DOI:
10.1039/C5QO00265F
(Research Article)
Org. Chem. Front., 2015,
2, 1500-1504
Open-cage fullerene with a stopper acts as a molecular vial for a single water molecule†
Received
24th August 2015
, Accepted 9th September 2015
First published on 10th September 2015
Abstract
An open-cage fullerene derivative with three carbonyl groups on the rim of the orifice reacts with o-diaminobenzene reversibly to form a tetrahydrofuran moiety above the orifice. Water encapsulation and release experiments show that the tetrahydrofuran moiety acts as a stopper effectively blocking the orifice. The addition and removal of o-diaminobenzene serve as a chemically controlled switching process for the fullerene-based water container, which is suited for just one water molecule due to its moderate cavity size.
Introduction
Molecular containers for water have attracted much attention. Various molecules have been prepared and studied as hosts for water molecules.1 In most cases the cavity of the host molecule is relatively large and water clusters bound by H-bonding can be trapped inside the cavity. Molecular containers for a single water molecule are rare. Fullerenes have a unique spherical structure and are suitable for encapsulation of just one water molecule. The water complex H2O@C60 has been successfully prepared through a molecular surgery method.2 A number of open-cage fullerenes have been prepared with a relatively large orifice,3,4 through which water can be trapped and released reversibly.5 These open-cage fullerene compounds act like a vial for a single water molecule but without a stopper since the trapped water molecule can readily exchange with water molecules outside the fullerene cage. To explore practical applications such as molecular containers for radioactive tritiated water, it is desirable to develop open-cage systems with a stopper which can be readily added and removed. We have reported a phosphate modulated open-cage derivative in which the phosphate on the rim of the orifice acts as a removable stopper.6 Here we report the preparation of a new open-cage fullerene derivative with an orifice large enough for water encapsulation and its reversible reaction with o-diaminobenzene. The results show that o-diaminobenzene acts as an effective stopper for the open-cage compound.
Results and discussion
In an effort to explore the reactivity of the functional groups on the rim of the orifice, we treated our previously reported compound 17 with o-diaminobenzene in the presence of acetic acid as the catalyst (Scheme 1).8 There is hardly any reaction without acetic acid. 1H NMR monitoring indicated that three products were formed in the process, one of which appeared to be the precursor for the other two compounds and eventually disappeared. Yields of the two isolated products 2 and 3 depend on the reaction time. Extending the reaction time favours product 3. Compound 2 slowly converts into compound 3 under the reaction conditions. The two products can be easily separated by column chromatography.
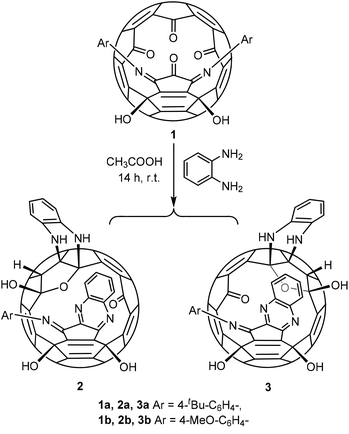 |
| Scheme 1 Preparation of compounds 2 and 3. Yields: 2a 44%; 2b 43%; 3a 33%; 3b 37%. | |
The intermediate observed in the formation of 2 and 3 is the monoaddition product 4. It was difficult to isolate 4 directly from the reaction of 1 with excess o-diaminobenzene. But treating 2 or 3 with acetic acid removed one o-diaminobenzene selectively to form 4 in yields ranging from 79% to 91% (Scheme 2). As expected, compound 4 reacts with o-diaminobenzene to form a mixture of compounds 2 and 3 under the same conditions as the reaction of 1 with o-diaminobenzene. The combined yields of the isomers 2 and 3 are 84% and 88% for 4a and 4b respectively. These yields are much improved compared to the triethylphosphate stopper system in which the removal of the phosphate is only 27% as we reported before.6
