DOI:
10.1039/C4RA15115A
(Communication)
RSC Adv., 2015,
5, 12592-12596
Multifunctional, marvelous polyimide aerogels as highly efficient and recyclable sorbents†
Received
24th November 2014
, Accepted 15th January 2015
First published on 15th January 2015
Abstract
With the increasing awareness of environmental protection, it is imperative to develop advanced materials that can clean up spilled oil and organic contaminants efficiently. Herein, we designed and fabricated a series of novel polyimide (PI) aerogels by freeze-drying. The PI aerogels exhibit excellent absorption capability. The toluene absorption capability even ranked first among reported organic absorbents. The PI aerogels also exhibit good absorption recyclability. Surprisingly, the PI aerogels show high flexibility in liquid nitrogen, and liquid nitrogen absorbed by PI aerogels can be squeezed out repeatedly as if it were water in a sponge. What is more, PI aerogels are also usable as sorbents at high temperature and in harsh acid. These desirable attributes would enable PI aerogels to act as ideal sorbents in extreme environments. Together with thermal insulation and fire-resistance, the multifunctional, marvelous PI aerogels are expected to further extend their practical applications.
Introduction
Environmental protection and water recycling draw increasing awareness nowadays.1–6 Owing to the possibility of absorbing, removing, and transferring oil and organic liquids from the surface of water, organic absorbent materials (natural fiber materials and synthetic polymers) have been widely used. With economic and environmental concerns, natural fiber materials are preferred,7,8 however, they are mostly hydrophilic and usually show relatively low sorption capacities. Synthetic polymers are high cost with complicated processing and often absorb slowly with poor recyclability.9–11 What is more, these conventional organic absorbent materials are unifunctional and cannot be used in extreme environments, such as ultra-high/low temperature and acid, restricting their application in aerospace, industry, and daily life. Therefore, demand for finding novel multifunctional materials that can clean up spilled oil and organic contaminants efficiently, immediately and repeatedly in extreme environments with low cost, and environmental friendliness is highly imperative.
With superior mechanical properties, excellent thermal stability, high glass transition temperature, as well as outstanding resistance to solvent and radiation, polyimide (PI), an important high performance plastic, is widely applied in the automotive, aerospace, railway transport, and microelectronic fields.12 Given its unique multifunctional attributes, PI is considered as an attractive candidate to make aerogel with high-efficient and recyclable absorption. As far as we know, the use of PI as a sorbent material hasn't been reported previously.
Herein, we present a facile method to synthesis environmental benign, multifunctional PI aerogels from a poly(amic acid) ammonium salt (PAS) (a precursor of PI)13 with high absorption capacity and low-cost. As is known, the control of pore structure would be very important because of its relation to properties. Freeze-drying, an environmental friendly and cost-effective shaping process for advanced materials with interconnecting pore channels or pore gradients in bodies,14–16 has emerged as a good candidate for preparing aerogels. The combination of PI with facile and versatile freeze-drying method would realize structural and functional integrity, and thus brings a new opportunity of developing novel PI aerogels with remarkable multifunction.
Experimental
Preparation of PI aerogels via the freeze-drying method
4,4′-ODA and DMAc were added into a 100 mL three-neck round-bottom flask fitted with a mechanical stirrer. Once the ODA had dissolved completely, equivalent molar quantity of PMDA was added, and the mixture was stirred for 4 h. A poly(amic acid) (PAA) solution with a solid content of 12 wt% was obtained after continued stirring of the reaction mixture for another 4 h. Then, the resultant solution was poured into deionized water and deposited. After washing, drying, and crushing, PAA powder was obtained. The ammonium salt solution of PAA was prepared from PAA (0.2 g) and triethylamine (TEA, 0.096 g) in deionized water (29.3 g) (solid content of 1%). Solid content of 3%, 5%, 8%, 10%, 15% were also made. The solutions were continuously stirred at room temperature until they became homogeneous and transparent. Afterward, the resulting suspensions were transferred to 5 mL cylindrical vials, frozen in a laboratory freezer, approximately −18 °C, and dried in a Christ freeze-dryer. After 24–36 h in the lyophilizer, the samples were removed and heated for 1 h at 100, 120, 150 °C, 0.5 h at 180 °C, 1 h at 250 and 300 °C gradually.
