DOI:
10.1039/C4RA12262C
(Paper)
RSC Adv., 2015,
5, 20764-20768
Biologically based method for the synthesis of Hg–Se nanostructures by Shewanella spp.†
Received
16th October 2014
, Accepted 5th February 2015
First published on 6th February 2015
Abstract
Living organisms, especially microorganisms, have the potential to offer cheap and benign synthetic routes for the production of nanomaterials. In this study, we demonstrated the formation of various Hg–Se nanostructures by a combinatory method with the major participation of Shewanella bacteria. The quantum dots of Hg–Se were produced and located on the membrane surface of Shewanella cells by incubating the Shewanella putrefaciens 200 with Hg(II) and Se(IV) under anaerobic conditions. The selenium nanowires and stellated polyhedral structures, successfully synthesized by Shewanella-mediated formation in 80 and 90% of DMSO, were used as templates for the formation of Hg–Se nanowires and stellated polyhedral structures. The liquid mercury was directly reacted with Se nanowires or stellated polyhedral structures in water and coated the Se nanostructures to form the core–shell structures of Hg–Se after 12 hours incubation at ambient temperature. SEM, TEM, XRD and EDX were used to characterize and confirm the Hg–Se nanoparticles and nanowires or stellated polyhedral structures.
Introduction
Recently, research into the synthesis of metal chalcogenides nanoparticles has been paid great attention due to the promising properties for catalysts, infrared detectors, light emitting diodes and electrochemical cells.1–4 In particular, nanocrystalline HgSe particles have been synthesized by the reducing reaction of mercury salt and SeCl4 in the presence of triethanolamine (TEA) as a complexing agent and hydrazine hydrate as a reductant under ultrasonication.5 The chemical route for the formation of HgSe nanoparticles was also applied for the study of the conductivity behaviour of polyvinyl alcohol–HgSe quantum dot hybrid films.4 In addition, HgSe particles possess the unique combination of properties characterized by the high electron mobility, large electron concentration and a variation in band gap with temperature,6 however, the toxicity of the chemicals in the preparation is concerning.
The biofabrication of nanomaterials provided economical and non-hazardous means because the living organisms (microbes/plants) are able to reduce metals or to facilitate the synthesis of nanoparticles under benign conditions. Bacteria are well known for the biofactory of functional metal nanoparticles such as nanocatalysts, nanomagnets, antimicrobials, remediation agents and quantum dots for electronic and optical devices.7 Among them, Shewanella spp. offered great applications in the production of nanomaterials from nanocatalyst (Pd,8 Au9) to nanomagnets of iron,10 silver nanoparticles for antimicrobial activity,11 and the As–S nanotubes for optoelectronic devices12 due to its capability of reducing various metals.
Elemental selenium has been known for its extremely high affinity to mercury with ΔGof = −38.1 kJ mol−1, capturing elemental mercury in the stable form of Hg–Se, as compared to sulfur, copper, nickel, zinc, and silver materials used.13 Take that advances, we proposed a combinatory approach including biological and chemical routs for the formation of different nanostructures of Hg–Se, in which Shewanella spp. acted as reducing and stablizing agents to produce chemical precursors for the formation of Hg–Se nanoparticles and mediating agents to form templates for the synthesis of Hg–Se nanowires or stellated polyhedral nanostructures.
Materials and methods
Bacterial strains and chemicals
All Shewanella strains were kindly supported from Laboratory of Environmental Microbiology, School of Environmental Science and Engineering, Gwangju Institute of Science and Technology (Gwangju, Korea). All other chemicals and solvents were analytical grade and from standard commercial sources (Sigma-Aldrich, Louise, MO).
Formation of the Hg–Se nanoparticles by Shewanella putrefaciens 200
The nanoparticles of Hg–Se were produced by incubating Shewanella putrefaciens 200 (OD600 = 0.1) in 50 ml of the defined medium containing 0.1 mM selenite (Se(IV)), 0.1 mM mercury chloride, and 10 mM pyruvate at 30 °C under the nitrogen-fluxing condition.14 Bacterial cultures were centrifuged at 10
400 × g for 10 min, and the Se(IV) and Hg(II) concentration in the supernatant was measured by inductively coupled plasma-optical emission spectrometry (ICP OES) (Perkin Elmer, Shelton, CT) or inductively coupled plasma-mass spectrometry (ICPMS) (Perkin Elmer, Shelton, CT) at the interval of four or eight hours. The black precipitates of Hg–Se nanoparticles and bacterial cells were collected at 15
700 × g centrifugation for 5 min, and washed three times with 5 ml of deionized water. TEM, EDS, and XRD were used to characterize the washed black precipitates.