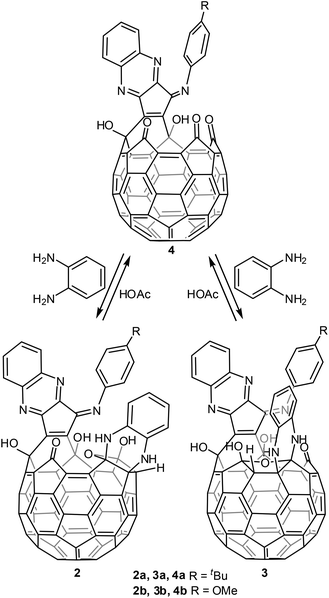 |
| Scheme 2 Reversible reactions of 4 with o-diaminobenzene. Conditions: 18 h at 80 °C in PhCl for the conversion of 2 or 3 to 4; 16 h at r.t. with CH3COOH as a catalyst in CH2Cl2 for the conversion of 4 to 2 and 3. Yields: 2a to 4a 88%; 2b to 4b 91%; 3a to 4a 88%; 3b to 4b 79%; 4a to 2a 24%; 4a to 3a 60%; 4b to 2b 29%; 4b to 3b 59%. | |
The above results indicate that in the conversion from compound 1 to 2 and 3, the o-diaminobenzene first reacted with the carbonyl group adjacent to two imino groups to form 4 with the quinoxaline moiety, followed by the formation of 2 and 3 with a tetrahydrofuran moiety. Similar to the reaction between 1 and triethylphosphite,6 the carbonyl group on the mirror plane is more reactive than the other two carbonyl groups on the rim of the orifice and probably initiates the formation of the tetrahydrofuran moiety in the formation of both isomers 2 and 3. The two double bonds connecting the three carbonyl groups on the rim of the orifice are more reactive than other double bonds on the cage towards nucleophiles as we have shown before.9 In the formation of the quinoxaline moiety, one aryl imino group was replaced. Dehydration efforts failed to remove the adjacent H and OH groups on the tetrahydrofuran moiety.
Unlike the isomeric compound 3, the solubility of 2 is quite low in common organic solvents. Crystals suitable for X-ray diffraction analysis of 2a were obtained from a mixture of hexane/CH2Cl2/CHCl3. The X-ray structure shows that 2a is racemic and 4 molecules (two pairs of enantiomers) of 2a are present in each unit cell (Fig. 1). There are strong intermolecular H-bonds in the crystal between the NH groups and OH groups, thus forming a linear chain structure as shown in Fig. 1. Two different enantiomers are adjacent to each other. The polymeric structure probably is responsible for the low solubility of 2a. Single crystals of 4a were obtained by a similar method from hexane/CH2Cl2. The structure of 4a also shows a racemic mixture with 4 molecules in each unit cell (Fig. 1). But unlike the linear chain structure in 2a, 4a forms dimeric structures through H-bonds between the OH groups of two enantiomeric isomers. A similar dimeric structure was reported previously between two open-cage molecules without the hydroxyl groups.10
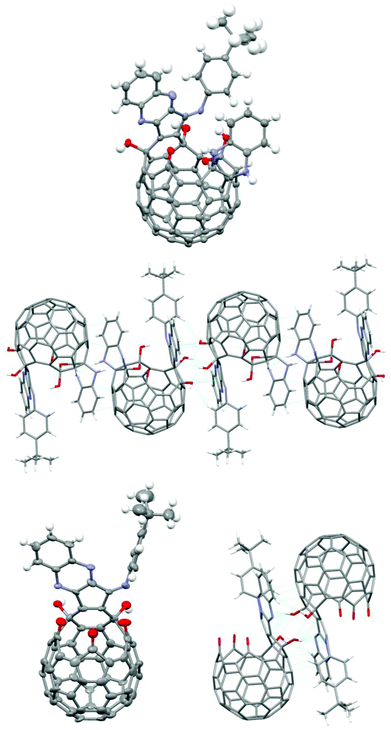 |
| Fig. 1 Single-crystal X-ray structures of 2a (top and middle) and 4a (bottom). Ellipsoids are set at 50% probability. Colour scheme grey = C, blue = N, red = O, white = H; light-blue lines indicate H-bonds and close contacts in the polymeric and dimeric structures. | |
In light of the X-ray structures of 2a and 4a, structures of other compounds were assigned according to their spectroscopic data. The ESI-MS spectra showed the molecular ion signals. In addition, the ESI-MS spectra of 4a and 4b showed minor signals due to the dimer in agreement with the dimeric packing in the X-ray structure of 4a. The strong dimeric structure of 4 is also observed in solution by NMR spectra. The 13C NMR spectrum of 4a and 4b showed a major set of signals and a minor set of signals in about 3
:
1 intensity ratio corresponding to the monomer and the dimer respectively (see supporting information† for spectra).