Characterizations and Instruments
A Quanta 250 FEG scanning electron microscopy (SEM) system was used to observe the aerogel microstructures. The wetting properties of different samples were evaluated through contact angle tests, which were performed by the DCAT21 contact angle analysis system at room temperature. Densities of the PI aerogels were calculated by dividing bulk mass by bulk volume. Bulk mass of the PI aerogels were measured using an electronic balance, bulk volume of the PI aerogels were calculated by using a slide caliper to measure the dimensions and heights. The compressive tests of the PI aerogels were performed by using an Instron 5567 testing machine, equipped with two flat-surface compression stages. The strain ramp rate was maintained at 0.8 mm min−1 for all of the tests. Thermogravimetric analysis (TGA) was conducted on a TA Instruments NETZSCH5 thermogravimetric analyzer at a heating rate of 10 °C min−1 from 40 to 800 °C under air atmosphere.
Organic liquids absorption of PI aerogels
We measured the absorption capacities (Q) of PI aerogels for various organic solvents and oils with different densities, including n-hexane, cyclohexane, acetone, ethanol, methanol, toluene, pump oil, THF, Arawana cooking oil, DMAc, DMF, glycerol, chloroform. The PI aerogels were placed inside the organic liquids, and picked out for measurements. The PI aerogels weights after and before absorption were recorded for calculating Q value. In order to avoid evaporation of absorbed organic liquids, weight measurements should be done quickly. The absorption capacities (Q) of the PI aerogels were calculated using the following equation:where S0 is the initial dry weight of the PI aerogel and St is the weight of the PI aerogel with organic liquid absorbed.
Results and discussion
As most of the pore forming agent of freeze-drying method is water, but the commonly used PI precursor poly(amic acid) (PAA) is water-insoluble, to address this issue, water-soluble PI precursor PAS13,17 solution is made (Fig. S1†). After freeze-drying and imidizing subsequently, PI aerogels are prepared (Fig. S2†). Porous structures and high mechanic properties are necessary prerequisites for oil spill cleanup sorbents. As is seen, PI aerogel with low density (Fig. S3†) can stand stably on the top of a dandelion, without deforming the dandelion at all (Fig. 1a), which is attributed to low density and high porosity of PI aerogel. Porous structures can be shaped easily by adjusting the solid content. As demonstrated by SEM images, the PI aerogels exhibit lamellar (Fig. 1b), interconnected, crinkly (Fig. 1c), honeycomb structures (Fig. 1d) with macropores ranged from hundreds of nanometers to tens of micrometers. Regardless of their porous structures, the PI aerogels exhibit high mechanic properties. The springy PI aerogels can be bent to a large degree (Fig. 1e) and compressed to about 80% (Fig. 1f, Movie 1†) for many times without breaking apart, and after that still recover to nearly original shape, demonstrating excellent mechanic property (Fig. S4†). The PI aerogels are of highly hydrophobic (Fig. S5†) with macropore, possessing super-high absorption capacities and ultrafast absorption rate for organic solvents and oils. As an example, the PI aerogel selectively absorb a layer of Arawana cooking oil (labeled with oil red O dye) on the water surface in seconds under hydrophobic and capillary effects (Fig. 2a). The PI aerogel floated on the water surface and moved freely throughout the oil area. Whenever the PI aerogel came into contact with the oil, it instantaneously absorbed the oil film, resulting in a local white-colored region that indicated the presence of filtered water. The PI aerogel tended to drift on the remaining area of the oil film due to its water-repelling and oil-wetting properties, which leads to a unique floating-and-cleaning capability that is particularly useful for cleaning up oil spills.18 Excellent selective absorption of PI aerogel was demonstrated by putting the sample into a beaker containing the mixture of Arawana cooking oil and water (Fig. 2b and Movie 2†). Then, the sample was briefly vortexed to shake the mixture with a rotor, mimicking the motion of ocean waves. The results illustrate that all of the oils can be completely absorbed into the PI aerogel within a few seconds. In order to characterize the absorption capacities (defined by Q, the ratio between the final and initial weight after full absorption), various organic liquids were evaluated