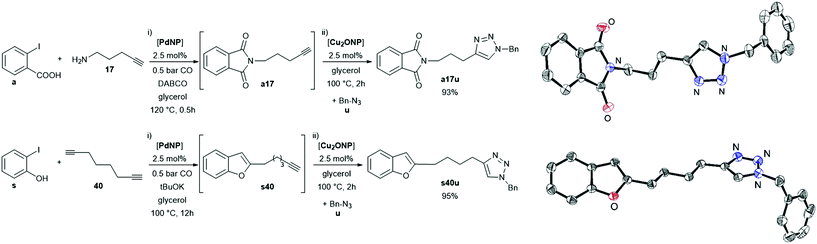 |
| Scheme 6 Pd- and Cu-catalysed one-pot multi-step sequential processes for the synthesis of polyheterocyclic compounds, a17u and s40u. Molecular views (ellipsoids drawn at 50% probability) of their X-ray diffraction structures are given; hydrogen atoms are omitted for clarity. | |
Pd-catalysed heterocycle formation followed by hydrogenation
After proving the efficiency of PdNP in glycerol in the synthesis of different kinds of heterocycles by C–C bond formations and heteroannulations, we decided to use the same catalytic system in one-pot sequential processes involving a hydrogenation step, taking advantage of the dual catalytic reactivity exhibited by MNPs dispersed in a liquid phase, i.e. their molecular- and surface-like reactivity behaviour.12,29
For that, we carried out the sequential coupling/cyclisation/hydrogenation process, using PdNP as a single catalytic precursor (Scheme 7). Therefore, upon formation of the phthalimides a18–a21, the system was pressurised with dihydrogen (1 bar) without isolation of the phthalimide intermediates; the corresponding hydrogenated compounds a18H–a21H were obtained in high yields (>90%) (Scheme 7a).
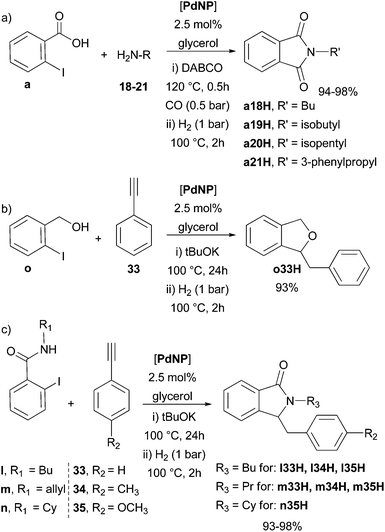 |
| Scheme 7 One-pot coupling/cyclisation/hydrogenation multi-step processes catalysed by PdNP in glycerol. | |
Following the same approach, 1-benzyl-1,3-dihydroisobenzofuran (o33H) and the 3-(4-substituted-benzyl)isoindolin-1-one derivatives l33H–l35H, m33H–m35H and n35H were efficiently prepared (Scheme 7b and 7c).
Conclusions
To sum up, we efficiently applied PdNPs dispersed in neat glycerol and stabilised by TPPTS in a large panel of reaction conditions, leading to the synthesis of heterocyclic compounds: (na)phthalimides, isoindole-1-ones, tetrahydroisoquinolin-1,3-diones, (Z)-3-(arylmethylene)isoindolin-1-one and (Z)-1-methylene-1,3-dihydroisobenzofurans. One-pot tandem and/or sequential methodologies gave the desired products without the isolation of the generated intermediates. Furthermore, compounds containing two heterocycles could be isolated, even using in the same medium two different catalysts, Pd and Cu2O based nanoparticles. The Pd-based glycerol phase could be recycled up to ten times without loss of reactivity.
Applications of palladium nanocatalysts stabilised by chiral agents in enantioselective processes are currently underway.
Acknowledgements
Financial support from the Centre National de la Recherche Scientifique (CNRS) and the Université Paul Sabatier are gratefully acknowledged. F. C. thanks the CNRS and Région Midi-Pyrénées for a PhD grant.
Notes and references
- For selected contributions, see:
(a) K. C. Nicolaou and J. S. Chen, Chem. Soc. Rev., 2009, 38, 2993 RSC;
(b) M. J. Climent, A. Corma and S. Iborra, ChemSusChem, 2009, 2, 500 CrossRef CAS PubMed;
(c) D. E. Fogg and E. N. dos Santos, Coord. Chem. Rev., 2004, 248, 2365 CrossRef CAS PubMed.