Formation of the tetrahydrofuran moiety on the rim of the orifice in 2a significantly reduced the size of the orifice as compared to that of 4a. The size of the orifice can be estimated by measuring the distances among the atoms on the rim of the orifice (Fig. 2). Comparison of the longest inter-atomic distances indicates that the orifice of 2a is reduced by 20% from its precursor 4a. In addition, the shape of the orifice changed from a roughly round hole in 4a to the irregular trapezoid in 2a. The space-filling model of 2a indicates that the orifice is too small to allow the passage of a water molecule. Compounds 2 to 4 thus correspond to the closed and open states for the open-cage fullerene system. The reversible conversion between 2 and 4 indicates that o-diaminobenzene could serve as a removable stopper.
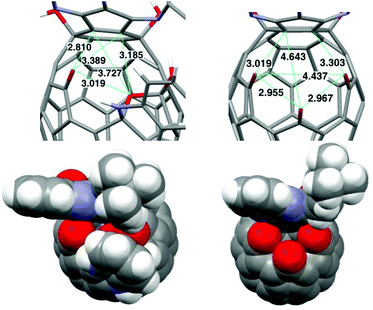 |
| Fig. 2 Orifice size parameters (Å) as measured from single-crystal X-ray structures of 2a (top left) and 4a (top right), and space-filling model of single-crystal X-ray structures of 2a (bottom left) and 4a (bottom right). Colour scheme grey = C, blue = N, red = O, white = H. | |
A number of water encapsulation and release experiments were carried out to investigate the efficiency of the o-diaminobenzene stopper for compound 4 (Scheme 3). Under normal reaction and routine purification conditions, compounds 4a and 4b contain less than 10% water encapsulated complexes as determined by the 1H NMR spectrum. Heating a chloroform or toluene solution of 4a in the presence of added water for a few hours at 80 °C could increase the water encapsulation ratio up to 80%. The trapped water in 4a could be removed by heating its solution at 80 °C in the presence of anhydrous Na2SO4. The trapped water in 4a could also exchange with D2O by heating its solution at 80 °C in the presence of D2O. The water content remained unchanged in the reaction of 4a with o-diaminobenzene at r.t. to form 2a and 3a. Under the same Na2SO4 dehydration and D2O exchange conditions at 80 °C there is hardly any change in the water content in compounds 2a and 3a. The results indicate that the tetrahydrofuran moiety in 2a and 3a acts as an effective stopper. The results are similar to compounds 2b, 3b and 4b.
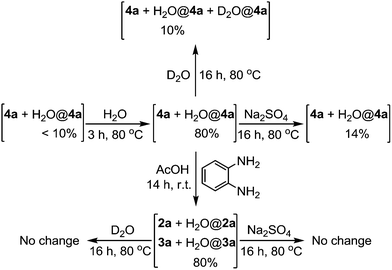 |
| Scheme 3 Water encapsulation and release experiments. Solvent of the experiments was CDCl3. Water encapsulation percentages were determined by 1H NMR integrals. | |
Experimental
Compounds 2a and 3a
Acetic acid (22 drops, 423 mg) and o-diaminobenzene (121 mg, 1.12 mmol) were added to a solution of 1a (74 mg, 0.068 mmol) in CH2Cl2 (50 mL) at r. t. After stirring for 14 h, the reaction mixture was washed with water and extracted with dichloromethane three times. The dichloromethane extraction solutions were combined and dried with anhydrous sodium sulfate, and chromatographed on a silica gel column eluting with dichloromethane/ethyl acetate (40
:
1). The solution was concentrated, and chromatographed again on a silica gel column eluting with dichloromethane. The first band was collected and evaporated to give 2a (35 mg, 0.030 mmol, 44%). The second band was eluted with dichloromethane/ethyl acetate (100
:
1) and evaporated to give 3a (26 mg, 0.030 mmol, 33%).
Characterization data for 2a.
