DOI:
10.1039/C5QO00285K
(Research Article)
Org. Chem. Front., 2015,
2, 1632-1636
Copper-catalyzed oxidative coupling reaction of α,β-unsaturated aldehydes with amidines: synthesis of 1,2,4-trisubstituted-1H-imidazole-5-carbaldehydes†
Received
15th September 2015
, Accepted 3rd November 2015
First published on 4th November 2015
Abstract
A practical and highly functional group-compatible synthesis of 1,2,4-trisubstituted-1H-imidazole-5-carbaldehydes has been developed via copper-catalyzed oxidative coupling of amidines and α,β-unsaturated aldehydes, which features aldehyde preservation, cheap catalysts, as well as high atom economy and mild conditions.
Introduction
The imidazole core is an important scaffold that can be found in a large number of natural products (Fig. 1a),1 pharmaceuticals (Fig. 1b and c),2 and advanced materials.3 Thus, the development of synthetic protocols for imidazole derivatives has always been an active area of research.4 The Bredereck synthesis,5 Van Leusen reaction,6 Debus-Radziszewski reaction,7 and the reaction of R-haloketones with amidines8 are well documented as traditional methods to build imidazole rings. However, these procedures generally involve a strong base or relatively high temperature.
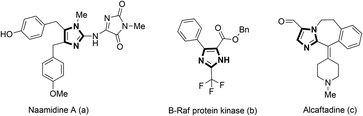 |
| Fig. 1 Examples of compounds with the imidazole skeleton. | |
With the rapid development of transition metal-catalysis in the past few decades, using simple N-arylated substrates as precursors for the synthesis of various imidazole derivatives has stimulated great research efforts.9 Among these, Cu-catalyzed oxidative synthesis of imidazole derivatives has become increasingly popular for their high efficiency.10 Typically, the group of Chiba has developed a series of strategies to afford various azaheterocycles under Cu-catalyzed oxidative conditions in the past few years.10b–g
Aldehyde is one of the most important groups in functional group transformation, such as the Aldol and Mannich reactions. As a potentially versatile synthetic intermediate, the aldehyde-substituted imidazoles contain an important reactive center for facile derivatization.11 For example, Qiao's group synthesized novel functional materials, Schiff-base linked polymeric imidazoles (SLPI),11i which are developed by aldehyde-substituted imidazoles. Based on the previous studies by others and our group,9j,t,10j,12 we report a copper-catalyzed oxidative coupling of α,β-unsaturated aldehydes with amidines to construct 1,2,4-trisubstituted-1H-imidazole-5-carbaldehydes under mild conditions with H2O as the sole byproduct.
Results and discussion
We initiated the investigation by using N-phenylbenzamidine (1a) and cinnamaldehyde (2a) as model substrates for optimization of this process, and the effects of all reaction parameters were systematically examined (Table 1). When the reaction was carried out in the presence of CuI (10 mol%), DABCO (1,4-diazabicyclo-[2.2.2]octane) (20 mol%) and PhCl (chlorobenzene) (2 mL) at 100 °C for 36 h under an air atmosphere, the desired product 3aa was isolated in 26% yield (entry 1). It was found that two equivalents of MnO2 under a N2 atmosphere increased the reactivity, producing the corresponding product 3aa in 75% yield (entry 3). Other oxidants were inefficient in the presence of N2 (entries 4–6). Among various copper catalysts that we screened, CuI gave the highest yield (entries 7–9). The replacement of DABCO with other ligands did not promote the efficiency of the reaction (entries 10–12). An evaluation of solvents revealed that PhCl was the optimal choice, while other solvents such as DCE (1,2-dichloroethane), DMF (N,N-dimethylformamide) and 1,4-dioxane showed inferior results (entries 13–15). In addition, lower yields of 3aa were obtained when we attempted to change the ratio of 1a/2a, catalyst loading and temperature (entries 16–19). When varying the amounts of MnO2, we found that the reaction with two equivalents of MnO2 offered the best yield (entry 4 vs. entries 20 and 21). The structure of 3aa was confirmed by X-ray crystallography (Fig. 2).13
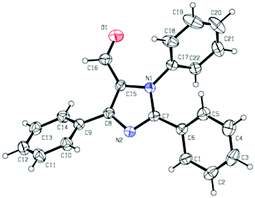 |
| Fig. 2 X-ray structure of 3aa. | |
Table 1 Optimization of reaction conditionsa
With the optimized conditions in hand, we proceeded to examine the substrate scope (Scheme 1). First, we studied the R1-substituted arylamidines. Electron-rich-substituted arylamidines such as p-Me and p-OMe gave the reaction products in excellent yields (3ba, 80%; 3ca, 82%). When both aromatic rings were substituted with electron-rich groups, the product was isolated in optimal yield (3na, 88%). Electron-deficient arylamidines bearing halide (F–, Cl–, Br–) and trifluoromethyl groups reacted under the standard conditions to afford the desired products in moderate yields (3da–3ha, 38%–62%).
