DOI:
10.1039/C4RA14382E
(Paper)
RSC Adv., 2015,
5, 16847-16855
Hydrophobic mesoporous silica-supported heteropolyacid induced by ionic liquid as a high efficiency catalyst for the oxidative desulfurization of fuel†
Received
12th November 2014
, Accepted 8th January 2015
First published on 9th January 2015
Abstract
Hydrogen peroxide is usually used as an oxidant in oxidative desulfurization. However, in order to reach a high level of sulfur removal, excess of hydrogen peroxide needs to be added into the oxidative system. In this study, phosphomolybdic acid immobilized on imidazole-based ionic liquid modified mesoporous SBA-15 (HPMo-IL/SBA-15) was prepared. The as-prepared material combines the advantages of H3PMo12O40 (HPMo) and SBA-15. The high special surface area of SBA-15 could well disperse the HPMo active species. The results show that HPMo could be steadily immobilized across the surface of SBA-15 by using an imidazole-based ionic liquid. At the same time, after the introduction of the imidazole-based ionic liquid, HPMo-IL/SBA-15 material exhibited hydrophobic property. Experiments demonstrated that the hydrophobic catalyst exhibited a high activity in oxidative desulfurization. When hydrogen peroxide with a stoichiometric ratio (H2O2/sulfur mole ratio is 2) was used, the removal of dibenzothiophene (DBT) could achieve levels of above 90% at 60 °C in 90 min. When the concentration of hydrogen peroxide was slightly increased (H2O2/sulfur mole ratio increased from 2 to 2.5), the sulfur removal reached 100% in 40 min. Moreover, the synthesis catalyst could adsorb dibenzothiophene sulfone (DBTO2), which is the oxidant product of DBT. In this regard, HPMo-IL/SBA-15 served as not only a high-efficiency catalyst but also an adsorbent.
1. Introduction
Recently, fossil fuels are the most widely used sources of energy in the world, providing over 80% of the worlds energy, of which half comes from petroleum.1–3 At this time, the sulfur content has a great effect on the value of crude oil. As severe environmental pollution problems are occurring, in many countries the sulfur content in diesel is limited to be less than 50 ppm or even less than 10 ppm; therefore; many efforts have been made to seek the highly efficient strategies for the deep desulfurization of fuel. It is known that most of the sulfur compounds in diesel are removed by hydrodesulfurization (HDS),4–6 but this method has a few distinct disadvantages such as severe operating conditions, less effective for the removal of aromatic organosulfur compounds (e.g. dibenzothiophene (DBT)) and their alkyl substitutions (e.g. 4,6-dimethyl-dibenzothiophen (4,6-DMDBT)). These disadvantages can be solved by oxidative desulfurization (ODS).7–10 The ODS process has the advantages of a low reaction temperature, low pressure and no hydrogen being involved. Compared with HDS, DBT and its derivates can be easily oxidized into the corresponding sulfoxides and sulfones and these oxidized products are removed by extraction or adsorption.
There have been many oxidative systems used to remove the sulfur compounds in fuels such as H2O2/organic acid,11,12 H2O2/Ti-zeolite,13,14 H2O2/IL,15–20 H2O2/heteropolyacid,21–26 and other non-hydrogen peroxide systems.27,28 Among them, the widely used oxidant is H2O2, for H2O2 as the “green” oxidant, water is the sole byproduct of H2O2 oxidation. However, it can be found that excess H2O2 is used in almost all of these oxidative desulfurization systems. This was mainly because of two reasons: some catalysts would cause H2O2 decomposing to occur drastically, leading the low utilization of H2O2; on the other hand, the surplus amount of oxidant could promote the equilibrium reaction, so the excess H2O2 could improve the desulfurization efficiency. Therefore, in view of economic reasons, exploring efficient catalysts to decrease the amount of H2O2 used for ODS is desirable.
As we know, large number of studies reported that heteropolyacid (HPA) and their derivatives, which have been widely studied as efficient catalysts for a variety of organic reactions, such as oxidation of alcohols,29,30 epoxidation of olefins,31 and oxidative desulfurization,32–35 due to their strong Brönsted acidity, redox, and structure alternative properties. However, the main disadvantages of them are extremely low surface area, instability and leaching problems.
Homogeneous catalysts normally exhibit a high level of activity in catalytic oxidative desulfurization, but their separation and regeneration is difficult. Though the heterogeneous catalysts show excellent performance in recovery and reuse compared with homogeneous catalysts, the catalytic sites have a lower exposure to the reactants. In order to achieve the heterogenization of homogeneous HPA catalysts, many research groups have made efforts to encapsulate HPA into support materials, which possess large surface areas to make the active sites of HPA more accessible to reactants.36–41 The support material is one of the most critical elements for a heterogeneous catalyst. It is known that mesoporous materials have been widely used in many fields, due to the large pore size and specific surface area. Especially, mesoporous silica material SBA-15 exhibits excellent textural properties and has been considered as a potential candidate of the support material.42–44 However, due to the hydrophilic nature of SBA-15, the activity of these catalysts can often decline for the reactions that are hydrophobic in the initial stages.45 Herein, to address this problem, our group designed a heterogeneous catalyst via the immobilization of phosphomolybdic acid on the imidazole-based ionic liquid functionalized SBA-15 and employed it for oxidative desulfurization. It is also noteworthy to mention that due to the introduction of IL, the heterogeneous catalyst exhibited good wettability for the model oil, which could provide more exposure active sites access for the reactants. The heterogeneous catalyst displayed high catalytic performance for removing sulfur compounds from the model oil; moreover, it was realized that the lowest amount of hydrogen peroxide (H2O2/S mole ratio is 2, the stoichiometric ratio) was added into the reaction system. The catalyst is stable and reusable. In addition, the as-prepared material functioned integrally both as a catalyst and as an adsorbent for the ODS process. After the reaction, the oxidant production could be adsorbed onto the catalyst, which can obtain clear oil. These advantages paved the way for research of effective, eco-friendly, and reusable heterogeneous catalysts.
