DOI:
10.1039/C5QO00040H
(Research Article)
Org. Chem. Front., 2015,
2, 726-729
Nickel-catalyzed decarboxylative arylation of azoles with perfluoro- and nitrobenzoates†
Received
31st January 2015
, Accepted 15th April 2015
First published on 12th May 2015
Abstract
This manuscript describes a Ni-catalyzed method for the direct arylation of azoles using benzoates. Perfluorophenyl and 2-nitrobenzoates participate in these reactions to afford the corresponding products in modest to good yields. The efficiency of the arylations with perfluorobenzoates is highly dependent on both the degree and position of fluorine atoms in the benzoates.
Transition metal catalyzed arylation of aromatic C–H bonds is widely used for the construction of biaryl scaffolds.1 The vast majority of these transformations entail the coupling of C–H bonds with aryl halides (or pseudohalides) or organometallic reagents. In recent years there has been interest in the development of transformations that replace the halides or organometallic reagents in these arylations with carboxylic acids.2 The use of carboxylic acids obviates the need for the preparation and use of often sensitive organometallic reagents. Additionally, structurally diverse benzoic acid derivatives are readily available and bench-stable compounds.
Despite these advantages, decarboxylative cross couplings have developed at a relatively slower rate because of the general requirement for harsh conditions for the extrusion of CO2.2 The first examples of decarboxylative biaryl bond formation involved the use of Pd catalysis.2,3 Following these seminal reports, several reports on decarboxylative biaryl formations have been published. Most of these methods employ expensive transition metals such as Pd.2–4 As such there is an increasing demand for the replacement of noble metals (e.g., Pd) with their earth-abundant counterparts (e.g., Ni).5–8 To this end, efforts have been made to use Ni catalysts in decarboxylative transformations. However, reports of Ni-catalyzed decarboxylative synthesis of biaryl motifs remain sparse.9–12 Herein, we describe a method for the Ni-catalyzed intermolecular coupling of perfluorobenzoates with azoles for the synthesis of biaryl compounds. A systematic exploration of the efficiency of these arylations with penta-, tetra- tri- and difluorinated benzoates is presented. These transformations can also be applied toward the coupling of benzoxazoles with 2-nitrobenzoate.
We commenced our studies with the investigation of reaction parameters for the coupling of 5-methylbenzoxazole with pentafluorobenzoate. This reaction serves as a good starting point because Ni-catalyzed C–H activation of acidic C–H bonds in azole substrates has been achieved previously.9 Furthermore, perfluoroaryl carboxylates are known to extrude CO2 at moderate temperatures.13 A plausible mechanism for the proposed Ni-catalyzed C–H arylation based on literature reports of analogous Pd-catalyzed reactions is depicted in Scheme 1.2 It involves: (i) base-assisted C–H nickelation, (ii) transmetallation between (I) and Ar'M (generated upon decarboxylation), (iii) reductive elimination to release the desired product and (iv) oxidation of Ni0 to regenerate the NiII catalyst. Importantly, the decarboxylation and oxidation steps are commonly promoted by the addition of Cu or Ag salts in Pd-catalyzed transformations.2 Furthermore, diglyme has been the solvent of choice for a number of transformations involving decarboxylation of perfluorobenzoate potassium salts.13
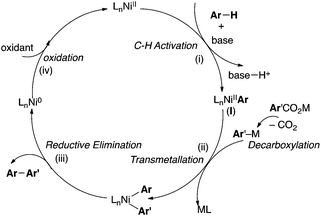 |
| Scheme 1 Plausible mechanism for C–H arylation. | |
As such we began our optimizations using the reaction conditions shown in Scheme 2. Several catalysts, oxidants, bases, solvents and temperatures were screened to optimize the yield of the transformation. However, product 1a is obtained in at most 41% yield. Importantly, only trace amounts (<10%) of 1a is formed in the absence of Ni(OTf)2 under otherwise optimal conditions suggesting that the Ni catalyst is necessary for the transformation to proceed. The low yield (41%) of 1a under the Ni-catalyzed reaction conditions is partly due to the homo-coupling of both the azole and the carboxylate substrates under the reaction conditions. These observations are consistent with a fundamental challenge associated with decarboxylative C–H arylations. Striking the optimal balance between the relative rates of C–H metallation (Scheme 1, step i) and the decarboxylation (Scheme 1, step ii) is essential toward preventing the undesired homo-coupling of the aryl-metal intermediates generated upon C–H nickelation (I) and decarboxylation (Ar'M) (Scheme 1). Previous reports on Pd and Cu-catalyzed decarboxylative couplings suggest that the relative rate of decarboxylation is highly dependent on the electronic nature of the carboxylate.2,13 As such, we next explored the use of diverse perfluorobenzoates under the optimal conditions for the formation of 1a.