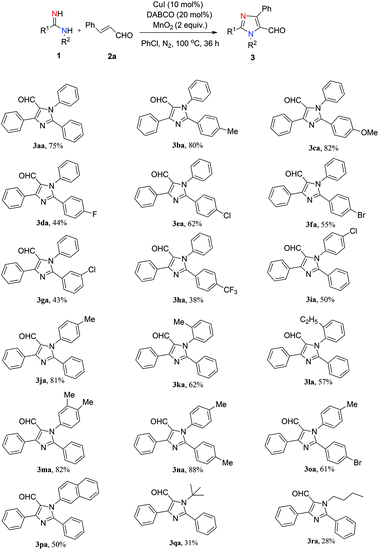 |
| Scheme 1 The scope of amidines. Isolated yields are given. Reaction conditions: 1 (0.2 mmol), 2a (0.4 mmol), CuI (10 mol%), DABCO (20 mol%), MnO2 (2 equiv.), N2, PhCl (2 mL), 100 °C, 36 h. | |
We next examined the substrate scope of this reaction using R2-substituted arylamidines. The p-Me-substituted arylamidine delivered 3ja in good yield (81%), while the p-Cl-substituted arylamidine afforded 3ia in lower yield (50%). O-Substituted arylamidines showed slightly lower reactivity than p-substituted arylamidine (3ja, 81%; 3ka, 62%), indicating that steric factors had a negative influence on this conversion. Furthermore, N-(naphthalen-2-yl)benzimidamide, N-(tert-butyl)benzimidamide and N-butylbenzimidamide were tolerated, affording the corresponding products in low yields (3pa, 50%; 3qa, 31%; 3ra, 28%).
The scope of α,β-unsaturated aldehydes was also examined (Scheme 2). Substrates bearing electron-donating groups (methyl and methoxyl) at the aromatic ring produced the corresponding products in good yields (3ab–3ad, 75–87%). The presence of electron-withdrawing substituents (F–, Cl–, Br–) at the para position reduce the efficiency of the reaction, as the corresponding products could be isolated in slightly lower yields (3ae–3ag, 57–65%). Additionally, substrates bearing a furan and an n-propyl were also compatible, albeit providing lower yields (3ai, 36%; 3aj, 34%). Unfortunately, the reaction with p-nitro-substituted α,β-unsaturated aldehyde did not afford the desired product 3ah under the standard reaction conditions. Furthermore, we also tried chalcone with 1a under the standard reaction conditions, which afforded the corresponding product (3ak) in 70% yield.
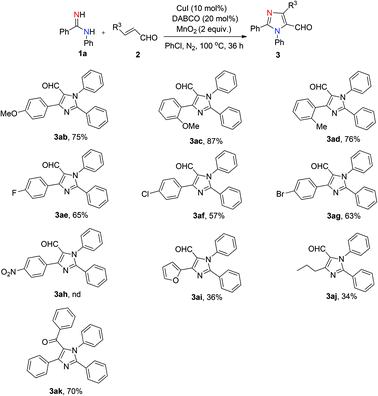 |
| Scheme 2 The scope of α,β-unsaturated aldehydes. Isolated yields are given. Reaction conditions: 1a (0.2 mmol), 2 (0.4 mmol), CuI (10 mol%), DABCO (20 mol%), MnO2 (2 equiv.), N2, PhCl (2 mL), 100 °C, 36 h. | |
To understand the possible mechanism of this reaction, several control experiments were investigated (Scheme 3). Firstly, the reaction between 1a and 2a without using a catalyst or an oxidant was carried out, but no desired product was detected (Scheme 3, eqn (1) and (2)). Moreover, when 2 equiv. of 2,2,6,6-tetramethyl-1-piperidinyloxy (TEMPO) was added into the standard reaction, the isolated yield of 3aa reduced from 75% to 51% (Scheme 3, eqn (3A)), in which it may be the weak oxidizing effect of TEMPO affected the reaction. We also tried other radical traps such as BHT (2,6-di-tert-butyl-4-methylphenol) (Scheme 3, eqn (3B)) and PBN (N-benzylidene-tert-butylamine N-oxide) (Scheme 3, eqn (3C)), which produced 3aa in 60% and 63% yields respectively, indicating that this transformation might not proceed via a radical pathway.