- For representative books, see:
(a)
Palladium in Heterocyclic Chemistry. A guide for the Synthetic Chemist, ed. J. J. Li and G. W. Gribble, 2nd edn, Elsevier, Oxford, 2007 Search PubMed;
(b)
J. Tsuji, Palladium reagents and catalysts, John Wiley & Sons, Sussex, 2004 Search PubMed.
- In 2010, R. Heck, A. Suzuki and E. Negishi were awarded with the Nobel Price for their research on palladium-catalysed cross-couplings in organic synthesis:
(a) A. Suzuki, Angew. Chem., Int. Ed., 2011, 50, 6722 CrossRef CAS PubMed;
(b) E. Negishi, Angew. Chem., Int. Ed., 2011, 50, 6738 CrossRef CAS PubMed;
(c) X.-F. Wu, P. Anbarasan, H. Neumann and M. Beller, Angew. Chem., Int. Ed., 2010, 49, 9047 CrossRef CAS PubMed.
- For selected reviews concerning the synthesis of heterocyclic compounds through Pd-catalysed processes, see:
(a) N. Kaur, Inorg. Chem. Commun., 2014, 49, 86 CrossRef CAS PubMed;
(b) C. J. Ball and M. C. Willis, Eur. J. Org. Chem., 2013, 425 CrossRef CAS;
(c) X.-F. Wu, H. Neumann and M. Beller, Chem. Rev., 2013, 113, 1 CrossRef CAS PubMed;
(d) S. Arseniyadis, J. Fournier, S. Thangavelu, O. Lozano, S. Prevost, A. Archambeau, C. Menozzi and J. Cossy, Synlett, 2013, 2350 CrossRef CAS PubMed;
(e) M. Platon, R. Amardeil, L. Djakovitch and J.-C. Hierso, Chem. Soc. Rev., 2012, 41, 3929 RSC;
(f) S. Cacchi and G. Fabrizi, Chem. Rev., 2005, 105, 2873 CrossRef CAS PubMed ; update of this contribution: Chem. Rev., 2011, 111, PR215.
- For a selected review concerning the applications of palladium nanoparticles in organic synthesis, see: I. Favier, D. Madec, E. Teuma and M. Gómez, Curr. Org. Chem., 2011, 15, 3127 CrossRef CAS and references therein.
- For an elegant way of synthesis of benzofurans catalysed by preformed Pd-NHC complexes, see: A. Zanardi, J. A. Mata and E. Peris, Organometallics, 2009, 28, 4335 CrossRef CAS.
- For some recent representative contributions, see:
(a) Y. Wang, B. Frett and H.-y. Li, Org. Lett., 2014, 16, 3016 CrossRef CAS PubMed;
(b) F. Jei, M.-f. Lv, W.-b. Yi and C. Cai, Synthesis, 2013, 1965 Search PubMed;
(c) J. Ghosh, P. Biswas, S. Maiti, T. Sarkar, M. G. B. Drew and C. Bandyopadhyay, Tetrahedron Lett., 2013, 54, 2221 CrossRef CAS PubMed;
(d) D. A. Barancelli, A. G. Salles Jr., J. G. Taylor and C. R. D. Correia, Org. Lett., 2012, 14, 6036 CrossRef CAS PubMed;
(e) S. K. Sahoo, A. Banerjee, S. Chakraborty and B. K. Patel, ACS Catal., 2012, 2, 544 CrossRef CAS.
-
(a) E. K. Yum, O.-K. Yang, J.-E. Kim and H. J. Park, Bull. Korean Chem. Soc., 2013, 34, 2645 CrossRef CAS;
(b) M. Pal, V. Subramanian and K. R. Yeleswarapu, Tetrahedron Lett., 2003, 44, 8221 CrossRef CAS PubMed.
-
(a) S. Santra, P. Ranjan, P. Bera, P. Ghosh and S. K. Mandal, RSC Adv., 2012, 2, 7523 RSC;
(b) L. Zhou and H.-F. Jiang, Tetrahedron Lett., 2007, 48, 8449 CrossRef CAS PubMed.
- For PdNPs supported on polymers
applied in the synthesis of heterocycles, see: I. P. Beletskaya, A. N. Kashin, A. E. Litvinov, V. S. Tyurin, P. M. Valetsky and G. van Koten, Organometallics, 2006, 25, 154 CrossRef CAS.
- In some contributions, palladium nanoparticles were evidenced during or at the end of the process starting from molecular palladium species, see:
(a) D. Saha, R. Dey and B. C. Ranu, Eur. J. Org. Chem., 2010, 6067 CrossRef CAS;
(b) S. S. Palimkar, V. S. More and K. V. Srinivasan, Ultrason. Sonochem., 2008, 15, 853 CrossRef CAS PubMed;
(c) R. Grigg, L. Zhang, S. Collard and A. Keep, Tetrahedron Lett., 2003, 44, 6979 CrossRef CAS.