in this study, e.g., pump oil, Arawana cooking oil, and cyclohexane. These materials are common pollutants in daily life or industry. Organic solvents, such as toluene, alcohol, and acetone are also used for experimentation. PI aerogel at a solid content of 1% showed excellent absorption of these liquids (Fig. 2c), In general, the Q of it ranged from 30 to 195 times its own weight. The mechanism is mainly physical absorption of organic molecules which can be stored in the aerogel pores.19,20 Owing to the low density (Fig. S3†) and high porosity, the Q values are significantly higher than the weight gains of other organic sorbent. We took the volatile organic compound toluene as an example (Fig. 2d), to our knowledge, the absorption capacity of PI aerogel at a solid content of 1% ranked first among organic absorbent materials reported to date for toluene.9,10,18,21–28 Surprisingly, the absorption capacity for toluene of PI aerogel at a solid content of 1% is even much higher than that of some graphene aerogels.29–31
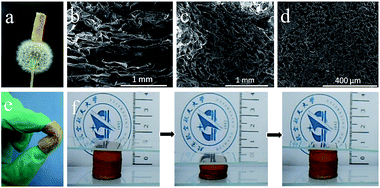 |
| Fig. 1 (a) A 2.0 cm3 PI aerogel at a solid content of 1% rested on a dandelion. SEM images of PI aerogel at a solid content of (b) 1%; (c) 10%; (d) 15%. (e) A PI aerogel bent to arch-shape at a large-angle by finger tips. (f) Images of PI aerogel (from left to right), before compression, being compressed, and after releasing of pressure. | |
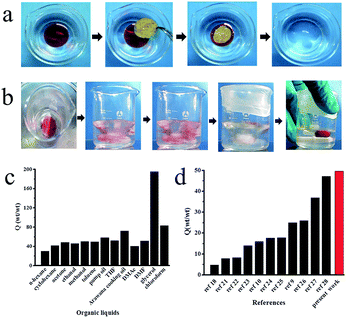 |
| Fig. 2 Uptake of organic liquids by the PI aerogels. (a) A layer of Arawana cooking oil (colored with oil red O for clear presentation) was absorbed by a PI aerogel completely in seconds. (b) Absorption process of the PI aerogel in a mixture of Arawana cooking oil (colored with oil red O for clear presentation) and water under vigorous stirring. (c) Absorption efficiency of PI aerogel at a solid content of 1% for various organic liquids. (d) Toluene absorption capacities for various organic absorbent materials. | |
The recyclability of sorbents and the recoverability of pollutants are key criteria for oil/chemical cleanup application. As the PI aerogels are mechanic robust, and durable to high temperature, direct combustion, simple squeezing and distillation were applied to recycle pollutants absorbed by the PI aerogels (Fig. 3a). Take cyclohexane as an example, the absorption capacities decreased with the number of direct combustion cycles (Fig. 3b), because the PI aerogels became smaller during the combustion process. However, the 3D networks of the PI aerogel still remained after absorption and combustion, thus making it recyclable for many times.20,32
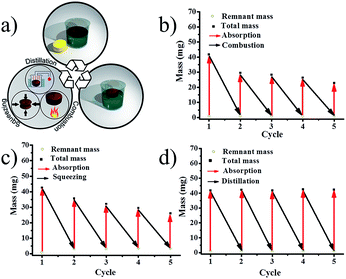 |
| Fig. 3 Absorption recyclability of PI aerogels. (a) Schematic diagram of PI aerogels recycling process. PI aerogels can be regenerated and reused using the direct combustion, simple squeezing and distillation method independently after absorption. Recyclability of PI aerogels for absorption of cyclohexane when using the direct combustion (b), simple squeezing (c) and distillation (d) method. | |
Interestingly, a simple squeezing operation was efficient to remove most of the cyclohexane absorbed in the pores of the aerogels due to the springy nature of PI aerogels (Fig. 3c and movie 3†). The absorption capacities of the aerogel for cyclohexane kept 26 even after five absorption/squeezing cycles, exhibiting good recyclability.
The decrease of cyclohexane-absorption capacities were caused by the residual cyclohexane in the pores of the aerogel.33 Pollution control and environmental protection efforts require that pollutants are not only absorbed and prevented from further harming the environment, but also properly recycled and thus reused, as they are either precious or toxic raw materials, e.g., pump oil and toluene. To meet the abovementioned need, distillation was employed as an alternative method for recycling (Fig. 3d). After absorption, the sample was heated to the boiling point to release the absorbed liquid. Then, we collected the vapor of the liquid for recycling. No obvious change in absorption capacity was found after five absorption/distillation cycles, indicating the highly stable recycling performance, because the size and the porosity structure stayed the same.20,34 The results clearly show the good recyclability of the PI aerogels when used as an absorbent. Among these three methods for recycling abovementioned, distillation is the most environmentally friendly and high-efficient to recycle the contaminant.