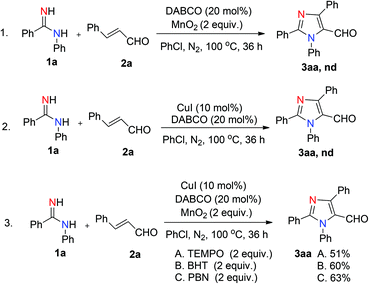 |
| Scheme 3 Controlled experiments. | |
Based on previous studies and control experiments, a plausible reaction mechanism is proposed as shown in Scheme 4.14 Initially, a Michael addition of N-arylbenzamidines (1a) to the cinnamaldehyde (2a) took place to form the corresponding Michael adduct A.14a–d Subsequently, the nitrogen atom that connected to the benzene ring bound with Cu(II) salts to produce intermediate B which simultaneously reacted with the enol to form the cyclic Cu(II) intermediate C.14e Then intermediate C on oxidation by the oxidant formed intermediate D in which copper was in the +III oxidation state.14f Finally, intermediate D through reductive elimination afforded intermediate E, which on rapid oxidative aromatization under oxidizing conditions leads to the tandem product 3aa. Reoxidation of the CuI to the CuII by MnO2 completed the catalytic cycle.
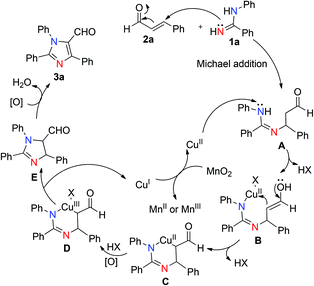 |
| Scheme 4 Proposed mechanism. | |
Conclusions
In summary, an efficient copper-catalyzed oxidative coupling of α,β-unsaturated aldehydes with amidines for the synthesis of 1,2,4-trisubstituted-1H-imidazole-5-carbaldehydes was developed, which shows a high atom economy, cheap catalysts and mild conditions. Further studies on the application of this transformation are underway.
Experimental
Typical procedure for the preparation of 3
1 (0.20 mmol), 2 (0.40 mmol), CuI (3.8 mg, 10 mol%), DABCO (4.5 mg, 20 mol%), MnO2 (34.8 mg, 2.0 equiv.), and PhCl (2 mL) were added to a flask with a magnetic stirring bar under a N2 atmosphere. The mixture was stirred at 100 °C for 36 h. After cooling to room temperature, the mixture was diluted with ethyl acetate and filtered. The filtrate was removed under reduced pressure to obtain the crude product, which was further purified by silica gel chromatography to give product 3. The identity and purity of the products was confirmed by 1H NMR and 13C NMR spectroscopic analysis.
Acknowledgements
We are grateful for sponsorship of this project by the National Natural Science Foundation of China (no. 21372102 and 21403256).
Notes and references
-
(a) Z. Jin, Nat. Prod. Rep., 2006, 23, 464–496 RSC;
(b) Z. Jin, Nat. Prod. Rep., 2009, 26, 382–445 RSC;
(c) B. Forte, B. Malgesini, C. Piutti, F. Quartieri, A. Scolaro and G. Papeo, Mar. Drugs, 2009, 7, 705–753 CrossRef CAS PubMed.
-
(a) D. J. Carini, J. V. Duncia, A. L. Johnson, A. T. Chiu, W. A. Price, P. C. Wong and P. B. M. W. Timmermans, J. Med. Chem., 1990, 33, 1330–1336 CrossRef CAS PubMed;
(b) G. J. Atwell, J.-Y. Fan, K. Tan and W. A. Denny, J. Med. Chem., 1998, 41, 4744–4754 CrossRef CAS PubMed;
(c) H. Koga, Y. Nanjoh, K. Makimura and R. Tsuboi, Med. Mycol., 2009, 47, 640–647 CrossRef CAS PubMed;
(d) J. Dietrich, V. Gokhale, X. Wang, L. H. Hurley and G. A. Flynn, Bioorg. Med. Chem., 2010, 18, 292–304 CrossRef CAS PubMed;
(e) S. J. Ono and K. Lane, Drug Des., Dev. Ther., 2011, 5, 77–84 CAS.