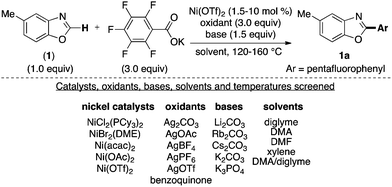 |
| Scheme 2 Optimization of decarboxylative arylation. | |
As shown in Scheme 3, tetra-, tri- and difluorinated salts participate in this transformation to afford the corresponding perfluorobiaryl products. The temperature and the solvent were optimized for each salt individually. The efficiency of the transformation is highly dependent on both the degree of fluorination and the relative positions of the fluorine atoms in the carboxylate salts. For example the trifluorinated product 1f is obtained in higher yields than the tetra and pentafluorinated products 1a and 1b. Furthermore, under the same reaction conditions (catalyst loading, temperature and solvent) the 2,3,4-trifluorobenzoate salt affords the corresponding biaryl (1f) in higher yield than the 2,3,6- and 2,4,6-trifluorobenzoate salts (cf. yields in parentheses). Notably, the chlorinated product 1h is also obtained in modest yield. The C–Cl bond in 1h can be further elaborated using a myriad of known cross-coupling reactions.14
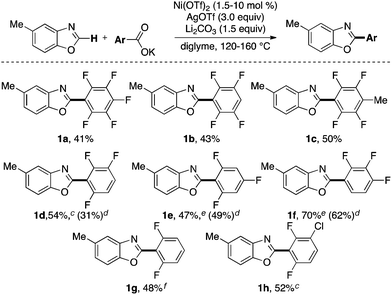 |
| Scheme 3 Scope of perfluorobenzoates: a,bConditions: azole (1.0 equiv.), benzoate (3.0 equiv.), Ni(OTf)2 (0.015 equiv.), AgOTf (3.0 equiv.), Li2CO3 (1.5 equiv.), diglyme, 120 °C, 20 h. Isolated yields. c General conditions with DMA/diglyme (1 : 4 v/v) as solvent. d Calibrated GC yields against hexadecane as the internal standard using general conditions but in DMA/diglyme (1 : 4 v/v) as solvent, 10 mol% Ni(OTf)2 and at 160 °C. e General conditions with 5 mol% Ni(OTf)2, DMA/diglyme (1 : 4 v/v) as solvent at 160 °C. f General conditions with 10 mol% Ni(OTf)2, DMA/diglyme (1 : 4 v/v) as solvent at 140 °C. | |
The reaction conditions for these transformations represent an advance over prior methodology.10 The optimal temperature for the formation of most products (1a–1d and 1h) in Scheme 3 is 120 °C, which is significantly lower than the temperature (170 °C) used to obtain similar products from the corresponding carboxylic acids in place of potassium benzoates.10 Additionally, the transformation proceeds in the absence of any added ligands to afford products in comparable yields to those reported previously using N-heterocyclic carbene ligands.10 Furthermore, the temperatures (120–160 °C) of the reactions described herein are at or below the boiling point of the solvent which makes this methodology more synthetically convenient and safer than the previous report which employs (trifluoromethyl)benzene (b.p. = 102 °C) at 170 °C.10
As detailed in Scheme 4, the optimal conditions for the arylation of 5-methylbenzoxazole can be applied toward the use of other substituted azoles. Electron-rich (e.g., OMe) and electron-deficient substituents (e.g., NO2) are compatible with the transformation. Additionally, products bearing aryl chloride groups are obtained in modest yields. The perfluorinated biaryl motifs obtained using this methodology are prevalent in numerous pharmaceutical and material science applications.15
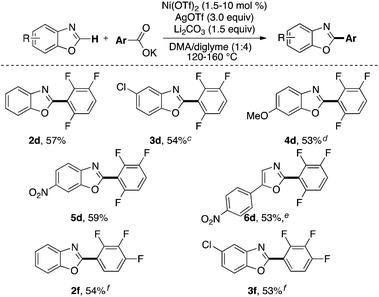 |
| Scheme 4 Scope of azoles: a,bConditions: azole (1.0 equiv.), benzoate (3.0 equiv.), Ni(OTf)2 (0.015 equiv.), AgOTf (3.0 equiv.), Li2CO3 (1.5 equiv.), DMA/diglyme (1 : 4 v/v), 120 °C, 20 h. Isolated yields. c General conditions with diglyme as solvent. d General conditions 5 mol% Ni(OTf)2. e General conditions 10 mol% Ni(OTf)2. f General conditions with 5 mol% Ni(OTf)2 at 160 °C. | |
Having explored the Ni-catalyzed decarboxylative C–H arylation using perfluorobenzoate salts, we next examined a preliminary scope for the coupling of benzoxazoles with 2-nitrobenzoate (Scheme 5). Analogous to the perfluorobenzoate salts, the 2-nitrobenzoates are known to decarboxylate under thermal conditions.2 As shown in Scheme 5, the scope of these reactions is similar to the transformations with perfluorobenzoates.