Formation of the Hg–Se nanowires and polyhedral structures
Se nanowires and polyhedral structures were formed by the suspension of the biogenic Se nanospheres14,16,17,20 and bacterial pellets (2.6 mg total weight) in one ml of aqueous solution containing 80 or 90% DMSO, and incubated at 30 °C for 24 h.15 The solution was centrifuged and washed as described above. The Se nanowires and polyhedral structures formed from the reaction were resuspended in one μl deionized H2O, and one μl drop of liquid mercury was added and incubated for 12 h. The produced black supernatant was decanted from the drop of liquid mercury and centrifuged to collect the black precipitates at 15
700 × g for 5 min. The black precipitates were washed three times as described previously and characterized with SEM, TEM, EDS and XRD.
Scanning electron microscopy (SEM), transmission electron microscopy (TEM) and X-ray powder diffraction (XRD) analyses
For scanning electron microscopy, 10 μl samples were dropped onto silicon wafers, dried at room temperature for 12 h, and analyzed by FE-SEM (S-4700, Hitachi) at 15 kV. The samples (10 μl) for transmission electron microscopy were placed onto copper grids and images were obtained with FE-TEM (JEM-2100F, Jeol) at an acceleration voltage of 200 kV. The X-ray powder diffraction analysis was performed using a D/max Ultima III diffractometer (Rigaku, Tokyo, Japan), equipped with monochromatic high-intensity Cu Kα radiation (λ = 1.54056 Å). Particle sizes were measured in SEM and TEM images by using the software ImageJ (http://rsbweb.nih.gov/ij/download.html).
Results and discussion
Formation of the Hg–Se nanoparticles by Shewanella putrefaciens 200
Fig. 1 described the TEM images of Shewanella cells with the black precipitates of Hg–Se and the EDS data. The black precipitates were produced by the incubation of Shewanella putrefaciens 200 cells in the 100 ml medium with the supplement of 0.1 mM mercury chloride and 0.1 mM sodium selenite for 7 days at the room temperature in 150 ml serum bottles. The TEM image depicted clearly mono-disperse black nano-sized particles and the size of particles on the cell surface is approximately 4.3 + 0.79 nm in average diameter based on the measurement of particles in the TEM images by ImageJ software (Fig. 1, ESI 1†). The EDS data of black precipitates confirmed the composition of particles contained Hg and Se elements. The composition of particles were indirectly confirmed by the percentage removal of aqueous Se(IV) and Hg(II) in the supernatant (Fig. 2). The kinetic of two metal removal showed that bacterial cells started to remove Hg(II) but not Se(IV) at time 4 h. The aqueous Se(IV) reduction occurred after 48 h incubation and the removal of Hg(II) increased again after 56 h incubation.
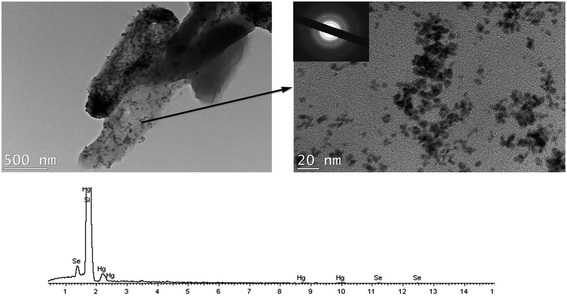 |
| Fig. 1 TEM images and EDS results of the Hg–Se particles produced by Shewanella putrefaciens 200 after 6 days. | |
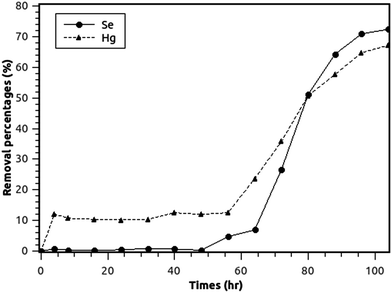 |
| Fig. 2 The kinetics of Se(IV) and Hg(II) removal from the aqueous medium. | |
Shewanella bacteria is known for the reduction of various metals including As(V), Cr(VI), Hg(II), U(VI), and Se(IV).9,12,14,18–21 Our previous study20 hypothesized that Shewanella putrefaciens 200 reduced Hg(II) ion into Hg(0) via the mercury resistance mechanism rather than the terminal electron acceptor mechanism as Wiatrowski (2006) reported and that the reduction of Se(IV) was able to induce the Hg(II) reduction. In this experiment, the removal of aqueous Hg(II) at time 4 h resulted from the adsorption of Hg(II) ion onto the bacterial cell surface with the negative charge or to glass serum bottles. The anaerobic condition and the toxicity of metals (probably both of Se(IV) and Hg(II)) in the serum bottle inhibited the growth rate of bacterial cells, thereby, bacteria used Se(IV) as final electron acceptor and induced the mercury resistance mechanism.20 Whether Hg(II) ions were reduced by the final electron acceptor or resistance mechanism, the elemental mercury is excreted out of the cells and reacts with elemental selenium formed in the surface of the bacterial cells.14,16,20 Here, the cell surface of the bacteria played an important role in the formation and stabilization of the mono-disperse, Hg–Se quantum dots. The cell membrane acts as a shelter for the electron transport system donating electron to Se(IV)16,22 and the two reduction processes with the approximately same rate ratio (Fig. 2).
