DOI:
10.1039/C5RA00018A
(Paper)
RSC Adv., 2015,
5, 25518-25524
Curcumin entrapped folic acid conjugated PLGA–PEG nanoparticles exhibit enhanced anticancer activity by site specific delivery
Received
1st January 2015
, Accepted 26th February 2015
First published on 27th February 2015
Abstract
Herein we report curcumin entrapped nanoparticles of PLGA–PEG copolymer which were conjugated with folic acid (PPF copolymer) for site specific targeting since many cancer cells exhibit external folic acid binding receptors. The PPF copolymer was synthesised by two steps using DCC/NHS chemistry and characterized by FT-IR and 1H-NMR techniques. The curcumin was encapsulated in PPF by single emulsion-solvent evaporation method. The nanoparticles were characterised by TEM and DLS for their size and morphology. The thermal properties were assessed by DSC data. In vitro release kinetics measurements of the drug from the nanoparticles showed a controlled release pattern over a period of time. The MTT assay depicted a high amount of cytotoxicity of PPF nanocurcumin in HeLa cells. Cellular uptake studies demonstrated the efficacy of surface engineering with folate over free nanoparticles. Furthermore, the biological evaluations, for example AO/EB staining, DAPI staining, and clonogenic assay of the nanoparticles confirm the efficacy and mechanism by which the cytotoxicity was induced.
Introduction
Although chemotherapy is a conventional treatment for cancers in clinical practice, its efficacy is usually restricted due to an insufficient concentration of the drug at the malignant tissue and its undesired side effects. In addition to this, the high interstitial pressure of the tumor microenvironment acts as a hurdle to the antineoplastic agents reaching the malignant tissues.1 These issues make researchers think about an alternative source of targeted drug delivery system to tumor (targeted delivery) by suitably encapsulating and conjugating the drug in a suitable polymer system and within the nano regime for easy delivery to tumor cells.
Curcumin, a naturally occurring polyphenol obtained from the rhizome of turmeric (Curcuma longa) is found to have a wide range of medicinal properties.2 It is capable of regulating a number of signalling pathways, along with acting as an antiproliferative, antiangiogenic antioxidant agent.3–5 These anti tumor property of curcumin results due to the down regulation of various transcription factors like NF-κB,6 AP-1,7 and beta-catenin.8 The therapeutic usage of curcumin was limited due to the shortcomings such as poor aqueous solubility at acidic and physiological pH, hydrolysis at basic pH, existence of interchanging tautomeric forms by virtue of its chemical structure, low bioavailability, rapid metabolism and elimination.9–11 Therefore studies are being undertaken to encapsulate curcumin into a suitable biocompatible polymer. We had already reported a nanoformulation of curcumin where curcumin is entrapped in PLGA nanoparticle to form nanocurcumin.12 So a slight modification in it to target towards the cancer cells will help to improve its bioavailability.
Receptor targeted drug delivery is now one of the most commonly used method of delivering drug to the specific site.13 This is one of the most commonly used method for delivering drugs to cancer sites. Several receptors are expressed on the surface of cancer cells. Receptor targeting helps in the easy uptake of nanoparticles via endocytosis. Among several receptors, folic acid is commonly expressed by many cancer cells. Folic acid receptor is a 38 kDa glycosylphosphatidylinositol anchored cell surface receptor which has been known to be over-expressed in several human malignancies including lung, head and neck, breast and ovarian cancer.14,15 Folic acid as a receptor targeting ligand offers many potential advantages over other macromolecules such as monoclonal antibodies. These include: small size of the ligand, convenient availability, nonimmunogenicity, facile conjugation chemistry, and high binding affinity, good stability in storage and circulation and lack of normal tissue receptor expression. This leads to high tumor tissue specificity.16–18
So here we tried to make curcumin entrapped nanoparticles with PPF (PPF) copolymer which is conjugated with folic acid for site specific targeting since many cancer cells externally exhibit folic acid binding receptors. So when drug loaded PPF nanoparticles are introduced into the body, they will be targeted specifically to cancer cells and deliver them to cells via folate receptor-mediated endocytosis (clathrin mediated) and produce enhanced cytotoxic effect.