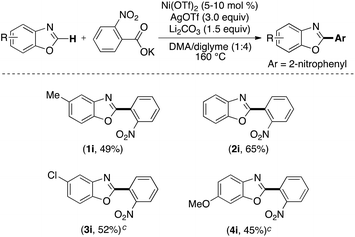 |
| Scheme 5 Coupling with 2-nitropotassiumbenzoate: a,bConditions: azole (1.0 equiv.), benzoate (3.0 equiv.), Ni(OTf)2 (0.05 equiv.), AgOTf (3.0 equiv.), Li2CO3 (1.5 equiv.), DMA/diglyme (1 : 4 v/v), 160 °C 20 h. Isolated yields. c General conditions with 10 mol% Ni(OTf)2. | |
Conclusions
In summary, this paper describes an example of Ni-catalyzed arylation of azole C–H bonds using perfluoro- and 2-nitrobenzoate salts. The efficiency of these arylations is highly dependent on the electronic nature of the carboxylate. Further explorations will focus on expanding the scope of these transformations. Additionally, efforts will be made to replace the use of silver triflate with less expensive oxidants in these transformations.
Acknowledgements
This work was supported by St. Olaf College, National Institutes of Health (R15 GM107892), and the American Chemical Society Petroleum Research Fund (PRF#52224-UNI3). The fluorine spectra for some compounds was obtained using the NMR Spectrometer (at Carleton College) supported by National Science Foundation under CHE-1428752.
Notes and references
- For representative reviews on C–H arylation, see:
(a) D. Alberico, M. E. Scott and M. Lautens, Chem. Rev., 2007, 107, 174–238 CrossRef CAS PubMed
;
(b) F. Kakiuchi and T. Kochi, Synthesis, 2008, 3013–3039 CrossRef CAS PubMed
;
(c) G. P. McGlacken and L. M. Bateman, Chem. Soc. Rev., 2009, 38, 2447–2464 RSC
;
(d) X. Chen, K. M. Engle, D.-H. Wang and J.-Q. Yu, Angew. Chem., Int. Ed., 2009, 48, 5094–5115 CrossRef CAS PubMed
;
(e) L. Ackermann, R. Vincente and A. R. Kapdi, Angew. Chem., Int. Ed., 2009, 48, 9792–9826 CrossRef CAS PubMed
;
(f) F. Bellina and R. Rossi, Tetrahedron, 2009, 65, 10269–10310 CrossRef CAS PubMed
;
(g) T. W. Lyons and M. S. Sanford, Chem. Rev., 2010, 110, 1147–1169 CrossRef CAS PubMed
;
(h) O. Daugulis, Top. Curr. Chem., 2010, 292, 57–84 CrossRef CAS
.
-
(a) L. J. Gooßen, N. Rodríguez and K. Gooßen, Angew. Chem., Int. Ed., 2008, 47, 3100–3120 CrossRef PubMed
;
(b) J. D. Weaver, A. Recio, A. J. Grenning and J. A. Tunge, Chem. Rev., 2011, 111, 1846–1913 CrossRef CAS PubMed
;
(c) N. Rodríguez and L. J. Gooßen, Chem. Soc. Rev., 2011, 40, 5030–5048 RSC
;
(d) W. I. Dzik, P. P. Lange and L. J. Gooßen, Chem. Sci., 2012, 3, 2671–2678 RSC
;
(e) L. J. Gooßen and K. Gooßen, Top. Organomet. Chem., 2013, 44, 121–141 CrossRef
;
(f) K. Park and S. Lee, RSC Adv., 2013, 3, 14165–14182 RSC
.