1H NMR (400 MHz, CDCl3) δ: 8.21–8.19 (m, 1H), 7.98–8.05 (m, 1H), 7.90–7.86 (m, 1H), 7.81–7.78 (m, 1H), 7.54–7.52 (d, 2H, J = 8.6 Hz), 7.43 (d, 2H, J = 8.3 Hz), 7.36 (s, 1H), 7.14 (s, 1H), 7.02–7.00 (m, 1H), 6.88 (m, 2H), 6.72–6.67 (m, 1H), 4.36 (d, J = 5.1 Hz, 1H), 1.48 (s, 9H), −10.99 (s). Three H signals overlapped with the toluene impurity. The 13C NMR spectrum could not be obtained due to low solubility. ESI-FT-ICR-HRMS: C82H28N5O5 (M + H+) calcd 1162.2085, found 1162.2073.
Crystals of 2a suitable for X-ray diffraction were obtained by diffusion of hexane into a solution of 2a in a mixture of CH2Cl2/CHCl3 (10
:
1). Crystal data: monoclinic, space group P21/c (no. 14), a = 17.987 (3) Å, b = 18.451 (4) Å, c = 19.171 (4) Å, β = 117.87 (2)°, V = 5625 (2) Å3, Z = 4, T = 180.01 (10) K, μ(MoKα) = 0.087 mm−1, Dcalc = 1.372 g cm−3, 22
175 reflections measured (6.138° ≤ 2Θ ≤ 50.056°), 9720 unique (Rint = 0.1546, Rsigma = 0.3486) which were used in all calculations. The final R1 was 0.0870 (I > 2σ(I)) and wR2 was 0.2221 (all data).
Characterization data for 3a.
1H NMR (400 MHz, CDCl3) δ: 8.29–8.27 (d, 1H, J = 6.7 Hz), 8.23–8.21 (d, 1H, J = 8.0 Hz), 7.96–7.90 (m, 2H), 7.86–7.82 (m, 3H), 7.64–7.62 (d, 2H, J = 8.3 Hz), 7.43–7.39 (m, 1H), 6.75–6.73 (d, 1H, J = 7.4 Hz), 6.63–6.60 (m, 1H), 6.23–6.17 (m, 1H), 5.03–4.98 (m, 1H), 4.79–4.68 (m, 2H), 4.29 (s, 1H), 4.12 (s, 1H), 1.49 (s, 9H), −11.18 (s). 1H NMR (400 MHz, CDCl3/CD3OD) δ: 8.22–8.20 (d, 1H, J = 8.1 Hz), 7.98–7.91 (m, 2H), 7.87–7.81 (m, 3H), 7.66–7.64 (d, 2H, J = 8.4 Hz), 6.78–6.77 (d, 1H, J = 7.4 Hz), 6.67–6.63 (t, 1H, J = 7.4 Hz), 6.24–6.20 (t, 1H, J = 7.5 Hz), 4.98–4.96 (d, 1H, J = 7.5 Hz), 4.13 (s, 1H), 1.50 (s, 9H). 13C NMR (125 MHz, CDCl3/CD3OD): all signals represent 1C except noted, δ: 190.64, 161.64, 155.26, 151.38, 151.30, 150.98, 150.93, 150.45, 150.04, 149.67, 149.42, 149.25, 149.12, 148.98, 148.86, 148.80, 148.69, 148.47, 148.42, 148.34, 148.26, 148.18, 148.01, 147.71, 147.66, 147.18, 147.12, 147.00, 146.94, 146.47, 145.55, 145.36, 145.34, 145.30, 144.96, 144.25, 144.19, 143.64, 143.37, 143.11, 143.07, 142.93, 142.47, 142.36, 142.22, 141.41, 141.30, 140.88, 139.25, 139.19, 139.16, 138.97, 138.49, 136.10, 134.73, 134.40, 132.16, 131.30, 130.90, 130.49, 130.35, 128.98, 125.51 (3C), 123.58 (3C), 122.31, 120.21, 117.53, 114.33, 114.18, 108.10, 79.06, 77.65, 76.52, 64.78, 35.00, 31.54 (3C). ESI-FT-ICR-HRMS: C82H28N5O5 (M + H+) calcd 1162.2085, found 1162.2082.