Not only being able to absorb oils at ambient temperatures, PI aerogel is also usable as a sorbent under harsh conditions. The PI aerogel shows high flexibility in liquid nitrogen (Fig. 4a and Movie 4†), and liquid nitrogen absorbed by PI aerogel can be squeezed out repeatedly as if it were water in a sponge. In addition, glycerol can be absorbed by PI aerogels at 260 °C easily without damaging the frameworks (Fig. 4b). As a result, PI aerogels can be used at a wide operational temperature range from −196 °C to 260 °C. Surprisingly, being immersed completely in harsh acid for 24 h, no obvious damage was observed in PI aerogel (Fig. 4c), demonstrating excellent acid-proof property. The temperature-variant absorption, acid-proof property and very low density make PI aerogels attractive sorbent materials to meet the extremely environmental oil spill cleanup needs of a number of special fields. What's more, PI aerogel possesses superior thermal properties, such as thermal insulation and thermal stability (Fig. 4d and S6†), and fire-resistance properties (Fig. 4e), making it a promising reusable oil sorbent in thermal insulation and fire safety field. Thus, the multifunctional, marvelous PI aerogels will enable a variety of applications.
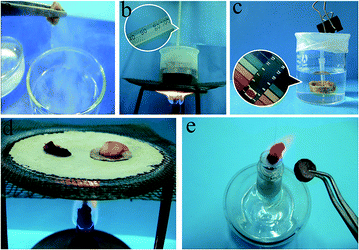 |
| Fig. 4 Multifunctional PI aerogels. (a) Liquid nitrogen absorbed by the PI aerogel can be squeezed out easily. (b) The PI aerogel can be used to absorb glycerol at 260 °C without breaking apart. (c) The PI aerogel can be immersed completely in harsh acid for 24 h without any damaging. (d) Two pieces of rose flower from the same rose were placed simultaneously on an asbestos network which is above a hot flame, and the left piece of rose flower was charred completely, but another piece of rose flower on a PI aerogel without obviously damaging after baking. (e) After contacting with a hot flame of an alcohol burner, the PI aerogel was taken away, it self-extinct immediately. | |
Conclusions
In summary, we report a facile, versatile and environmentally benign method to fabricate PI aerogels with high performance. The absorption capability of the PI aerogel can be as high as 30–195 times its own weight for organic pollutants and oils, which is higher than that of other organic absorbents. The toluene absorption capability even ranked first among reported organic absorbents. The PI aerogels exhibit good recyclability, and distillation is the most environmentally friendly and high-efficient method to recycle spill oils and organic solvents. The PI aerogels are also usable as sorbents under harsh conditions, e.g., ultra-low, high temperature and harsh acid. These desirable attributes would enable PI aerogels as ideal sorbents in extreme environment. Together with thermal insulation and fire-resistance, the unique multifunctional PI aerogels are expected to further extend the practical applications.
Acknowledgements
This work is financially supported by the National Natural Science Foundation of China (no. 51373007, no. 51003004, no. 51173188), the Beijing Natural Science Foundation (no. 2142019), the National Research Fund for Fundamental Key Projects (no. 2010CB934701, no. 2014CB643605), the Fundamental Research Funds for the Central Universities, and the SRF for ROCS, SEM.
Notes and references
- M. A. Shannon, P. W. Bohn, M. Elimelech, J. G. Georgiadis, B. J. Mariñas and A. M. Mayes, Nature, 2008, 452, 301 CrossRef CAS PubMed.
- C. H. Peterson, S. D. Rice, J. W. Short, D. Esler, J. L. Bodkin, B. E. Ballachey and D. B. Irons, Science, 2003, 302, 2082 CrossRef CAS PubMed.
- A. Jernelöv, Nature, 2010, 466, 182 CrossRef PubMed.
- D. D. Nguyen, N.-H. Tai, S.-B. Lee and W.-S. Kuo, Energy Environ. Sci., 2012, 5, 7908 CAS.
- B. Wang, W. Liang, Z. Guo and W. Liu, Chem. Soc. Rev., 2015, 44, 336 RSC.
- V. Chabot, D. Higgins, A. Yu, X. Xiao, Z. Chen and J. Zhang, Energy Environ. Sci., 2014, 7, 1564 CAS.
- G. Deschamps, H. Caruel, M.-E. Borredon, C. Bonnin and C. Vignoles, Environ. Sci. Technol., 2003, 37, 1013 CrossRef CAS.
- V. Singh, R. J. Kendall, K. Hake and S. Ramkumar, Ind. Eng. Chem. Res., 2013, 52, 6277 CrossRef CAS.
- T. Ono, T. Sugimoto, S. Shinkai and K. Sada, Nat. Mater., 2007, 6, 429 CrossRef CAS PubMed.
- T. Ono, T. Sugimoto, S. Shinkai and K. Sada, Adv. Funct. Mater., 2008, 18, 3936 CrossRef CAS.