2. Experimental section
2.1. Preparation of the catalyst
2.1.1. Materials. Commercially available 30 wt% H2O2, phosphomolybdic acid hydrate (H3PMo12O40), n-octane, tetradecane, ethylsilicate (TEOS) and N-methylimidazole were purchased from Sinopharm Chemical Reagent Co., Ltd. Benzothiophene (BT), dibenzothiophene (DBT), 4,6-dimethyldibenzothiophene (4,6-DMDBT) and poly(ethyleneglycol)-block-poly(propylene glycol)-block-poly(ethylene glycol) (Pluronic P123) were purchased from Sigma-Aldrich. (3-Chloropropyl)trimethoxysilane (98%) was marketed by Aladdin Chemistry.
2.1.2. Preparation of 1-methyl-3-(trimethoxysilylpropyl)-imidazolium chloride ([pmim]Cl). 8.2 g (0.1 mol) of N-methyl imidazolium and 19.87 g (0.1 mol) of 3-chloropropyltriethoxysilan were added to a three-neck glass flask and the mixture was refluxed for 24 h at 95 °C. 1-Methyl-3-(trimethoxysilylpropyl)-imidazolium chloride ([pmim]Cl) ionic liquid was obtained.
2.1.3. Grafting of ionic liquid ([pmim]Cl) on SBA-15 ([pmim]Cl-SBA-15, denoted as IL/SBA-15). Pure siliceous SBA-15 was synthesized according to the procedures reported previously,46 using P123 as the template and TEOS as the silica source. SBA-15 (1 g) was dispersed in 50 mL of dried toluene containing 0.5 g [pmim]Cl. The mixture was stirred at 90 °C reflux for 16 h and cooled to room temperature. The solid production was separated by filtration. Then, the solid production was dealt with by 48 h of extraction with boiling dichloromethane and then dried under vacuum at 50 °C for 24 h.
2.1.4. Preparation of immobilized HPMo-based ionic liquid on SBA-15 (HPMo-IL/SBA-15). The catalysts of xHPMo-IL/SBA-15 with different loadings of HPMo on IL/SBA-15 were synthesized by adding 0.5 g IL/SBA-15 into 50 mL HPMo aqueous solution and stirring at room temperature for 12 h, where x represents the weight of HPMo in the samples. Then, the obtained samples were isolated by filtration and washed with deionized water. The resulting solid was dried in vacuum at 50 °C for 24 h. The weight contents of HPMo were 0.01, 0.03, 0.05, and 0.08 g.
2.1.5. Preparation of HPMo/SBA-15. Sample HPMo/SBA-15 was prepared similar to the aforementioned method. 0.5 g SBA-15 was added into 50 mL of deionized water containing 0.05 g phosphomolybdic acid, and the mixture was stirred at room temperature for 12 h. Then, the solid was isolated by filtration and washed with deionized water. The resulting solid was dried in vacuum at 50 °C for 24 h to produce HPMo/SBA-15.
2.2. Catalyst characterization
Fourier transform infrared (FTIR) spectra were acquired on a Nicolet FTIR spectrophotometer (Nexus 470, Thermo Electron Corporation) using KBr pellets at room-temperature. Powder X-ray diffraction (XRD) analysis was carried out on Bruker D8 diffractometer with high-intensity Cu Kα (λ = 1.54 Å). X-ray photoemission spectroscopy (XPS) was recorded on a VG MultiLab 2000 system with a monochromatic Mg-Kα source operated at 20 kV. The field-emission scanning electron microscopy (SEM) measurements were carried out with a field-emission scanning electron microscope (JEOL JSM-7001F) equipped with an energy-dispersive X-ray spectroscope (EDS) operated at an acceleration voltage of 10 kV. Transmission electron microscopy (TEM) micrographs were taken with a JEOL-JEM-2010 (JEOL, Japan) operating at 200 kV. The samples used for TEM were prepared by dispersing a small amount of the product in ethanol, then placing a drop of the solution onto a copper grid, and letting the ethanol evaporate slowly in the air. The N2 adsorption–desorption isotherms at 77 K were investigated using a TriStar II 3020 surface area and porosity analyzer (Micromeritics Instrument).
2.3. Preparation of model oil and desulfurization procedure
Model oil was prepared by dissolving DBT, BT, and 4,6-DMDBT in n-octane with their initial S-content of 500, 500, and 185 ppm, respectively. In a typical desulfurization experiment, 0.01 g catalyst and 5 mL model oil were added to a 40 mL two-necked flask. Then 30 wt% hydrogen peroxide was added within 10 min with magnetic stirring at the corresponding temperature. After the reaction, the concentration of sulfur-containing compounds in the model oil were analyzed by a Gas Chromatography-Flame Ionization Detector (GC-FID) with tetradecane as the internal standard (Agilent 7890A; HP-5, 30 m × 0.32 mm i.d. × 0.25 μm; FID: Agilent). Gas Chromatography-Mass Spectrometer (GC-MS) (Agilent 7890/5975C-Gas Chromatography (GC)/Mass Selective Detector (MSD); HP-5 MS column, 30 m × 250 μm i.d. × 0.25 μm; temperature program: 100 °C temperature rising 15 °C min−1 until 200 °C for 10 min) was also used to analyze the oxidized S-compounds after reaction.