Compound 4a
Acetic acid (1 ml) was added to a solution of 2a (25 mg, 0.022 mmol) in PhCl (15 mL) at 80 °C. After 18 h, the reaction mixture was washed with water and extracted with dichloromethane three times. The dichloromethane extraction solutions were combined and dried with anhydrous sodium sulfate. The solution was concentrated, and chromatographed on a silica gel column eluting with dichloromethane. The first red band was collected and evaporated to give 4a (20 mg, 0.019 mmol, 88%). The reaction starting from 3a was carried out under the same conditions also with 88% yield.
Characterization data for 4a.
1H NMR (400 MHz, C6D4Cl2/CD3OD) δ: 8.37–8.35 (d, 1H, J = 8.1 Hz), 8.23–8.21 (d, J = 8.4 Hz, 2H), 8.05–8.03 (d, J = 8.2 Hz, 1H), 7.94–7.90 (t, J = 7.3 Hz, 1H), 7.84 (s, 1H), 7.82 (s, 1H), 7.81–7.76 (t, J = 7.6 Hz, 1H), 1.71 (s, 9H), −12.86 (s). 13C NMR (125 MHz, C6D4Cl2/CD3OD): all signals represent 1C except noted, δ: 184.49, 184.16, 184.00, 160.74, 155.91, 151.49, 149.69, 149.44, 149.38, 149.35, 149.29, 149.25, 147.98, 147.90, 147.86, 147.82, 147.78, 147.08, 146.96, 146.50, 146.43, 145.74, 145.72, 145.62, 145.55, 145.21, 145.17, 145.10, 144.92, 144.67, 144.58, 144.36, 144.33, 144.25, 143.61, 143.42, 143.32, 143.27, 142.64, 142.53, 141.42, 141.00, 140.58, 140.44, 140.40, 136.80, 136.63, 135.86, 135.43, 134.88, 134.19, 125.28, 124.64, 78.25, 77.48, 34.86, 31.47. ESI-FT-ICR-HRMS: C76H20N3O5 (M + H+) calcd 1054.1398, found 1054.1378. C152H39N6O10 (2M + H+) calcd 2107.2722, found 2107.2749.
Crystals of 4a suitable for X-ray diffraction were obtained by diffusion of hexane into a solution of 4a in dichloromethane. Crystal data: monoclinic, space group P21/n (no. 14), a = 13.0600 (8) Å, b = 13.2703 (7) Å, c = 29.7423 (18) Å, β = 95.060 (6)°, V = 5134.5 (5) Å3, Z = 4, T = 180.00 (10) K, μ(MoKα) = 0.086 mm−1, Dcalc = 1.363 g cm−3, 13
765 reflections measured (6.292° ≤ 2Θ ≤ 47.632°), 6501 unique (Rint = 0.1193, Rsigma = 0.1636) which were used in all calculations. The final R1 was 0.0965 (I > 2σ(I)) and wR2 was 0.2328 (all data). Crystallographic data have been deposited in the Cambridge. CCDC 1414193 (4a) and 1414194 (2a) contain the supplementary crystallographic data for this paper.
Conclusions
o-Diaminobenzene is shown to react with two of the three carbonyl groups on the rim of the orifice of an open-cage fullerene to form a tetrahydrofuran moiety above the orifice, thus blocking the entry and exit of water as confirmed by D2O exchange and water releasing experiments. The added o-diaminobenzene can be readily removed by treatment with acetic acid. The reversible addition and removal of o-diaminobenzene corresponds to the controlled closing and opening of the fullerene based molecular container for a single molecule of water.
Acknowledgements
This work was supported by NSFC (21272013 and 21132007) and the Major State Basic Research Development Program (2011CB808401 and 2015CB856600).
Notes and references
- For example:
(a) K. Koga, G. T. Gao, H. Tanaka and X. C. Zeng, Nature, 2001, 412, 802 CrossRef CAS PubMed;
(b) J. Garric, J. M. Léger and I. Huc, Chem. – Eur. J., 2007, 13, 8454 CrossRef CAS PubMed;
(c) Q. Q. Wang, V. W. Day and K. Bowman-James, Angew. Chem., Int. Ed., 2012, 51, 2119 CrossRef CAS PubMed;
(d) Q. Q. Wang, V. W. Day and K. Bowman-James, J. Am. Chem. Soc., 2013, 135, 392 CrossRef CAS PubMed.