Experimental section
Materials
Poly lactic-co-glycolic acid (PLGA) 50
:
50 (lactic/glycolic ratio) having MW 24
000 Da to 38
000 Da, poly ethylene glycol (PEG) bisamine MW 3000 Da, N-hydroxy succinimide (NHS) MW 115.09, dicyclohexylcarbodiimide (DCC) MW 206.3, pyridine, folic acid, dichloro methane (DCM), dimethyl sulfoxide (DMSO), acetone, curcumin, acridine orange and ethidium bromide were obtained from Sigma (Steinheim, Germany). Polyvinyl alcohol (PVA) 87% hydrolysed was procured from SD fine (Mumbai, India). DAPI was purchased from Santa Cruz Biotechnology. Dulbecco's Modified Eagle Medium (DMEM) was purchased from Life Technologies (Grand Island, NY, USA). 3-(4,5-Dimethylthiazol-2-yl)-2,5-diphenyltetrazolium bromide (MTT), propidium iodide, were purchased from Calbiochem (San Diego, CA, USA). The solvents used for the study were of analytical grade.
Preparation of PPF (PPF) copolymer
Folic acid (FA, 646 mg, 1 mmol) was reacted with DCC/NHS in mild base triethylamine (TEA) to obtain an activated FA (NHS-ester of FA). This reaction was carried out in dry DMSO, inert atmosphere with overnight stirring. To the activated FA; PEG bisamine was added to get folate conjugated PEG bisamine. This compound was isolated and purified by dialysis using MEMBRA-CEL® dialysis tubing (SERVA Diameter-22 nm MWCO 3500 Da) ensuring the removal of traces of unreacted reactants and catalysts. Similarly NHS ester of PLGA was also prepared by using DCC/NHS chemistry in dry DMSO. PLGA (MW = 24
000 Da to 38
000 Da; 2.147 g) was dissolved in dry DMSO and reacted with DCC/NHS to obtain PLGA–NHS ester (activated PLGA). This product was further reacted with folate conjugated PEG bisamine to get folic acid conjugated PLGA–PEG diblock copolymer (PPF). The product was purified by dialysis as mentioned above and lyophillised (Scheme 1).17
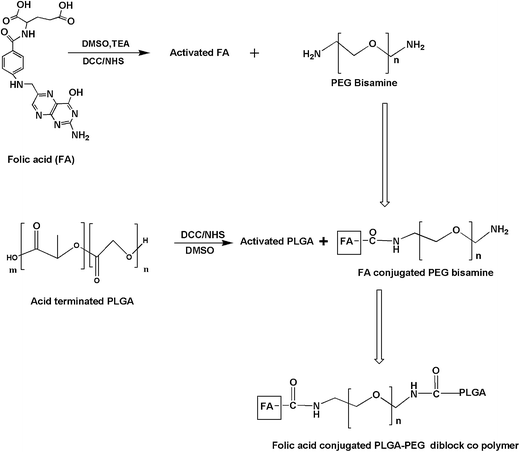 |
| Scheme 1 Synthesis of folic acid conjugated PPF (PPF) polymer nanoparticles. | |
Characterization of prepared PPF (PPF) copolymer
Proton nuclear magnetic resonance spectra (1H-NMR) were taken using Bruker 500 MHz NMR spectrometer using DMSO as solvent to confirm the structure and formation of co polymer. Fourier Transform Infrared (FT-IR) spectra were also analysed by FT-IR spectrometer (Perkin-Elmer, USA, Spectrum 65) using potassium bromide (KBr) pellets to confirm the formation of PLGA–PEG co polymer with folic acid conjugation with it.