-
(a) R. H. Holm, P. Kennepohl and E. I. Solomon, Chem. Rev., 1996, 96, 2239–2314 CrossRef CAS PubMed;
(b) W. A. Herrmann, Angew. Chem., Int. Ed., 2002, 41, 1290–1309 CrossRef CAS;
(c) T. Yamamoto, T. Uemura, A. Tanimoto and S. Sasaki, Macromolecules, 2003, 36, 1047–1053 CrossRef CAS.
-
(a) H. Du, Y. He, R. Sivappa and C. J. Lovely, Synlett, 2006, 0965–0992 CAS;
(b) C. Kanazawa, S. Kamijo and Y. Yamamoto, J. Am. Chem. Soc., 2006, 128, 10662–10663 CrossRef CAS PubMed;
(c) R. A. Altman and S. L. Buchwald, Org. Lett., 2006, 8, 2779–2782 CrossRef CAS PubMed;
(d) F. Bellina, S. Cauteruccio and R. Rossi, Tetrahedron, 2007, 63, 4571–4624 CrossRef CAS;
(e) S. Kamijo and Y. Yamamoto, Chem. – Asian J., 2007, 2, 568–578 CrossRef CAS PubMed;
(f) F. Bellina and R. Rossi, Adv. Synth. Catal., 2010, 352, 1223–1276 CrossRef CAS;
(g) S. Ueda and S. L. Buchwald, Angew. Chem., Int. Ed., 2012, 51, 10364–10367 CrossRef CAS PubMed;
(h) J. Huang, Y. He, Y. Wang and Q. Zhu, Chem. – Eur. J., 2012, 18, 13964–13967 CrossRef CAS PubMed;
(i) S. K. Alla, R. K. Kumar, P. Sadhu and T. Punniyamurthy, Org. Lett., 2013, 15, 1334–1337 CrossRef CAS PubMed;
(j) Y. He, J. Huang, D. Liang, L. Liu and Q. Zhu, Chem. Commun., 2013, 49, 7352–7354 RSC;
(k) D. Liang, Y. He, L. Liu and Q. Zhu, Org. Lett., 2013, 15, 3476–3479 CrossRef CAS PubMed;
(l) J.-P. Lin, F.-H. Zhang and Y.-Q. Long, Org. Lett., 2014, 16, 2822–2825 CrossRef CAS PubMed;
(m) H. Chen and S. Chiba, Org. Biomol. Chem., 2014, 12, 42–46 RSC;
(n) H. Chen, A. Kaga and S. Chiba, Org. Lett., 2014, 16, 6136–6139 CrossRef CAS PubMed.
- H. Bredereck and G. Theilig, Chem. Ber., 1953, 86, 88–96 CrossRef CAS.
- A. M. Van Leusen, J. Wildeman and O. H. Oldenziel, J. Org. Chem., 1977, 42, 1153–1159 CrossRef CAS.
-
(a) B. Radziszewski, Ber. Dtsch. Chem. Ges., 1882, 15, 1493–1496 CrossRef;
(b) F. R. Japp and H. H. Robinson, Ber. Dtsch. Chem. Ges., 1882, 15, 1268–1270 CrossRef.
- F. Kunckell, Ber. Dtsch. Chem. Ges., 1901, 34, 637–642 CrossRef CAS.