-
(a) A. Fromm, C. Wüllen, D. Hackenberger and L. J. Gooßen, J. Am. Chem. Soc., 2014, 136, 10007–10023 CrossRef CAS PubMed
;
(b) C. K. Haley, C. D. Gilmore and B. M. Stoltz, Tetrahedron, 2013, 69, 5732–5736 CrossRef CAS PubMed
;
(c) P. Hu, M. Zhang, X. Jie and W. Su, Angew. Chem., Int. Ed., 2012, 51, 227–231 CrossRef CAS PubMed
;
(d) K. Xie, Z. Yang, X. Zhou, X. Li, S. Wang, Z. Tan, X. An and C.-C. Guo, Org. Lett., 2010, 12, 1564–1567 CrossRef CAS PubMed
;
(e) L. J. Gooßen, N. Rodríguez, P. P. Lange and L. Christophe, Angew. Chem., Int. Ed., 2010, 49, 1111–1114 CrossRef PubMed
;
(f) F. Zhang and M. F. Greaney, Angew. Chem., Int. Ed., 2010, 49, 2768–2771 CrossRef CAS PubMed
;
(g) C. Wang, I. Piel and F. Glorius, J. Am. Chem. Soc., 2009, 131, 4194–4195 CrossRef CAS PubMed
;
(h) J. Cornella, P. Lu and I. Larrosa, Org. Lett., 2009, 11, 5506–5509 CrossRef CAS PubMed
;
(i) A. Voutchkova, A. Coplin, N. E. Leadbeater and R. H. Crabtree, Chem. Commun., 2008, 6312–6314 RSC
;
(j) A. G. Myers, D. Tanaka and M. R. Mannion, J. Am. Chem. Soc., 2002, 124, 11250–11251 CrossRef CAS PubMed
.
- For Rh and Ru-catalyzed reactions see:
(a) J. M. Neely and T. Rovis, J. Am. Chem. Soc., 2014, 136, 2735–2738 CrossRef CAS PubMed
;
(b) J. Zhang, J.-F. Liu, A. Ugrinov, A. F. X. Pillai, Z.-M. Sun and P. Zhao, J. Am. Chem. Soc., 2013, 135, 17270–17273 CrossRef CAS PubMed
;
(c) J. Wang, B. Liu, H. Zhao and J. Wang, Organometallics, 2012, 31, 8598–8607 CrossRef CAS
;
(d) Z. M. Sun, J. Zhang and P. Zhao, Org. Lett., 2010, 12, 992–995 CrossRef CAS PubMed
;
(e) M. Austeri, D. Linder and J. Lacour, Adv. Synth. Catal., 2010, 352, 3339–3347 CrossRef CAS PubMed
;
(f) M. Austeri, D. Linder and J. Lacour, Chem. – Eur. J., 2008, 14, 5737–5741 CrossRef CAS PubMed
;
(g) E. C. Burger and J. A. Tunge, Org. Lett., 2004, 6, 2603–2605 CrossRef CAS PubMed
.
-
(a) E. Nakamura, T. Hatakeyama, S. Ito, K. Ishizuka, L. Ilies and M. Nakamura, Org. React., 2014, 83, 1–209 CAS
;
(b) J. Yamaguchi, K. Muto and K. Itami, Eur. J. Org. Chem., 2013, 19–30 CrossRef CAS PubMed
;
(c) S. U. Tekale, V. B. Jadhav, V. P. Pagore, S. S. Kauthale, D. D. Gaikwad and R. P. Pawar, Mini-Rev. Org. Chem., 2013, 10, 281–301 CrossRef CAS
;
(d) G. Cahiez and A. Moyeux, Chem. Rev., 2010, 110, 1435–1462 CrossRef CAS PubMed
.
- For Ni-catalyzed decarboxylative cross-coupling for construction of bonds other than the biaryl bond see:
(a) K. Yang, C. Zhang, P. Wang, Y. Zhang and H. Ge, Chem. – Eur. J., 2014, 20, 7241–7244 CrossRef CAS PubMed
;
(b) Y. Wu, L. Liu, K. Yan, P. Xu, Y. Gao and Y. Zhao, J. Org. Chem., 2014, 79, 8118–8127 CrossRef CAS PubMed
;
(c) Z. Zuo, D. Ahneman, L. Chu, J. A. Terrett, A. G. Doyle and D. W. C. MacMillan, Science, 2014, 345, 437–440 CrossRef CAS PubMed
;
(d) J. Choe, J. Yang, K. Park, T. Palani and S. Lee, Tetrahedron Lett., 2012, 53, 6908–6912 CrossRef CAS PubMed
.