Differential Scanning Calorimetry (DSC) analysis was done to monitor the thermal response of co polymer matrix. DSC thermo grams were obtained using an automatic thermal analyzer system (Pyres 6 DSC, Perkin-Elmer, USA). Samples were crimped in standard aluminium pan and heated from 20 to 250 °C at a heating rate of 10 °C min−1 under constant purging of N2 at 10 mL min−1.
Encapsulation of curcumin in PPF copolymeric nanoparticles
Curcumin loaded PPF co polymeric nanoparticles were prepared by solvent evaporation technique [single emulsion (o/w)]. From the prepared co polymer, about 150 mg was taken and dissolved in DCM. To that about 15 mg of curcumin in acetone was added and vortexed well for proper mixing and this act as organic phase. That mixture is then emulsified by adding 100 mL of (1.5%) PVA followed by sonication for about 2–3 minutes and subjected to gentle agitation at room temperature for 12 h to remove the organic phase. The sample solutions were then washed with water 2–3 times by centrifugation and lyophilised.
Characterization of prepared curcumin entrapped PPF nanoparticles
Morphological analysis of prepared co polymer PPF was performed using a Transmission Electron Microscope (TEM, JEOL 1011, and Japan). The nanoparticle suspension in Milli-Q Water was dropped on to formvar-coated copper grid and images were taken after the complete drying.
Further the amount of curcumin loading content was assessed as follows. Weighed amount of curcumin loaded nanoparticles were dissolved in dichloromethane
:
methanol (10
:
1) followed by vortexing to get a clear solution. The amount of curcumin in PPF nanoparticles was then calculated using a standard curve obtained by measuring the UV absorbance (Perkin-Elmer, USA) of curcumin at a wavelength of 430 nm. The drug encapsulation efficiency was then calculated as the ratio of curcumin in nanoparticles to the initial amount of curcumin used in formulation. OD of supernatant after water washing by centrifugation of curcumin nanoparticles were also taken for finding the encapsulation efficiency.
Drug release studies
The aim of this study was to analyze the release of curcumin from PPF nanoparticles. 1 mg mL−1 of lyophilized polymeric nanoparticles with curcumin were dispersed in phosphate buffer solution (PBS) of physiological pH (7.4) and incubated at 37 °C under constant stirring. A known volume of the solution was withdrawn at specific intervals from the medium and analyzed for curcumin using UV at 430 nm. The percentage of curcumin released was also calculated using a standard curve.
Cellular uptake studies
Confocal microscopy was used to study the cellular uptake of different formulations of curcumin in HeLa cells. HeLa cells were grown on cover slips placed in 24 well plates. On attaining 80% confluency, the cells were treated with curcumin dissolved in DMSO (25 μM) and, PLGA nanocurcumin (25 μM), PPF nanocurcumin (25 μM) or their corresponding blank polymers suspended in aqueous medium. The cells were then incubated for 2 h, washed with PBS and fixed using PFA. The nuclei were stained using propidium iodide, mounted using DPX and were analyzed for intracellular fluorescence of curcumin using confocal laser scanning microscope in the FITC channel (488 nm).
MTT assay
Cytotoxicity of curcumin dissolved in DMSO was compared to PLGA nanocurcumin and folic acid-conjugated PLGA nanocurcumin dissolved in aqueous medium, in the cervical cancer cell line, HeLa using MTT assay.19 Cells were seeded (3.0 × 103 cells per well) in a 96-well culture plate and were treated with different concentrations of curcumin dissolved in DMSO (5–50 μM) and PLGA nanocurcumin (5–50 μM), PPF nanocurcumin (5–50 μM) or their corresponding blank polymers suspended in aqueous medium for 72 h and then 20 μL MTT (5 mg mL−1) was added in 80 μL culture medium (DMEM) to each well. The cells were lysed after the incubation for 2 h at 37 °C. The measurement of optical density was carried out at 570 nm using a microplate reader (Bio-Rad Laboratories, Hercules, CA). The relative cell viabilities in percentage were calculated as mentioned below and plotted.