Formation of the Hg–Se nanowires and polyhedral structures
The three-steps benign method has been developed to synthesize the Hg–Se nanowires or polyhedral structures, including the biological synthesis of spherical selenium nanoparticles by Shewanella putrefaciens 200, the formation of nanowires or polyhedral structures by bacterial cells' mediation, and the chemical reaction with the liquid mercury. The image of SEM showed that the nanowires or polyhedral structure of selenium was unchanged but there were short rips at the tip of polyhedral structure (Fig. 3). The characterisation of two structures with TEM image and the lining profile of elements depicted the core–shell structure of nanowires and polyhedral structure of Hg–Se (Fig. 4). The elemental composition of two structures included Hg and Se element and the atom percentage of Se in both the nanowires and polyhedral structure was higher than Hg atom percentage (Table 1). In the stellated polyhedral structure, the mass percentage of Se was 4 times higher than mass percentage of Hg, implying the Hg element only reacted with the Se atom on the surface of Se stellated polyhedral structure. Further characterization by XRD confirmed that the hexagonal crystal structure of the selenium nanowires and polyhedral structures was transformed after the reaction with Hg element, in particular, the major peaks of selenium hexagonal plane were disappeared (Fig. 5).
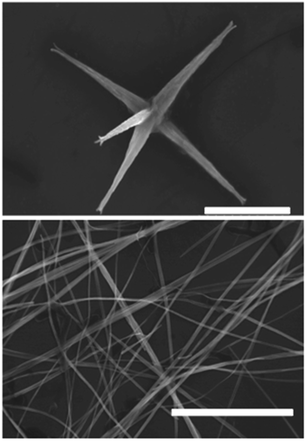 |
| Fig. 3 SEM images of the Hg–Se nanowires and polyhedral structures formed by Shewanella-mediating Se nanowires and polyhedral structures with liquid mercury. Scale bar = 5 μm. | |
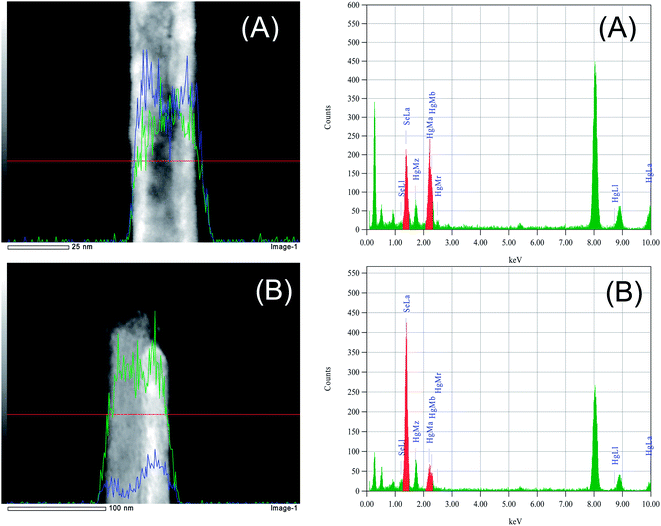 |
| Fig. 4 TEM images, lining profiles and EDS results of the Hg–Se nanowires (A) at magnification of 1 500 000 and polyhedral structures (B) at magnification 600 000. | |
Table 1 The composition of elements found in the Hg–Se nanowires or stellated polyhedral structures from EDS data
Element |
Nanowires |
Polyhedral structures |
Mass (%) |
Atom (%) |
Mass (%) |
Atom (%) |
Se |
39.6 |
62.5 |
83.2 |
92.6 |
Hg |
60.4 |
37.5 |
16.8 |
7.4 |
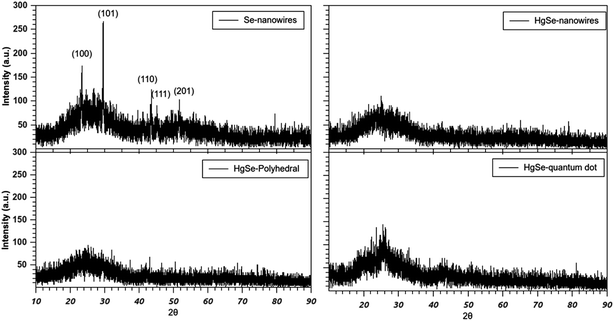 |
| Fig. 5 XRD results of Se nanowires and different Hg–Se nanostructures produced by Shewanella spp. mediation. | |
The reaction between mercury and selenium elements is able to occur at the room temperature due to the high affinity of selenium with mercury.13 Johnson et al. (2008) reported that commercial, stabilized selenium has the Hg capture capacity of over 5000 (μg g−1), meanwhile, amorphous and unstabilized selenium has the capacity of 188