3. Results and discussion
3.1. Characterization of the catalysts
Fig. 1 shows small-angle powder XRD patterns of the SBA-15 support and 0.05HPMo-IL/SBA-15 catalyst. As can be seen from the figure, the typical XRD pattern of SBA-15 consisted of a strong peak (2θ at around 1°) for d100 and two weak peaks (2θ at around 1.6°and 1.8°) for d110 and d200, which indicates a significant degree of long range ordering in the structure and a well-formed hexagonal p6mm lattice. When HPMo-IL was immobilized on SBA-15, all the three aforementioned peaks could also be observed, but the intensities were slightly decreased compared to pure SBA-15. The result indicates that the HPMo-IL was successfully grafted onto SBA-15 and retained the hexagonal mesostructure of SBA-15. The wide-angle XRD pattern of the HPMo and HPMo-IL/SBA-15 samples are presented in Fig. 2. No remarkable peaks of HPMo were found on the wide-angle XRD pattern of HPMo-IL/SBA-15 samples, which indicates that the HPMo may uniformly disperse on the SBA-15. After being immobilized, the ordered structure was further confirmed by TEM (Fig. 3). In order to ascertain the ionic liquid and HPMo grafting onto the SBA-15, the FTIR analysis was investigated. The FTIR spectra of SBA-15, IL/SBA, HPMo-IL/SBA-15, and HPMo are depicted in Fig. S1.† The absorption bands at 1085 and 804 cm−1 are attributed to the asymmetric and symmetric stretch of Si–O–Si, respectively.47,48 The bands at 960 cm−1 ascribed to the bending vibration of Si–OH.47,48 The absorption bands at 460 cm−1 are assigned to the Si–O bending vibration.47,48 Above these bands (1085, 804, 960, 460 cm−1) all were from SBA-15. In IL/SBA-15, besides the absorption bands of SBA-15, the bands of C
N and C
C vibrations (1571 cm−1), C–H stretching and deformation vibrations (3158, 2973, and 1450 cm−1) can be observed, which indicates the functionalization of ionic liquid into SBA-15. The characteristic bands of Keggin-type HPMo appeared at 1061, 960, 867, 782 cm−1.49 For HPMo-IL/SBA-15, both the characteristic bands of SBA-15 and IL could be found in it. Because the bands of 1061, 960, 782 cm−1 are masked by the bands of SBA-15, the characteristic bands of HPW can not be observed. Moreover, the band of 867 cm−1 appeared at HPMo-IL/SBA-15. However, it noted that a slight shift of 879 cm−1 characteristic peak appeared (Fig. S2†), which implies that HPMo was supported on IL/SBA-15 and the interactions were formed between HPMo and IL/SBA-15.
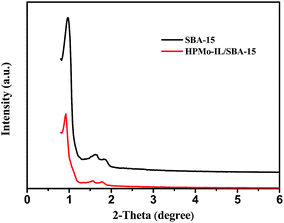 |
| Fig. 1 Low-angle XRD patterns of SBA-15 and 0.05HPMo-IL/SBA-15. | |
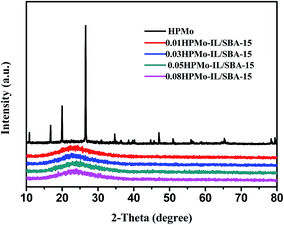 |
| Fig. 2 Wide-angle XRD patterns of different samples. | |
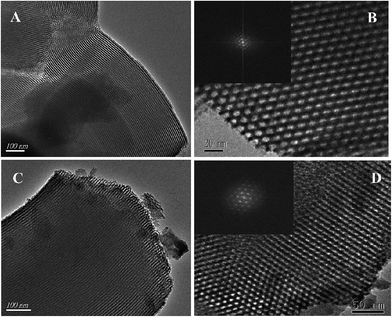 |
| Fig. 3 TEM images of SBA-15 (A and B) and 0.05HPMo-IL/SBA-15 (C and D). The insets are the corresponding FFT diffractograms. | |
The N2 adsorption–desorption isotherms of parent SBA-15 and HPMo-IL/SBA-15 with different HPMo catalyst loadings are shown in Fig. 4A. All the samples presented type IV isotherm patterns with an H1-type hysteresis loop with adsorption at relative pressures p/p0 = 0.5–0.80. It can be observed that, compared with parent SBA-15, the lower amount of adsorbed nitrogen and the shift of the hysteresis loop of the HPMo-based IL modified samples toward lower relative pressure, which indicates that the HPMo-based ionic liquid was successfully immobilized onto SBA-15 and the introduction of the HPMo-based ionic liquid significantly affected the surface area of SBA-15. The pore size distribution curves are shown in Fig. 4B. It was observed that all the samples have a narrow pore size distribution in the range 5–7.5 nm. The physical parameters of samples are also shown in Table 1.
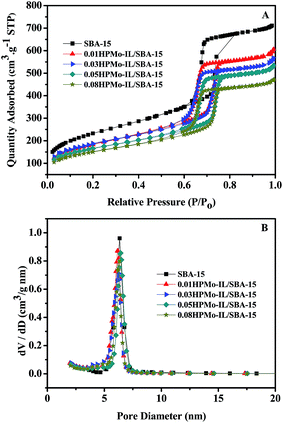 |
| Fig. 4 Nitrogen adsorption–desorption isotherms (A) and BJH pore size distributions (B) of SBA-15 and HPMo-IL/SBA-15 samples with different HPMo contents. | |
Table 1 The structural properties of the samples
Sample |
SBET (m2 g−1) |
Pore volume (cm3 g−1) |
Pore size (nm) |
SBA-15 |
842 |
1.01 |
5.6 |
0.01HPMo-IL/SBA-15 |
657 |
0.97 |
5.6 |
0.03HPMo-IL/SBA-15 |
583 |
0.92 |
5.6 |
0.05HPMo-IL/SBA-15 |
551 |
0.86 |
5.8 |
0.08HPMo-IL/SBA-15 |
531 |
0.76 |
5.6 |
The chemical state and surface composition of the catalyst are revealed by XPS. Fig. 5 shows the XPS spectrum of 0.05HPMo-IL/SBA-15. It shows that the 0.05HPMo-IL/SBA-15 sample was composed of the elements of Si, O, C, N, P and Mo (Fig. 5). The high-resolution spectra of these elements are presented in Fig. S3.† The bonding energy values corresponding to the O1s and Si2p appear at 532.8, 103.2 eV (Fig. S3†), respectively, which is attributed to support SBA-15. The C1s and N1s peaks could be attributed to the imidazole ionic liquid part in the sample. The P2p and Mo3d came from the HPMo. Therefore, it can be confirmed that the HPMo was successfully incorporated into IL functionalized SBA-15.