Relative cell viability = (A570 of treated samples/A570 of untreated samples) × 100 (A570 = absorbance at 570 nm) |
Acridine orange (AO)/ethidium bromide (EB) staining
In order to analyze the efficacy of different curcumin formulations in inducing apoptosis acridine orange/ethidium bromide (AO/EB) double staining was used.20 Briefly, 5 × 103 cells per well were seeded in a 96-well plate and treated with curcumin dissolved in DMSO (25 μM) and PLGA nanocurcumin (25 μM), PPF nanocurcumin (25 μM) or their corresponding blank polymers suspended in aqueous medium for 24 h. The cells were then washed with 1× PBS, and were stained with acridine orange (100 μg mL−1) and ethidium bromide (EtBr) (100 μg mL−1) solutions for 2 min. After washing the cells using 1× PBS, they were viewed under an inverted fluorescent microscope (Nikon Eclipse, TE-300) and were photographed.
DAPI staining
DAPI staining was used to analyze the apoptotic efficacy of various curcumin formulations. HeLa cells were grown on cover slips by placing them in 24 well plate and were treated with curcumin dissolved in DMSO (25 μM) and PLGA nanocurcumin (25 μM), PPF nanocurcumin (25 μM) or their corresponding blank polymers suspended in aqueous medium for 24 h. The cells were then washed with 1× PBS and were fixed using a mixture of methanol–EDTA. The nuclei of the cells were stained with 1 μg mL−1 of DAPI and were mounted. The cells were then visualised using a fluorescent microscope and the images were taken.
Clonogenic assay
Clonogenic assay was performed to compare the anti clonogenic potential of different formulations of curcumin. 103 HeLa cells were seeded in 24 well plate and treated curcumin dissolved in DMSO (25 μM) and PLGA nanocurcumin (25 μM), PPF nanocurcumin (25 μM) or their corresponding blank polymers suspended in aqueous medium for 72 h. The media was replaced with fresh media and incubated for 1 week. The developed clones were fixed with glutaraldehyde and stained using crystal violet.
Statistics
All the measurements for different experiment were done in triplicates. The results were expressed as arithmetic mean ± standard error of the mean.
Results
Synthesis and characterisation of PPF copolymer
The synthesis of PPF copolymer was achieved by facile DCC/NHS strategy in DMSO with good yield. The dialysis and lyophilisation produced the copolymer in good yield (64.23 ± 2.56%). The prepared PPF copolymer was showing good solubility in DCM. Further the synthesis was confirmed by different characterisation techniques like FT-IR, NMR etc.
To confirm the formation of PPF co polymer FTIR was done (Fig. 1A). Presence of a broad at 3435 cm−1 depicts the –NH2 stretching band. Peak at 2928 cm−1 indicates asymmetric stretching of aliphatic –C–H bond in PLGA or PEG and peak at 2851 cm−1 shows the aromatic –C–H stretching of folate ligand. The presence of amide I band (1626 cm−1) and amide II band (1574 cm−1) was also noted from the spectra which confirms the conjugation of folate. The peak at 1709 cm−1 demonstrated the existence of free carboxylic group of the folate. In addition to this other peaks like 1465 cm−1 (–C
C– aromatic stretching of phenyl ring) and 1419 cm−1 (OH deformation of phenyl skeleton) confirms the presence of folic acid in PPF copolymer.21 The ether –C–O band of PEG was observed in 1088 cm−1.
 |
| Fig. 1 (A) FTIR spectra of prepared PPF co polymer. (B) 1H NMR spectra showing folate conjugation. B(i) showing NMR spectra of PLGA–PEG bisamine while B(ii) shows the spectra of PPF polymer with highlighted portion showing folate conjugation. | |
1H NMR was also done to confirm the structure of the prepared co polymer (Fig. 1B). Fig. 1B(i) shows the NMR spectra of PLGA–PEG bis amine while B(ii) shows the spectra of PPF. The chemical shift values 1–2 δ (Peak a) in both spectra is due to methyl and methylenic protons of PLGA moiety while 3–4 δ (Peak b) are due to methylenic protons of PEG moiety. The highlighted portion 6.8–7.6 δ (Peak c) in Fig. 1B(ii) shows the presence of aromatic protons which confirms the folic acid conjugation in the polymer.