000 (μg g−1). In this experiment, the nanowires of hexagonal selenium in the brown color turned to the black nanowires within 12 h incubation with mercury element, and longer reaction time resulted in the fragments of nanowires observed in TEM image (ESI 2 and 3†). In contrast, the Hg–Se nanoparticles produced by Shewanella putrefaciens 200 were formed directly by the reaction between produced Hg and Se element due to the active amorphous selenium. The crystallized Se favors stronger covalent bonds over the relatively weak interchain van der Waals forces,23 thereby, the crystallized nanowires and polyhedral structures of Se are more stable than amorphous Se. By controlling the reaction time, the crystalline selenium nanowires or stellated polyhedral structures captured the Hg element on the surface of materials and the bonding forces between Se atoms in the crystal held the reacted Se atoms in the position. In contrast, because of the weak bonding forces between amorphous Se atoms, the reacted amorphous Se atoms on the surface of the Se particles are not held in the position and released out as Jiang et al. (2012) reported previously.20
Conclusions
The biofabrication of nanomaterials are increasingly aware of as simple and economical synthesis routes. We described here the first demonstration of the biologically based method with the combination with facile chemical routes for the synthesis of different nanostructure of Hg–Se as a potential example for the combinatory and safer strategy to produce nanomaterials. Shewanella spp. facilitated the production of Hg–Se nanoparticles with the size of 4 nm and mediated the formation of Se nanowires and stellated polyhedral structures serving as templates for the synthesis of the similar structures of Hg–Se. Although the mechanism for the reduction of Hg(II) and Se(IV) and formation of Hg–Se nanowires or stellated polyhedral structure should be studied further, Shewanella spp. played an important role in the process of the production of the stable and mono-disperse Hg–Se nanoparticles as well as the formation of the Hg–Se nanowires or stellated polyhedral structures.
Acknowledgements
This research is funded by Vietnam National Foundation for Science and Technology Development (NAFOSTED) under grant number 106.16-2011.65.
References
- V. L. Colvin, M. C. Schlamp and A. P. Alivisatos, Nature, 1994, 370, 354–357 CrossRef CAS.
- M. K. Mathe, S. M. Cox, V. Venkatasamy, U. Happek and J. L. Stickney, J. Electrochem. Soc., 2005, 152, C751–C755 CrossRef PubMed.
- S. Einfeldt, M. Behringer, J. Nurnberger, H. Heinke, T. Behr, C. R. Becker, D. Hommel and G. Landwehr, Phys. Status Solidi B, 1995, 187, 439–450 CrossRef CAS.
- S. Sinha, S. K. Chatterjee, J. Ghosh and A. K. Meikap, J. Phys. D: Appl. Phys., 2014, 47, 275301 CrossRef.
- M. Esmaeili-Zare, M. Salavati-Niasari and A. Sobhani, J. Ind. Eng. Chem., 2014, 20, 3518–3523 CrossRef CAS PubMed.