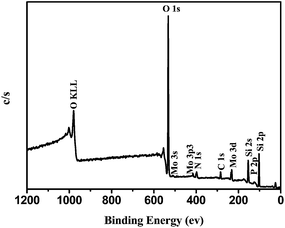 |
| Fig. 5 XPS spectrum of 0.05HPMo-IL/SBA-15. | |
SEM images of SBA-15 and HPMo-IL/SBA-15 catalysts are shown in Fig. S4.† SBA-15 shows the typical long fiber-like structure. After HPMo-IL was incorporated into the mesoporous sample, a fiber-like structure can also be seen, which demonstrated that the structure didn't change after being immobilized. The EDS analysis of the catalyst HPMo-IL/SBA-15 is shown in Fig. S4C.† It shows that C, N, O, Si, P, and Mo elements were contained in the catalysts, which also confirms the material was HPMo-IL/SBA-15.
3.2. Influence of different catalysts on the removal of DBT
HPMo, 0.05HPMo/SBA-15 and 0.05HPMo-IL/SBA-15 were investigated for their catalytic desulfurization performance. As shown in Fig. 6, when the HPMo was anchored on the mesoporous SBA-15, little catalytic performance for oxidative desulfurization was seen, whereas the incorporation of the imidazolium ionic liquid into the mesoporous SBA-15 support led to a drastic increase with the catalytic activity reaching above 90% sulfur removal. In order to explore the difference, contact angles analysis was carried out. When a water droplet was dropped to contact the surface of 0.05HPMo/SBA-15, the contact angle was zero (Fig. 7A). However, the contact angle was 52° for a water droplet in contact with the surface of 0.05HPMo-IL/SBA-15 (Fig. 7B). It has been reported that when a water droplet is dropped to contact the surface of the materials, and the contact angle is above 45° it indicates that the surfaces has more hydrophobic character; in contrast, contact angle below 45° exhibited that the surface has more hydrophilic character.50 Thus, compared with 0.05HPMo-SBA-15, IL incorporated into the SBA-15 of material, 0.05HPMo-IL/SBA-15, enhanced the hydrophobicity performance. According to “like dissolves like” principle, the HPMo-IL functionalized SBA-15 was hydrophobic, which was easier to provide a higher degree of exposure of the catalytic sites to the model oil. Therefore, the enhanced removal of DBT was strongly related to the unique feature of good miscibility of the hydrophobic HPMo-IL/SBA-15 for the reactants. For comparison, neat HPMo was investigated as a catalyst for oxidative desulfurization. The result showed that sulfur removal was only 16.4% in 90 min. At the same time, it can be observed that neat HPMo changed into liquid state in the reaction system. Thus, it can be concluded that HPMo-IL/SBA-15 was a high efficient heterogeneous catalyst for oxidative desulfurization.
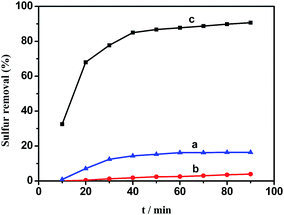 |
| Fig. 6 Oxidation removal of DBT on different catalysts HPMo (a), HPMo/SBA-15 (b) and HPMo-IL/SBA-15 (c). Experimental conditions: 5 mL model oil, m (catalyst) = 0.01 g, n (H2O2)/n (sulfur) = 2, T = 60 °C. | |
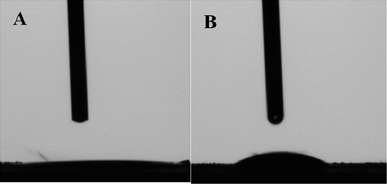 |
| Fig. 7 The contact angle of HPMo-SBA-15 (A) and HPMo-IL/SBA-15 (B). | |
It is also noteworthy to mention that, in this catalysis system H2O2 with a stoichiometric ratio (H2O2/sulfur mole ratio is 2) was used and 0.05HPMo-IL/SBA-15 could achieve above 90% sulfur removal. It was known that based on the reaction stoichiometry that 2 mol of H2O2 are consumed for oxidation of 1 mol DBT to their corresponding sulfones, DBTO2. In other desulfurization systems, the consumption of H2O2/sulfur mole ratio usually higher than 2. Apparently, HPMo-IL/SBA-15 exhibited excellent catalytic performance for the ODS reaction. The excellent activity could be attributed to (a) the larger surface area, which could ensure HPMo-IL good dispersion; (b) uniform pore channels, which is beneficial for mass transport; (c) good wettability for the model oil.
3.3. The effect of different parameters on sulfur removal
The effect of various HPMo loading amounts on the sulfur removal amount is shown in Fig. 8. It can be seen that the sulfur removal percentage increased as the supported loading amount of HPMo was increased. 0.05HPMo-IL/SBA-15 is the most active catalyst. However, when the HPMo loading amount was further increased, the sulfur removal decreased. Based on N2 adsorption–desorption analysis, the BET surface areas of xHPMo-IL/SBA-15 dropped from 657 to 531 m2 g−1 with the increasing amount of HPMo loading. It can be explained that the activity of the catalyst was the result of interaction of the total active centre and accessible surface area.
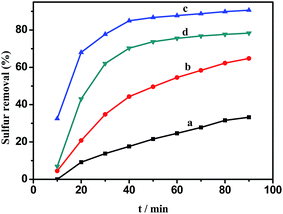 |
| Fig. 8 Oxidation removal of DBT of the HPMo-IL/SBA-15 catalysts with different HPMo loadings. (a) 0.01 HPMo-IL/SBA-15, (b) 0.03 HPMo-IL/SBA-15, (c) 0.05 HPMo-IL/SBA-15, (d) 0.08 HPMo-IL/SBA-15. Experimental conditions: 5 mL model oil, m (catalyst) = 0.01 g, n (H2O2)/n (sulfur) = 2, T = 60 °C. | |
Although, when H2O2/sulfur (O/S) molar ratio was 2, the removal of DBT could achieve 90.6%. When the amount of H2O2 is increased slightly up to an O/S molar ratio of 2.5, DBT can be completely removed in 60 min, (Fig. 9) which was also hard to realize in other reports. Similar catalytic oxidative desulfurization systems reported in previous studies are listed in Table S1.† As shown in this table, when the H2O2/DBT mole ratio was 2 (stoichiometric rate), the sulfur removal all hardly reached 90% (entries 1, 11, 14). It is noted that with increase in the H2O2/DBT mole ratio and reaction time, the sulfur removal could be improved and achieve beyond 90% (entries 3, 5, 6, 7, 9, 10, 13, 15). In the case of HPMo-IL/SBA-15 a low dosage of hydrogen peroxide could achieve deep desulfurization. Hence, HPMo-IL/SBA-15 is one of the most efficient catalytic oxidative desulfurization systems reported so far.