- For Cu-catalyzed decarboxylative biaryl bond formation see:
(a) T. Ponpandian and S. Muthusubramanian, Tetrahedron Lett., 2012, 53, 4248–4252 CrossRef CAS PubMed
;
(b) R. Shang, Y. Fu, Y. Wang, Q. Xu, H.-Z. Yu and L. Liu, Angew. Chem., Int. Ed., 2009, 48, 9350–9354 CrossRef CAS PubMed
.
- For Fe-catalyzed decarboxylative cross-couplings see:
(a) G. Rong, D. Liu, L. Lu, H. Yan, Y. Zheng, J. Chen and J. Mao, Tetrahedron, 2014, 70, 5033–5037 CrossRef CAS PubMed
;
(b) J. Zhao, W. Zhou, J. Han, G. Li and Y. Pan, Tetrahedron Lett., 2013, 54, 6507–6510 CrossRef CAS PubMed
;
(c) J. Zhao, H. Fang, J. Han and Y. Pan, Beilstein J. Org. Chem., 2013, 9, 1718–1723 CrossRef PubMed
;
(d) H. Yang, H. Yan, P. Sun, Y. Zhu, L. Lu, D. Liu, G. Rong and J. Mao, Green Chem., 2013, 15, 976–981 RSC
;
(e) H.-P. Bi, W.-W. Chen, Y.-M. Liang and C.-J. Li, Org. Lett., 2009, 11, 3246–3249 CrossRef CAS PubMed
.
- For Ni-catalyzed decarbonylative C–H Arylation see: K. Amaike, K. Muto, J. Yamaguchi, K. Itami, C. Zhang, P. Wang, Y. Zhang and H. Ge, J. Am. Chem. Soc., 2012, 134, 13573–13576 CrossRef CAS PubMed
.
- During the preparation of this manuscript the first report on Ni-catalyzed decarboxylative C–H arylation was published, namely K. Yang, P. Wang, C. Zhang, A. A. Kadi, K.-K. Fun, Y. Zhang and H. Lu, Eur. J. Org. Chem., 2014, 7586–7589 CrossRef CAS PubMed
.
- For Ni-catalyzed decarboxylative cross-coupling see: L. W. Sardzinski, W. C. Wertjes, A. M. Schnaith and D. Kalyani, Org. Lett., 2015, 17, 1256–1259 CrossRef CAS PubMed
.
- The key differences between ref. 10 and the method developed herein are: (1) use of benzoic acids in place of benzoates, (2) focus on synthesis of nitro substituted biaryl products rather than perfluorinated biaryls (note: the report by Zhang group provides 5 examples that employ non-nitro containing fluorinated carboxylic acids and only benzoxazole was employed as the C–H substrate for coupling with these non-nitro containing benzoic acids), (3) the reaction conditions for coupling.
- R. Shang, Q. Xu, Y.-Y. Jiang, Y. Wang and L. Liu, Org. Lett., 2010, 12, 1000–1003 CrossRef CAS PubMed
.
-
(a)
Metal-Catalyzed Cross-Coupling Reactions, ed. A. de Meijere and F. Diederich, Wiley-VCH, Weinheim, Germany, 2nd edn, 2004 Search PubMed
;
(b) C. C. C. J. Seechurn, M. O. Kitching, T. J. Colacot and V. Snieckus, Angew. Chem., Int. Ed., 2012, 51, 5062–5085 CrossRef PubMed
.
-
(a) T. Okamoto, K. Nakahara, A. Saeki, S. Seki, J. H. Oh, H. B. Akkerman, Z. Bao and Y. Matsuo, Chem. Mater., 2011, 23, 1646–1649 CrossRef CAS
;
(b) H. Amii and K. Uneyama, Chem. Rev., 2009, 109, 2119–2183 CrossRef CAS PubMed
;
(c) F. Babudri, G. M. Farinola, F. Naso and R. Ragni, Chem. Commun., 2007, 1003–1022 RSC
;
(d) E. A. Meyer, R. K. Castellano and F. Diederich, Angew. Chem., Int. Ed., 2003, 42, 1210–1250 CrossRef CAS PubMed
.
Footnotes |
† Electronic supplementary information (ESI) available: Experimental details and spectroscopic and analytical data for new compounds. See DOI: 10.1039/c5qo00040h |
‡ These authors contributed equally to this work. |
|
This journal is © the Partner Organisations 2015 |