Characterization of PPF copolymeric nanoparticles with curcumin
Curcumin loaded PPF co polymeric nanoparticles were successfully synthesized using solvent evaporation method. The entrapment efficiency of curcumin in PPF nanoparticle was calculated and was found to be 52.2 ± 0.25%. The nanoparticles demonstrated facile dispersibility in water which is an essential property for effective delivery. The morphology and the size of the particles were studied using Transmission Electron Microscopy which shows the particle size range between 100–200 nm (Fig. 2a).
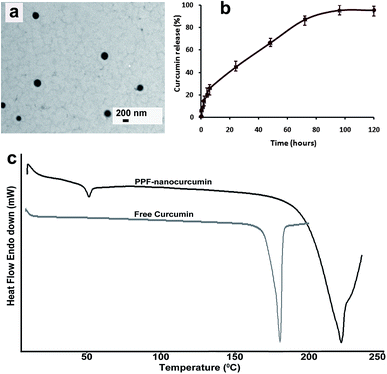 |
| Fig. 2 (a) TEM image showing spherical morphology of curcumin loaded PPF nanoparticles. (b) Release kinetics of curcumin from PPF co polymeric nanoparticles. (c) DSC image of synthesized curcumin entrapped PPF copolymeric nanoparticles and free curcumin. | |
To assess the thermal behavior of prepared folate conjugated PLGA–PEG nanoparticles, DSC was done as shown in Fig. 2c. Exothermic peak at 220 °C may indicate decomposition of folate conjugated PLGA–PEG nanoparticles. Free folic acid usually shows degradation after 250 °C. So this slight shift in the degradation may be because of the conjugation of folic acid with PLGA–PEG entity. Further the distribution of curcumin inside nanoparticles was compared to free curcumin with the help of DSC curve. The disappearance of melting point curve in the PPF-nanocurcumin establishes as the uniform amorphous distribution of the drug inside the nanoparticles.
The release kinetics of curcumin from PPF copolymeric nanoparticles was being studied and it showed 40% of release within 24 h and about 95% of release occurs within five days. But the whole release kinetic profile was slow and sustained pattern (Fig. 2b).
Biological evaluation of PPF-nanocurcumin
Majority of malignant cells are believed to be over-expressing folate receptors. Using confocal microscopy, we analyzed whether conjugation of folate to PLGA nanocurcumin improves the cell adhesion of these particles which in turn will result in better drug delivery. The results illustrate that, folic acid conjugation to PLGA nanocurcumin improve the internalization of curcumin entrapped in them compared to free curcumin and PLGA nanocurcumin (Fig. 3).
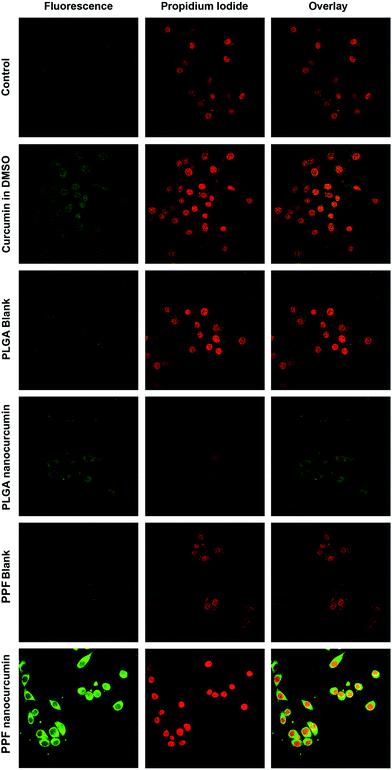 |
| Fig. 3 Cellular uptake studies of PPF nanocurcumin. The nucleus was stained with propidium iodide (red). Curcumin shows green fluorescence. | |
The cytotoxic efficacy of different formulations of curcumin in HeLa cells were assessed by MTT assay. Folic acid-conjugation of PLGA nanocurcumin has shown to increase the cytotoxic effect of curcumin in HeLa cells compared to the free drug dissolved in DMSO as well as PLGA nanocurcumin suspended in aqueous medium (Fig. 4).