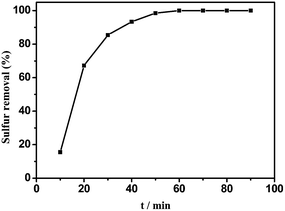 |
| Fig. 9 Oxidant removal of DBT at n (H2O2)/n (sulfur) = 2. Experimental condition: 5 mL model oil, m (0.05HPMo-IL/SBA-15) = 0.01 g, T = 60 °C. | |
The effect of temperature and time on the removal of DBT from the model oil is shown in Fig. 10. It can be seen that the temperature has a significant influence on sulfur removal. The reaction activity increased with increasing temperature. At low temperature (30 °C), DBT removal was only 19.8% in 90 min. However, 78.1% sulfur removal was achieved at 40 °C in 90 min. When the temperature increased to 60 °C, a high DBT removal (90.6%) was obtained. Therefore, 60 °C was chosen as the optimal temperature for further studies. The reaction data of oxidation of DBT with 0.05HPMo-IL/SBA-15 as the catalyst after 10 min at 50 and 60 °C was well described by the pseudo-first-order kinetic model. The first-order rate equation was ln(Ct/C0) = −kt, where C0 and Ct (mg L−1) are the concentration of sulfur at the initial time and t (min), respectively, and k (min−1) is the rate constant. The plots of ln(Ct/C0) vs. t are shown in Fig. S5.† The rate constants calculated from the slopes of the plots is 0.0308 and 0.04864 min−1 at 323 K and 333 K, respectively. The Arrhenius equation is
. It also can be expressed as
. The active energy Ea (slope = −Ea/R) can be derived from the plot of ln
k versus 1/T. Based on the data, an activation energy of 37.99 kJ mol−1 was obtained, which is similar with other reports about the phosphomolybdate/H2O2 systems for the removal of DBT.49
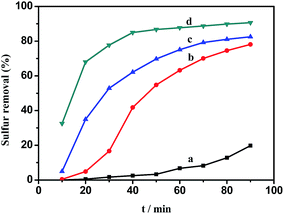 |
| Fig. 10 Effect of reaction temperature and time on removal of DBT. (a) 30 °C, (b) 40 °C, (c) 50 °C, (d) 60 °C. Experimental condition: 5 mL model oil, m (0.05HPMo-IL/SBA-15) = 0.01 g, n (H2O2)/n (sulfur) = 2. | |
The catalytic performances of 0.05HPMo-IL/SBA-15 with H2O2 as oxidant for different substrates, including DBT, BT and 4,6-DMDBT were evaluated. As shown in Fig. 11, it was evident that with BT and 4,6-DMDBT, sulfur removal reached 71.0% and 69.8% after 90 min, respectively, while the DBT reached above 90%. The sulfur removal of the three substrates decreased in the order DBT > BT > 4,6-DMDBT at 60 °C for 90 min under the same reaction conditions. The different removal rate of the three sulfur-containing compounds may be related to their electron density and the steric hindrance around the sulfur atoms. The electron densities on sulfur atoms are 5.739 for BT, 5.758 for DBT, and 5.760 for 4,6-DMDBT.51 For DBT and BT, the oxidative performance improved with increasing aromatic electron density. Compared with DBT and BT, 4,6-DMDBT has a higher electron density, but exhibited the lowest reactivity, which can be attributed to the steric hindrance of the methyl groups, which might be an obstacle for the sulfur atom to the approach active species. Therefore, the oxidative reactivity of different sulfur compounds followed the order DBT > BT > 4,6-DMDBT.
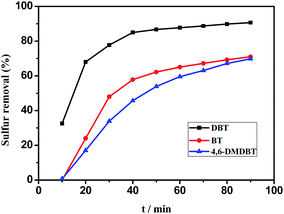 |
| Fig. 11 Influence of different substrates on sulfur removal. Experimental condition: 5 mL model oil, m (0.05HPMo-IL/SBA-15) = 0.01 g, n (H2O2)/n (sulfur) = 2, T = 60 °C. | |
3.4. Recycle experiment and desulfurization mechanism
The reusability of the 0.05HPMo-IL/SBA-15 catalyst was investigated in the catalytic ODS system (Fig. 12). After the oxidative desulfurization reaction, the model oil was decanted. Then, fresh model oil and H2O2 were added into the original reaction flask for the next run. The results indicated that the catalyst recycled three times without an activity loss. After sulfur removal, the catalyst still retained almost 100% of its activity. When the catalyst was recycled for the fifth time, the sulfur removal percentage decreased to 88.7%. After recycling five times, some white precipitates were clearly observed. The TEM images of used catalyst were recorded (Fig. S6†). It can be found that some materials are covered on the used catalyst. To understand this, the model oil and catalyst were analyzed by GC-MS after reaction. In the oil phase, the GC peak of DBT almost cannot be found, but a weak peak of DBTO2 can be observed (Fig. 13). When the used catalyst was washed by tetrachloromethane, a strong DBTO2 peak can be observed in tetrachloromethane (Fig.14). On the basis of the abovementioned analysis, it can be believed that DBT could be oxidized into DBTO2, and the product DBTO2 can be adsorbed onto the catalyst after reaction. The result demonstrates that HPMo-IL/SBA-15 was not only acting as a catalyst but also as an adsorbent. It can remove its own reaction product from fuel in a one-pot process. Surprisingly, after the used catalyst was washed by toluene, the ordered mesoporous structure was still clearly visible (Fig. S7†) and the sulfur removal percentage increased to 100% again.