-
(a) C. T. Brain and J. T. Steer, J. Org. Chem., 2003, 68, 6814–6816 CrossRef CAS PubMed;
(b) S. Zaman, K. Mitsuru and A. D. Abell, Org. Lett., 2005, 7, 609–611 CrossRef CAS PubMed;
(c) A. R. Siamaki and B. A. Arndtsen, J. Am. Chem. Soc., 2006, 128, 6050–6051 CrossRef CAS PubMed;
(d) G. Abbiati, A. Arcadi, V. Canevari and E. Rossi, Tetrahedron Lett., 2007, 48, 8491–8495 CrossRef CAS;
(e) N. Zheng, K. W. Anderson, X. Huang, H. N. Nguyen and S. L. Buchwald, Angew. Chem., Int. Ed., 2007, 46, 7509–7512 CrossRef CAS PubMed;
(f) Q. Xiao, W.-H. Wang, G. Liu, F.-K. Meng, J.-H. Chen, Z. Yang and Z.-J. Shi, Chem. – Eur. J., 2009, 15, 7292–7296 CrossRef CAS PubMed;
(g) R. K. Kumar, M. A. Ali and T. Punniyamurthy, Org. Lett., 2011, 13, 2102–2105 CrossRef CAS PubMed;
(h) D. Zhao, J. Hu, N. Wu, X. Huang, X. Qin, J. Lan and J. You, Org. Lett., 2011, 13, 6516–6519 CrossRef CAS PubMed;
(i) S. Ueda, M. Su and S. L. Buchwald, J. Am. Chem. Soc., 2012, 134, 700–706 CrossRef CAS PubMed;
(j) X. Liu, D. Wang, Y. Chen, D. Tang and B. Chen, Adv. Synth. Catal., 2013, 355, 2798–2802 CrossRef CAS;
(k) N. Zheng and S. L. Buchwald, Org. Lett., 2007, 9, 4749–4751 CrossRef CAS PubMed;
(l) D. Yang, H. Fu, L. Hu, Y. Jiang and Y. Zhao, J. Org. Chem., 2008, 73, 7841–7844 CrossRef CAS PubMed;
(m) X. Deng, H. McAllister and N. S. Mani, J. Org. Chem., 2009, 74, 5742–5745 CrossRef CAS PubMed;
(n) X. Deng and N. S. Mani, Eur. J. Org. Chem., 2010, 680–686 CrossRef CAS;
(o) H. Wang, Y. Wang, C. Peng, J. Zhang and Q. Zhu, J. Am. Chem. Soc., 2010, 132, 13217–13219 CrossRef CAS PubMed;
(p) J. Peng, M. Ye, C. Zong, F. Hu, L. Feng, X. Wang, Y. Wang and C. Chen, J. Org. Chem., 2011, 76, 716–719 CrossRef CAS PubMed;
(q) J. Li, S. Bénard, L. Neuville and J. Zhu, Org. Lett., 2012, 14, 5980–5983 CrossRef CAS PubMed;
(r) H.-F. He, Z.-J. Wang and W. Bao, Adv. Synth. Catal., 2010, 352, 2905–2912 CrossRef CAS;
(s) K.-S. Masters, T. R. M. Rauws, A. K. Yadav, W. A. Herrebout, B. Van der Veken and B. U. W. Maes, Chem. – Eur. J., 2011, 17, 6315–6320 CrossRef CAS PubMed;
(t) D. Tang, X.-L. Li, X. Guo, P. Wu, J.-H. Li, K. Wang, H.-W. Jing and B.-H. Chen, Tetrahedron, 2014, 70, 4038–4042 CrossRef CAS.
-
(a) G. Brasche and S. L. Buchwald, Angew. Chem., Int. Ed., 2008, 47, 1932–1934 CrossRef CAS PubMed;
(b) H. Chen, S. Sanjaya, Y.-F. Wang and S. Chiba, Org. Lett., 2013, 15, 212–215 CrossRef CAS PubMed;
(c) S. Sanjaya and S. Chiba, Org. Lett., 2012, 14, 5342–5345 CrossRef CAS PubMed;
(d) S. Sanjaya, S. H. Chua and S. Chiba, Synlett, 2012, 1657–1661 CAS;
(e) K. K. Toh, S. Sanjaya, S. Sahnoun, S. Y. Chong and S. Chiba, Org. Lett., 2012, 14, 2290–2292 CrossRef CAS PubMed;
(f) Y.-F. Wang, X. Zhu and S. Chiba, J. Am. Chem. Soc., 2012, 134, 3679–3682 CrossRef CAS PubMed;
(g) Y.-F. Wang, H. Chen, X. Zhu and S. Chiba, J. Am. Chem. Soc., 2012, 134, 11980–11983 CrossRef CAS PubMed;
(h) H. Wang, Y. Wang, D. Liang, L. Liu, J. Zhang and Q. Zhu, Angew. Chem., Int. Ed., 2011, 50, 5678–5681 CrossRef CAS PubMed;
(i) J. Li and L. Neuville, Org. Lett., 2013, 15, 1752–1755 CrossRef CAS PubMed;
(j) D. Tang, P. Wu, X. Liu, Y.-X. Chen, S.-B. Guo, W.-L. Chen, J.-G. Li and B.-H. Chen, J. Org. Chem., 2013, 78, 2746–2750 CrossRef CAS PubMed.