- J. Ren, D. B. Eason, L. E. Churchill, Z. Yu, C. Boney, J. W. Cook Jr, J. F. Schetzina and N. A. El-Masry, J. Cryst. Growth, 1994, 138, 455–463 CrossRef CAS.
- J. R. Lloyd, J. M. Byrne and V. S. Coker, Curr. Opin. Biotechnol., 2011, 22, 509–515 CrossRef CAS PubMed.
- W. De Windt, P. Aelterman and W. Verstraete, Environ. Microbiol., 2005, 7, 314–325 CrossRef CAS PubMed.
- A. K. Suresh, D. a. Pelletier, W. Wang, M. L. Broich, J.-W. Moon, B. Gu, D. P. Allison, D. C. Joy, T. J. Phelps and M. J. Doktycz, Acta Biomater., 2011, 7, 2148–2152 CrossRef CAS PubMed.
- J. Lee and Y. Roh, J. Microbiol. Biotechnol., 2008, 18, 1572–1577 CAS.
- A. K. Suresh, M. J. Doktycz, W. Wang, J. W. Moon, B. Gu, H. M. Meyer, D. K. Hensley, D. P. Allison, T. J. Phelps and D. A. Pelletier, Acta Biomater., 2011, 7, 4253–4258 CrossRef CAS PubMed.
- J.-H. Lee, M.-G. Kim, B. Yoo, N. V Myung, J. Maeng, T. Lee, A. C. Dohnalkova, J. K. Fredrickson, M. J. Sadowsky and H.-G. Hur, Proc. Natl. Acad. Sci. U. S. A., 2007, 104, 20410–20415 CrossRef CAS PubMed.
- N. C. Johnson, S. Manchester, L. Sarin, Y. Gao, I. Kulaots and R. H. Hurt, Environ. Sci. Technol., 2008, 42, 5772–5778 CrossRef CAS.
- J.-H. Lee, J. Han, H. Choi and H.-G. Hur, Chemosphere, 2007, 68, 1898–1905 CrossRef CAS PubMed.
- C. T. Ho, J. W. Kim, W. B. Kim, K. Song, R. a. Kanaly, M. J. Sadowsky and H.-G. Hur, J. Mater. Chem., 2010, 20, 5899 RSC.
- A. Klonowska, T. Heulin and A. Vermeglio, Appl. Environ. Microbiol., 2005, 71, 5607–5609 CrossRef CAS PubMed.
- R. S. Oremland, M. J. Herbel, J. S. Blum, S. Langley, T. J. Beveridge, P. M. Ajayan, T. Sutto, A. V. Ellis and S. Curran, Appl. Environ. Microbiol., 2004, 70, 52–60 CrossRef CAS.
- S. Jiang, M.-G. Kim, S.-J. Kim, H. S. Jung, S. W. Lee, D. Y. Noh, M. J. Sadowsky and H.-G. Hur, Chem. Commun., 2011, 47, 8076–8078 RSC.
- H. a. Wiatrowski, P. M. Ward and T. Barkay, Environ. Sci. Technol., 2006, 40, 6690–6696 CrossRef CAS.
- S. Jiang, C. T. Ho, J.-H. Lee, H. Van Duong, S. Han and H.-G. Hur, Chemosphere, 2012, 87, 621–624 CrossRef CAS PubMed.
- K. Tam, C. T. Ho, J.-H. Lee, M. Lai, C. H. Chang, Y. Rheem, W. Chen, H.-G. Hur and N. V. Myung, Biosci., Biotechnol., Biochem., 2010, 74, 696–700 CrossRef CAS PubMed.
- T. E. Meyer, A. I. Tsapin, I. Vandenberghe, L. D. E. Smet, D. Frishman, K. H. Nealson, M. A. Cusanovich, J. J. V. A. N. Beeumen and M. E. T. Al, OMICS: J. Integr. Biol., 2004, 8(1), 57–77 CrossRef CAS PubMed.
- Y. Xia, P. Yang, Y. Sun, Y. Wu, B. Mayers, B. Gates, Y. Yin, F. Kim and H. Yan, Adv. Mater., 2003, 15, 353–389 CrossRef CAS.
Footnote |
† Electronic supplementary information (ESI) available: TEM image of the Se nanowires after 24 h incubation with liquid mercury. See DOI: 10.1039/c4ra12262c |
|
This journal is © The Royal Society of Chemistry 2015 |
Click here to see how this site uses Cookies. View our privacy policy here.