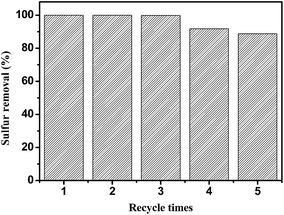 |
| Fig. 12 Recycle of the 0.05HPMo-IL/SBA-15 catalysts in desulfurization system. Experimental conditions: 5 mL model oil, m (0.05HPMo-IL/SBA-15) = 0.01 g, t = 90 min, n (H2O2)/n (sulfur) = 2, T = 60 °C. | |
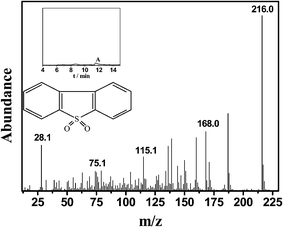 |
| Fig. 13 The mass spectrogram of oil phase after reaction. The inset is the total ion chromatograms (TIC). | |
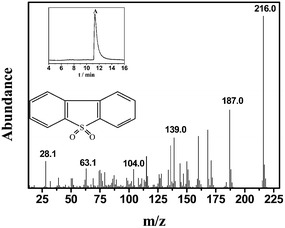 |
| Fig. 14 The mass spectrogram of extracted solution from used catalyst. The inset is the total ion chromatograms (TIC). | |
4. Conclusion
In summary, heteropoly acid HPMo was successfully immobilized onto ionic liquid-modified platelet SBA-15. The obtained catalyst displayed a typical mesoporous structure with HPMo and imidazole ionic liquid. The resulting catalysts exhibited hydrophobicity and good catalytic oxidative desulfurization ability under mild conditions. Under the optimal reaction conditions, the DBT removal reached up to 90.6% within the condition of O/S = 2. Subsequently, the solid catalyst was separated easily from the reaction system; moreover, after five times of regeneration, the activity of mesoporous HPMo-IL/SBA-15 showed only a slight decrease. The excellent recyclability of the catalyst is potentially important for industrial applications. After the reaction, the oxidant product can be adsorbed onto HPMo-IL/SBA-15. Therefore, the HPMo-IL/SBA-15 materials prepared in the present study are potential and efficient catalysts for the ODS of the fuel oils.
Acknowledgements
This study was financially supported by the National Nature Science Foundation of China (nos 21276117, 21376111, 21406092), the Doctoral Innovation Fund of Jiangsu Province (CXZZ13_0692) and the Postdoctoral Foundation of China (no. 2014M551516).
References
- V. C. Srivastava, An evaluation of desulfurization technologies for sulfur removal from liquid fuels, RSC Adv., 2012, 2, 759–783 RSC
. - X. X. Zhang, W. Zhang, D. Tian, Z. H. Zhou and C. H. Lu, A new application of ionic liquids for heterogeneously catalyzed acetylation of cellulose under solvent-free conditions, RSC Adv., 2013, 3, 7722–7725 RSC
. - D. Tian, Y. Y. Han, C. H. Lu, X. X. Zhang and G. P. Yuan, Acidic ionic liquid as “quasi-homogeneous” catalyst for controllable synthesis of cellulose acetate, Carbohydr. Polym., 2014, 113, 83–90 CrossRef CAS PubMed
. - L. Lizama and T. Klimova, Highly active deep HDS catalysts prepared using Mo and W heteropolyacids supported on SBA-15, Appl. Catal., B, 2008, 82, 139–150 CrossRef CAS PubMed
. - F. J. Méndez, A. Llanos, M. Echeverría, R. Jáuregui, Y. Villasana, Y. Díaz, G. Liendo-Polanco, M. A. Ramos-García, T. Zoltan and J. L. Brito, Mesoporous catalysts based on Keggin-type heteropolyacids supported on MCM-41 and their application in thiophene hydrodesulfurization, Fuel, 2013, 110, 249–258 CrossRef PubMed
. - L. Yang, X. Li, A. J. Wang, R. Prins, Y. Wang, Y. Y. Chen and X. P. Duan, Hydrodesulfurization of 4,6-dimethyldibenzothiophene and its hydrogenated intermediates over bulk Ni2P, J. Catal., 2014, 317, 144–152 CrossRef CAS PubMed
. - J. Xiong, W. S. Zhu, H. M. Li, Y. H. Xu, W. Jiang, S. H. Xun, H. Liu and Z. Zhao, Immobilized Fenton-Like Ionic Liquid: Catalytic Performance for Oxidative Desulfurization, AIChE J., 2013, 59, 4696–4704 CrossRef CAS
. - M. Zhang, W. S. Zhu, H. M. Li, S. H. Xun, W. J. Ding, J. J. Liu, Z. Zhao and Q. Wang, One-pot synthesis, characterization and desulfurization of functional mesoporous W-MCM-41 from POM-based ionic liquids, Chem. Eng. J., 2014, 243, 386–393 CrossRef CAS PubMed
. - J. Xiao, L. M. Wu, Y. Wu, B. Liu, L. Dai, Z. Li, Q. B. Xia and H. X. Xi, Effect of gasoline composition on oxidative desulfurization using a phosphotungstic acid/activated carbon catalyst with hydrogen peroxide, Appl. Energy, 2014, 113, 78–85 CrossRef CAS PubMed
. - F. S. Mjalli, O. U. Ahmed, T. Al-Wahaibi, Y. Al-Wahaibi and I. M. AlNashef, Deep oxidative desulfurization of liquid fuels, Rev. Chem. Eng., 2014, 30, 337–378 CAS
. - W. H. Lo, H. Y. Yang and G. T. Wei, One-pot desulfurization of light oils by chemical oxidation and solvent extraction with room temperature ionic liquids, Green Chem., 2003, 5, 639–642 RSC
. - F. Al-Shahrani, T. C. Xiao, S. A. Llewellyn, S. Barri, Z. Jiang, H. H. Shi, G. Martinie and M. L. H. Green, Desulfurization of diesel via the H2O2 oxidation of aromatic sulfides to sulfones using a tungstate catalyst, Appl. Catal., B, 2007, 73, 311–316 CrossRef CAS PubMed
. - W. H. Wang, G. Li, W. G. Li and L. P. Liu, Synthesis of hierarchical TS-1 by caramel templating, Chem. Commun., 2011, 47, 3529–3531 RSC