-
(a) L. Oresmaa, H. Kotikoski, M. Haukka, J. Salminen, O. Oksala, E. Pohjala, E. Moilanen, H. Vapaatalo, P. Vainiotalo and P. Aulaskari, J. Med. Chem., 2005, 48, 4231–4236 CrossRef CAS PubMed;
(b) Y. Sunatsuki, R. Kawamoto, K. Fujita, H. Maruyama, T. Suzuki, H. Ishida, M. Kojima, S. Iijima and N. Matsumoto, Inorg. Chem., 2009, 48, 8784–8795 CrossRef CAS PubMed;
(c) B. Shi, Z. Shen, H. Zhang, J. Bi and S. Dai, Biomacromolecules, 2011, 13, 146–153 CrossRef PubMed;
(d) Y. Wu, X.-P. Zhou, J.-R. Yang and D. Li, Chem. Commun., 2013, 49, 3413–3415 RSC;
(e) X.-P. Zhou, Y. Wu and D. Li, J. Am. Chem. Soc., 2013, 135, 16062–16065 CrossRef CAS PubMed;
(f) F. Reichel, J. K. Clegg, K. Gloe, K. Gloe, J. J. Weigand, J. K. Reynolds, C.-G. Li, J. R. Aldrich-Wright, C. J. Kepert and L. F. Lindoy, Inorg. Chem., 2014, 53, 688–690 CrossRef CAS PubMed;
(g) V. K. Outlaw, F. B. d'Andrea and C. A. Townsend, Org. Lett., 2015, 17, 1822–1825 CrossRef CAS PubMed;
(h) P. B. Pansuriya, G. E. Maguire and H. B. Friedrich, Spectrochim. Acta, Part A., 2015, 142, 311–319 CrossRef CAS PubMed;
(i) B. Shi, H. Zhang, S. Dai, X. Du, J. Bi and S. Z. Qiao, Small, 2014, 10, 871–877 CrossRef CAS PubMed.
-
(a) J. Qu, P. Wu, D. Tang, X. Meng, Y. Chen, S. Guo and B. Chen, New J. Chem., 2015, 39, 4235–4239 RSC;
(b) S. B. Ferreira, M. S. Costa, N. Boechat, R. J. S. Bezerra, M. S. Genestra, M. M. Canto-Cavalheiro, W. B. Kover and V. F. Ferreira, Eur. J. Med. Chem., 2007, 42, 1388–1395 CrossRef CAS PubMed;
(c) B. Hu, Z. Wang, N. Ai, J. Zheng, X.-H. Liu, S. Shan and Z. Wang, Org. Lett., 2011, 13, 6362–6365 CrossRef CAS PubMed.
- CCDC 1409382 contains the supplementary crystallographic data for this paper.
-
(a) J. B. Bharate, S. Abbat, P. V. Bharatam, R. A. Vishwakarma and S. B. Bharate, Org. Biomol. Chem., 2015, 13, 7790–7794 RSC;
(b) K. Monir, A. Kumar Bagdi, S. Mishra, A. Majee and A. Hajra, Adv. Synth. Catal., 2014, 356, 1105–1112 CrossRef CAS;
(c) P. Kaswan, K. Pericherla, Rajnikant and A. Kumar, Tetrahedron, 2014, 70, 8539–8544 CrossRef CAS;
(d) Y. Zhu, C. Li, J. Zhang, M. She, W. Sun, K. Wan, Y. Wang, B. Yin, P. Liu and J. Li, Org. Lett., 2015, 17, 3872–3875 CrossRef CAS PubMed;
(e) S. Ueda and H. Nagasawa, J. Am. Chem. Soc., 2009, 131, 15080–15081 CrossRef CAS PubMed;
(f) A. E. King, L. M. Huffman, A. Casitas, M. Costas, X. Ribas and S. S. Stahl, J. Am. Chem. Soc., 2010, 132, 12068–12073 CrossRef CAS PubMed.
Footnote |
† Electronic supplementary information (ESI) available. CCDC 1409382. For ESI and crystallographic data in CIF or other electronic format see DOI: 10.1039/c5qo00285k |
|
This journal is © the Partner Organisations 2015 |
Click here to see how this site uses Cookies. View our privacy policy here.