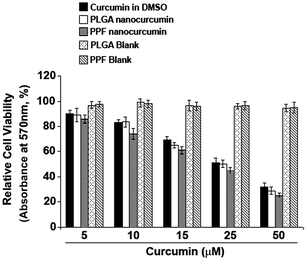 |
| Fig. 4 Cytotoxicity studies (MTT assay) of PPF nanocurcumin. | |
Acridine orange/ethidium bromide staining was used to analyze the apoptotic ability of various formulations of curcumin. In the wells treated with folic acid-conjugated PLGA nanocurcumin, most of the cells were in late apoptotic stage as evidenced by the increased nuclear condensation and more number of red stained cells in these wells. This clearly indicates that folic acid-conjugation to PPF nanocurcumin enhances the ability of curcumin in inducing apoptosis in HeLa cells (Fig. 5A). To confirm this observation, we have performed DAPI staining in HeLa cells treated with the various formulations and compared to that of untreated controls. The nuclei of the cells in apoptotic phase will contain fragmented chromatin or condensed chromatin and will exhibit bright fluorescence compared to normal nuclei. From (Fig. 5B), it is very clear that folic acid conjugation significantly improves the efficacy of PPF nanocurcumin in inducing nuclear condensation of HeLa cells indicative of apoptosis.
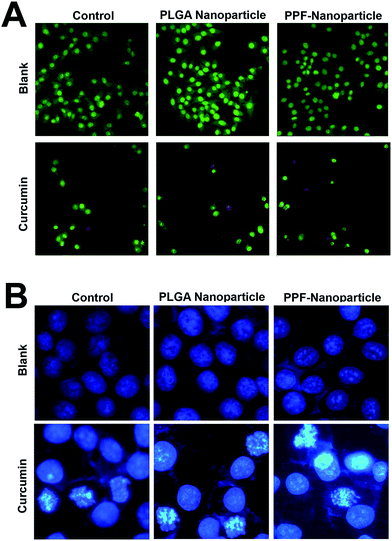 |
| Fig. 5 (A) Acridine orange/ethidium bromide (AO/EB) staining – orange to red colour indicates late apoptotic stage of the cells. (B) DAPI staining of PPF-nanocurcumin. Curcumin (second row) shows condensed chromatin (apoptotic condition) and will exhibit bright fluorescence compared to normal nuclei (blank-first row). | |
Clonogenic assay was conducted to compare the efficacy of various formulations of curcumin in down-regulating the clonogenic potential of HeLa cells. The result indicates that all the three formulations are successful in preventing the formations of HeLa clones almost completely and the effect is comparable (Fig. 6).
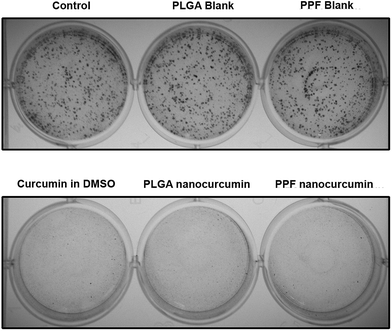 |
| Fig. 6 Clonogenic assay of PPF-nanocurcumin. | |
Discussion
Although curcumin, was reported to be an active antineoplastic agent22 the major hurdle in chemotherapy lies in poor aqueous solubility resulting in low bioavailability which restricts its application. Nanotechnology based approaches will be one of the finest option to overcome this obstacle. The nanoformulation of curcumin were displaying high amount of water dispersive nature which facilitates the elevation in therapeutic efficacy in cancer treatment. So here we synthesized a curcumin entrapped folate conjugated PPF co polymer nanoparticles so that the curcumin entrapped in co polymer nanoparticles help to overcome the solubility problem and bioavailability and the folate conjugation helps in targeting which in turn improves therapeutic efficacy.