. - A. Sengupta, P. D. Kamble, J. K. Basu and S. Sengupta, Kinetic Study and Optimization of Oxidative Desulfurization of Benzothiophene Using Mesoporous Titanium Silicate-1 Catalyst, Ind. Eng. Chem. Res., 2012, 51, 147–157 CrossRef CAS
. - H. S. Gao, C. Guo, J. M. Xing, J. M. Zhao and H. Z. Liu, Extraction and oxidative desulfurization of diesel fuel catalyzed by a Brønsted acidic ionic liquid at room temperature, Green Chem., 2010, 12, 1220–1224 RSC
. - Y. Nie, Y. X. Dong, L. Bai, H. F. Dong and X. P. Zhang, Fast oxidative desulfurization of fuel oil using dialkylpyridinium tetrachloroferrates ionic liquids, Fuel, 2013, 103, 997–1002 CrossRef CAS PubMed
. - J. H. Xu, S. Zhao, Y. C. Ji and Y. F. Song, Deep Desulfurization by Amphiphilic Lanthanide-Containing Polyoxometalates in Ionic-Liquid Emulsion Systems under Mild Conditions, Chem.–Eur. J., 2013, 19, 709–715 CrossRef CAS PubMed
. - W. S. Zhu, P. W. Wu, L. Yang, Y. H. Chang, Y. H. Chao, H. M. Li, Y. Q. Jiang, W. Jiang and S. H. Xun, Pyridinium-Based Temperature-responsive Magnetic Ionic Liquid for Oxidative Desulfurization of Fuels, Chem. Eng. J., 2013, 229, 250–256 CrossRef CAS PubMed
. - W. S. Zhu, J. T. Zhang, H. M. Li, Y. H. Chao, W. Jiang, S. Yin and H. Liu, Fenton-like ionic liquids/H2O2 system: one-pot extraction combined with oxidation desulfurization of fuel, RSC Adv., 2012, 2, 658–664 RSC
. - J. Xiong, W. S. Zhu, W. J. Ding, L. Yang, Y. H. Chao, H. P. Li, F. X. Zhu and H. M. Li, Phosphotungstic Acid Immobilized on Ionic Liquid-Modified SBA-15: Efficient Hydrophobic Heterogeneous Catalyst for Oxidative Desulfurization in Fuel, Ind. Eng. Chem. Res., 2014, 53, 19895–19904 CrossRef CAS
. - L. S. Nogueira, S. Ribeiro, C. M. Granadeiro, E. Pereira, G. Feio, L. Cunha-Silva and S. S. Balula, Novel polyoxometalate silica nano-sized spheres: efficient catalysts for olefin oxidation and the deep desulfurization process, Dalton Trans., 2014, 43, 9518–9528 RSC
. - X. M. Zhou, W. Chen and Y. F. Song, Superhydrophobic Polyoxometalate/Calixarene Inorganic–Organic Hybrid Materials with Highly Efficient Desulfurization Ability, Eur. J. Inorg. Chem., 2014, 2014, 812–817 CrossRef CAS
. - M. Chamack, A. R. Mahjoub and H. Aghayan, Cesium salts of tungsten-substituted molybdophosphoric acid immobilized onto platelet mesoporous silica: Efficient catalysts for oxidative desulfurization of dibenzothiophene, Chem. Eng. J., 2014, 255, 686–694 CrossRef CAS PubMed
. - W. S. Zhu, H. M. Li, Q. Q. Gu, P. W. Wu, G. P. Zhu, Y. S. Yan and G. Y. Chen, Kinetics and mechanism for oxidative desulfurization of fuels catalyzed by peroxo-molybdenum amino acid complexes in water-immiscible ionic liquids, J. Mol. Catal. A: Chem., 2011, 336, 16–22 CrossRef CAS PubMed
. - W. S. Zhu, B. L. Dai, P. W. Wu, Y. H. Chao, J. Xiong, S. H. Xun, H. P. Li and H. M. Li, Graphene-Analogue Hexagonal BN Supported with Tungsten-based Ionic Liquid for Oxidative Desulfurization of Fuels, ACS Sustainable Chem. Eng., 2015, 3, 186–194 CrossRef CAS
. - J. Dou and H. C. Zeng, Integrated Networks of Mesoporous Silica Nanowires and Their Bifunctional Catalysis–Sorption Application for Oxidative Desulfurization, ACS Catal., 2014, 4, 566–576 CrossRef CAS
. - W. Zhang, H. Zhang, J. Xiao, Z. X. Zhao, M. X. Yu and Z. Li, Carbon nanotube catalysts for oxidative desulfurization of a model diesel fuel using molecular oxygen, Green Chem., 2014, 16, 211–220 RSC
. - H. Y. Lü, J. B. Gao, Z. X. Jiang, Y. X. Yang, B. Song and C. Li, Oxidative desulfurization of dibenzothiophene with molecular oxygen using emulsion catalysis, Chem. Commun., 2007, 150–152 Search PubMed
. - R. Tan, C. Liu, N. D. Feng, J. Xiao, W. G. Zheng, A. M. Zheng and D. H. Yin, Phosphotungstic acid loaded on hydrophilic ionic liquid modified SBA-15 for selective oxidation of alcohols with aqueous H2O2, Microporous Mesoporous Mater., 2012, 158, 77–87 CrossRef CAS PubMed
. - A. Bordoloi, S. Sahoo, F. Lefebvre and S. B. Halligudi, Heteropoly acid-based supported ionic liquid-phase catalyst for the selective oxidation of alcohols, J. Catal., 2008, 259, 232–239 CrossRef CAS PubMed
. - Z. Karimi, A. R. Mahjoub and S. M. Harati, Polyoxometalate-based hybrid mesostructured catalysts for green epoxidation of olefins, Inorg. Chim. Acta, 2011, 376, 1–9 CrossRef CAS PubMed
. - A. Nisar, J. Zhuang and X. Wang, Construction of Amphiphilic Polyoxometalate Mesostructures as a Highly Efficient Desulfurization Catalyst, Adv. Mater., 2011, 23, 1130–1135 CrossRef CAS PubMed
. - A. Nisar, Y. Lu, J. Zhuang and X. Wang, Polyoxometalate Nanocone Nanoreactors: Magnetic Manipulation and Enhanced Catalytic Performance, Angew. Chem., Int. Ed., 2011, 50, 3187–3192 CrossRef CAS PubMed