- Z. Xue, S. Wang, L. Lin, L. Chen, M. Liu, L. Feng and L. Jiang, Adv. Mater., 2011, 23, 4270 CrossRef CAS.
- K. L. Mittal, Polyimides and Other High Temperature Polymers: Synthesis, Characterization and Applications, VSP International Science Publishers, 2009, vol. 5 Search PubMed.
- L. Zhang, J. Wu, N. Sun, X. Zhang and L. Jiang, J. Mater. Chem. A, 2014, 2, 7666 CAS.
- S. Deville, E. Saiz, R. K. Nalla and A. P. Tomsia, Science, 2006, 311, 515 CrossRef CAS PubMed.
- H. Zhang, I. Hussain, M. Brust, M. F. Butler, S. P. Rannard and A. I. Cooper, Nat. Mater., 2005, 4, 787 CrossRef CAS PubMed.
- H. Hu, Z. Zhao, W. Wan, Y. Gogotsi and J. Qiu, Adv. Mater., 2013, 25, 2219 CrossRef CAS PubMed.
- J. V. Facinelli, S. L. Gardner, L. Dong, C. L. Sensenich, R. M. Davis and J. S. Riffle, Macromolecules, 1996, 29, 7342 CrossRef CAS.
- S.-J. Choi, T.-H. Kwon, H. Im, D.-I. Moon, D. J. Baek, M.-L. Seol, J. P. Duarte and Y.-K. Choi, ACS Appl. Mater. Interfaces, 2011, 3, 4552 CAS.
- X. Gui, J. Wei, K. Wang, A. Cao, H. Zhu, Y. Jia, Q. Shu and D. Wu, Adv. Mater., 2010, 22, 617 CrossRef CAS PubMed.
- Z.-Y. Wu, C. Li, H.-W. Liang, J.-F. Chen and S.-H. Yu, Angew. Chem., 2013, 125, 2997 CrossRef.
- G. Jiang, R. Hu, X. Wang, X. Xi, R. Wang, Z. Wei, X. Li and B. Tang, J. Text. Inst., 2013, 104, 790 CrossRef CAS.
- G. Hayase, K. Kanamori, M. Fukuchi, H. Kaji and K. Nakanishi, Angew. Chem., Int. Ed, 2013, 52, 1986 CrossRef CAS PubMed.
- A. Li, H.-X. Sun, D.-Z. Tan, W.-J. Fan, S.-H. Wen, X.-J. Qing, G.-X. Li, S.-Y. Li and W.-Q. Deng, Energy Environ. Sci., 2011, 4, 2062 CAS.
- N. Ji, H. Chen, M. Yu, R. Qu and C. Wang, Polym. Adv. Technol., 2011, 22, 1898 CrossRef CAS.
- D. Ceylan, S. Dogu, B. Karacik, S. D. Yakan, O. S. Okay and O. Okay, Environ. Sci. Technol., 2009, 43, 3846 CrossRef CAS.
- J. T. Korhonen, M. Kettunen, R. H. A. Ras and O. Ikkala, ACS Appl. Mater. Interfaces, 2011, 3, 1813 CAS.
- R. K. Farag and S. M. El-Saeed, J. Appl. Polym. Sci., 2008, 109, 3704 CrossRef CAS.
- X. Yuan and T. C. M. Chung, Energy Fuels, 2012, 26, 4896 CrossRef CAS.
- H.-P. Cong, X.-C. Ren, P. Wang and S.-H. Yu, ACS Nano, 2012, 6, 2693 CrossRef CAS PubMed.
- Z. Niu, J. Chen, H. H. Hng, J. Ma and X. Chen, Adv. Mater., 2012, 24, 4144 CrossRef CAS PubMed.
- J. Wang, Z. Shi, J. Fan, Y. Ge, J. Yin and G. Hu, J. Mater. Chem., 2012, 22, 22459 RSC.
- Y. Zhao, C. Hu, Y. Hu, H. Cheng, G. Shi and L. Qu, Angew. Chem., 2012, 124, 11533 CrossRef.
- Q. Zhu, Q. Pan and F. Liu, J. Phys. Chem. C, 2011, 115, 17464 CAS.
- H. Bi, X. Xie, K. Yin, Y. Zhou, S. Wan, L. He, F. Xu, F. Banhart, L. Sun and R. S. Ruoff, Adv. Funct. Mater., 2012, 22, 4421 CrossRef CAS.
Footnote |
† Electronic supplementary information (ESI) available. See DOI: 10.1039/c4ra15115a |
|
This journal is © The Royal Society of Chemistry 2015 |
Click here to see how this site uses Cookies. View our privacy policy here.