- K. Kurotobi and Y. Murata, Science, 2011, 333, 613 CrossRef CAS PubMed.
- For reviews:
(a) M. Murata, Y. Murata and K. Komatsu, Chem. Commun., 2008, 6083 RSC;
(b) G. C. Vougioukalakis, M. M. Roubelakis and M. Orfanopoulos, Chem. Soc. Rev., 2010, 39, 817 RSC;
(c) L. B. Gan, D. Z. Yang, Q. Y. Zhang and H. Huang, Adv. Mater., 2010, 22, 1498 CrossRef CAS PubMed.
- For recent examples,
(a) S. M. Liu and L. B. Gan, Chin. J. Chem., 2014, 32, 819 CrossRef CAS PubMed;
(b) R. Zhang, T. Futagoishi, M. Murata, A. Wakamiya and Y. Murata, J. Am. Chem. Soc., 2014, 136, 8193 CrossRef CAS PubMed;
(c) Y. Hashikawa, M. Murata, A. Wakamiya and Y. Murata, Org. Lett., 2014, 16, 2970 CrossRef CAS PubMed;
(d) C.-S. Chen, Y.-F. Lin and W.-Y. Yeh, Chem. – Eur. J., 2014, 20, 936 CrossRef CAS PubMed;
(e) Y. M. Yu, T. Zhang and L. B. Gan, Fullerenes, Nanotubes, Carbon Nanostruct., 2014, 22, 54 CrossRef CAS PubMed;
(f) A. Krachmalnicoff, M. H. Levitt and R. J. Whitby, Chem. Commun., 2014, 50, 13037 RSC;
(g) A. Krachmalnicoff, R. Bounds, S. Mamone, M. H. Levitt, M. Carravetta and R. J. Whitby, Chem. Commun., 2015, 51, 4993 RSC;
(h) L. Xu, H. J. Ren, S. S. Liang, J. H. Sun, Y. J. Liu and L. B. Gan, Chem. – Eur. J., 2015, 21, 13539 CrossRef CAS PubMed.
- For review, L. J. Shi and L. B. Gan, J. Phys. Org. Chem., 2013, 26, 766 CrossRef CAS PubMed.
- Q. Y. Zhang, T. Pankewitz, S. M. Liu, W. Klopper and L. B. Gan, Angew. Chem., Int. Ed., 2010, 49, 9935 CrossRef CAS PubMed.
- Q. Y. Zhang, Z. S. Jia, S. M. Liu, G. Zhang, Z. Xiao, D. Z. Yang, L. B. Gan, Z. M. Wang and Y. L. Li, Org. Lett., 2009, 11, 2772 CrossRef CAS PubMed.
- For reactions of open-cage fullerene with o-diaminobenzene,
(a) S.-i. Iwamatsu and S. Murata, Tetrahedron Lett., 2004, 45, 6391 CrossRef CAS PubMed;
(b) Z. Xiao, G. Ye, Y. Liu, S. Chen, Q. Peng, Q. Q. Zuo and L. M. Ding, Angew. Chem., Int. Ed., 2012, 51, 9038 CrossRef CAS PubMed;
(c) Y. M. Yu, L. Xu, X. C. Huang and L. B. Gan, J. Org. Chem., 2014, 79, 2156 CrossRef CAS PubMed.
- L. Xu, Q. Y. Zhang, G. Zhang, S. S. Liang, Y. M. Yu and L. B. Gan, Eur. J. Org. Chem., 2013, 7272 CrossRef CAS PubMed.
- S. M. Liu, Q. Y. Zhang, Y. M. Yu and L. B. Gan, Org. Lett., 2012, 14, 4002 CrossRef CAS PubMed.
Footnote |
† Electronic supplementary information (ESI) available: Spectroscopic data for compound 1. CCDC 1414193 (4a) and 1414194 (2a). For ESI and crystallographic data in CIF or other electronic format see DOI: 10.1039/c5qo00265f |
|
This journal is © the Partner Organisations 2015 |