- F. Chahdoura, C. Pradel and M. Gómez, Adv. Synth. Catal., 2013, 355, 3648 CrossRef CAS.
- F. Chahdoura, C. Pradel and M. Gómez, ChemCatChem, 2014, 6, 2929 CrossRef CAS.
- For selected reviews, see:
(a) F. Chahdoura, I. Favier and M. Gómez, Chem. – Eur. J., 2014, 20, 10884 CrossRef CAS PubMed;
(b) A. E. Díaz-Alvarez, J. Francos, B. Lastra-Barreira, P. Crochet and V. Cadierno, Chem. Commun., 2011, 47, 6208 RSC;
(c) Y. Gu and F. Jérôme, Green Chem., 2010, 12, 1127 RSC;
(d) A. Corma, S. Iborra and A. Velty, Chem. Rev., 2007, 107, 2411 CrossRef CAS PubMed.
- For a representative example, see: M. Sani, G. Fossati, F. Huguenot and M. Zanda, Angew. Chem., Int. Ed., 2007, 46, 3526 CrossRef CAS PubMed.
- A. Schoenberg and R. F. Heck, J. Am. Chem. Soc., 1979, 96, 7761 CrossRef , and references therein.
- For recent reviews, see:
(a) J. Cossy, Pure Appl. Chem., 2010, 82, 1365 CrossRef CAS;
(b) Y. Yamamoto, Chem. Soc. Rev., 2014, 43, 1575 RSC.
- H. Cao and H. Alper, Org. Lett., 2010, 12, 4126 CrossRef CAS PubMed.
-
(a) M. V. Khedkar, S. R. Khan, K. P. Dhake and B. M. Bhanage, Synthesis, 2012, 2623 CAS;
(b) M. V. Khedkar, A. R. Shinde, T. Sasaki and B. M. Bhanage, J. Mol. Catal. A: Chem., 2014, 385, 91 CrossRef CAS PubMed;
(c) M. V. Khedkar, S. R. Khan, D. N. Sawant, D. B. Bagal and B. M. Bhanage, Adv. Synth. Catal., 2011, 353, 3415 CrossRef CAS.
- For a representative contribution, see: A. Takács, P. Acs and L. Kollár, Tetrahedron, 2008, 64, 983 CrossRef PubMed.
- For a selected contribution related to isoindolinones, see: M. Efdi, S. Fujita, T. Inuzuka and M. Koketsu, Nat. Prod. Res., 2010, 24, 657 CrossRef CAS PubMed.
- L. Li, M. Wang, X. Zhang, Y. Jiang and D. Ma, Org. Lett., 2009, 11, 1309 CrossRef CAS PubMed.
- H. Cao, L. McNamee and H. Alper, Org. Lett., 2008, 10, 5281 CrossRef CAS PubMed.
-
(a) S. Couty, B. Liegault, C. Meyer and J. Cossy, Tetrahedron, 2006, 62, 3882 CrossRef CAS PubMed.
- M. Hellal and G. D. Cuny, Tetrahedron Lett., 2011, 52, 5508 CrossRef CAS PubMed.
- For Cu-free Sonogashira couplings applied in the synthesis of heterocycles, see the following selected references:
(a) S. Sarkar, S. Dutta, R. Dey and S. Naskar, Tetrahedron Lett., 2012, 53, 6789 CrossRef CAS PubMed;
(b) R. Chinchilla and C. Nájera, Chem. Soc. Rev., 2011, 40, 5084 RSC.
- For the synthesis of N-substituted (Z)-3-(arylmethylene)isoindolin-1-ones by Pd/Cu-catalysed heteroannulation with terminal alkynes, see: N. G. Kundu and M. W. Khan, Tetrahedron, 2000, 56, 4777 CrossRef CAS.
- For the synthesis of N-substituted (E)-3-(arylmethylene)isoindolin-1-ones, see: J. W. Wrigglesworth, B. Cox, G. Lloyd-Jones and K. I. Booker-Milburn, Org. Lett., 2011, 13, 5326 CrossRef CAS PubMed and ref. 24.
-
(a) J. Durand, E. Teuma and M. Gómez, Eur. J. Inorg. Chem., 2008, 3577 CrossRef CAS;
(b) D. Astruc, Inorg. Chem., 2007, 46, 1884 CrossRef CAS PubMed.
Footnote |
† Electronic supplementary information (ESI) available: Experimental procedures, copies of NMR spectra and X-ray diffraction data. CCDC 1020806–1020812. For ESI and crystallographic data in CIF or other electronic format see DOI: 10.1039/c4qo00338a |
|
This journal is © the Partner Organisations 2015 |
Click here to see how this site uses Cookies. View our privacy policy here.