. - D. Huang, Y. C. Lu, Y. J. Wang and G. S. Luo, Catalytic Kinetics of Dibenzothiophene Oxidation with the Combined Catalyst of Quaternary Ammonium Bromide and Phosphotungstic Acid, Ind. Eng. Chem. Res., 2007, 46, 6221–6227 CrossRef CAS
. - Z. E. A. Abdalla and B. S. Li, Preparation of MCM-41 supported (Bu4N)4H3(PW11O39) catalyst and its performance in oxidative desulfurization, Chem. Eng. J., 2012, 200–202, 113–121 CrossRef CAS PubMed
. - Y. H. Ren, B. Yue, M. Gu and H. Y. He, Progress of the Application of Mesoporous Silica-Supported Heteropolyacids in Heterogeneous Catalysis and Preparation of Nanostructured Metal Oxides, Materials, 2010, 3, 764–785 CrossRef CAS PubMed
. - S. Benadji, P. Eloy, A. Leonard, B. L. Su, C. Rabia and E. M. Gaigneaux, Characterization of H3+xPMo12−xVxO40 heteropolyacids supported on HMS mesoporous molecular sieve and their catalytic performance in propene oxidation, Microporous Mesoporous Mater., 2012, 154, 153–163 CrossRef CAS PubMed
. - J. Zhang, A. J. Wang, Y. J. Wang, H. Y. Wang and J. Z. Gui, Heterogeneous oxidative desulfurization of diesel oil by hydrogen peroxide: catalysis of an amphipathic hybrid material supported on SiO2, Chem. Eng. J., 2014, 245, 65–70 CrossRef CAS PubMed
. - X. M. Yan, P. Mei, J. H. Lei, Y. Z. Mi, L. Xiong and L. P. Guo, Synthesis and characterization of mesoporous phosphotungstic acid/TiO2 nanocomposite as a novel oxidative desulfurization catalyst, J. Mol. Catal. A: Chem., 2009, 304, 52–57 CrossRef CAS PubMed
. - X. F. Hu, Y. K. Lu, F. N. Dai, C. G. Liu and Y. Q. Liu, Host–guest synthesis and encapsulation of phosphotungstic acid in MIL-101 via “bottle around ship”: an effective catalyst for oxidative desulfurization, Microporous Mesoporous Mater., 2013, 170, 36–44 CrossRef CAS PubMed
. - S. Ribeiro, C. M. Granadeiro, P. Silva, F. A. A. Paz, F. F. de Biani, L. Cunha-Silvaa and S. S. Balula, An efficient oxidative desulfurization process using terbium-polyoxometalate@MIL-101(Cr), Catal. Sci. Technol., 2013, 3, 2404–2414 CAS
. - D. Y. Zhao, Q. S. Huo, J. L. Feng, B. F. Chmelka and G. D. Stucky, Nonionic triblock and star diblock copolymer and oligomeric surfactant syntheses of highly ordered, hydrothermally stable, mesoporous silica structures, J. Am. Chem. Soc., 1998, 120, 6024–6036 CrossRef CAS
. - K. S. Cho and Y. K. Lee, Effects of nitrogen compounds, aromatics, and aprotic solvents on the oxidative desulfurization (ODS) of light cycle oil over Ti-SBA-15 catalyst, Appl. Catal., B, 2014, 147, 35–42 CrossRef CAS PubMed
. - T. Ressler, U. Dorn, A. Walter, S. Schwarz and A. H. P. Hahn, Structure and properties of PVMo11O40 heteropolyoxomolybdate supported on silica SBA-15 as selective oxidation catalyst, J. Catal., 2010, 275, 1–10 CrossRef CAS PubMed
. - B. Karimi and M. Khorasani, Selectivity Adjustment of SBA-15 Based Tungstate Catalyst in Oxidation of Sulfides by Incorporating a Hydrophobic Organic Group inside the Mesochannels, ACS Catal., 2013, 3, 1657–1664 CrossRef CAS
. - W. H. Tian, L. B. Sun, X. L. Song, X. Q. Liu, Y. Yin and G. S. He, Adsorptive Desulfurization by Copper Species within Confined Space, Langmuir, 2010, 26, 17398–17404 CrossRef CAS PubMed
. - J. Xiong, W. S. Zhu, W. J. Ding, P. Wang, Y. H. Chao, M. Zhang, F. X. Zhu and H. M. Li, Controllable synthesis of functionalized ordered
mesoporous silica by metal-based ionic liquids, and their effective adsorption of dibenzothiophene, RSC Adv., 2014, 4, 40588–40594 RSC
. - X. C. Shao, X. T. Zhang, W. G. Yu, Y. Y. Wu, Y. C. Qin, Z. L. Sun and L. J. Song, Effects of surface acidities of MCM-41 modified with MoO3 on adsorptive desulfurization of gasoline, Appl. Surf. Sci., 2012, 263, 1–7 CrossRef CAS PubMed
. - J. H. Qiu, G. H. Wang, D. L. Zeng, Y. Tang, M. Wang and Y. J. Li, Oxidative desulfurization of diesel fuel using amphiphilic quaternary ammonium phosphomolybdate catalysts, Fuel Process. Technol., 2009, 90, 1538–1542 CrossRef CAS PubMed
. - R. V. Mambrini, A. L. M. Saldanha, J. D. Ardisson, M. H. Araujo and F. C. C. Moura, Adsorption of sulfur and nitrogen compounds on hydrophobic bentonite, Appl. Clay Sci., 2013, 83–84, 286–293 CrossRef CAS PubMed
. - S. Otsuki, T. Nonaka, N. Takashima, W. H. Qian, A. Ishihara, T. Imai and T. Kabe, Oxidative desulfurization of light gas oil and vacuum gas oil by oxidation and solvent extraction, Energy Fuels, 2000, 14, 1232–1239 CrossRef CAS
.
Footnote |
† Electronic supplementary information (ESI) available: See DOI: 10.1039/c4ra14382e |
|
This journal is © The Royal Society of Chemistry 2015 |
Click here to see how this site uses Cookies. View our privacy policy here.