The incorporation of PEG and PLGA was proved to be effective in imparting the prolonged circulation time, amphiphilic nature to the nanosystem, reduced systemic clearance which plays a major role in the passive targeting of nanocarrier to tumor by the enhanced permeability and retention (EPR) effects.23,24 Further the conjugation of folate to the nanocarrier was hypothesised to increase the receptor mediated targeting of the malignant cells. The facile synthetic strategy yielded good amount of polymer in a biocompatible solvent; DMSO.25 The physical characterisation followed by consistent amount of curcumin entrapment produced the desired surface engineered nanoparticles. The sustained release pattern observed in kinetics experiment substantiated the use of nanoparticles for tumor targeting.
Further the biological evaluation of the nanomaterial with HeLa cells depicted considerable amount of cellular uptake and cytotoxicity which boost up the efficacy of the surface modified nanosystems. The difference in uptake of PLGA nanocurcumin and PPF nanocurcumin can be attributed to the presence of folic acid on the surface of the nanoparticles which plays a major role in folate receptor mediated endocytosis. These results in higher uptake of PPF nanocurcumin compared to the previous one. The difference in cytotoxicity was observed in all the experimental concentration. The percentage viability of PPF nanocurcumin was considerably lower in all the experimental concentration as shown in Fig. 4. The mechanism of induction of cytotoxicity was assured by the AO/EB and DAPI staining as apoptosis. Apoptosis was hypothesised to be an essential process which maintains the homeostasis of tissues and is thus considered as an ideal way to inhibit cancer cell proliferation.26 Thus the combined physicochemical and biological techniques support the use of the nanosystem for curcumin delivery.
Conclusions
In summary, we have presented the process of surface engineered, tailor made polymeric nanoparticles for the delivery of a poor water soluble molecule, curcumin. The synthesis, characterisation and biological evaluation of the nanoparticles shows that the barriers in the delivery of curcumin can be circumvented successfully. Even though further in vivo validation of the nonmaterial is inevitable, we strongly believe that the nanomaterial can be successfully used in cancer chemotherapy.
Acknowledgements
Authors are thankful to Department of Biotechnology, Government of India, for financial support. Arun Kumar T Thulasidasan and N. Ashwanikumar acknowledge Council of Scientific and Industrial Research, New Delhi, India for providing Senior Research Fellowship.
Notes and references
- R. Cairns, I. Papandreou and N. Denko, Mol. Cancer Res., 2006, 4, 61–70 CrossRef CAS PubMed.
- B. B. Aggarwal and B. Sung, Trends Pharmacol. Sci., 2009, 30, 85–94 CrossRef CAS PubMed.
- A. Goel, A. B. Kunnumakkara and B. B. Aggarwal, Biochem. Pharmacol., 2008, 75, 787–809 CrossRef CAS PubMed.
- S. Shishodia, G. Sethi and B. B. Aggarwal, Ann. N. Y. Acad. Sci., 2005, 1056, 206–217 CrossRef CAS PubMed.
- A. S. Strimpakos and R. A. Sharma, Antioxid. Redox Signaling, 2008, 10, 511–546 CrossRef CAS PubMed.
- A. C. Bharti, N. Donato, S. Singh and B. B. Aggarwal, Blood, 2003, 101, 1053–1062 CrossRef CAS PubMed.
- C. N. Sreekanth, S. V. Bava, E. Sreekumar and R. J. Anto, Oncogene, 2011, 30, 3139–3152 CrossRef CAS PubMed.
- H. P. Chi, R. H. Eun, P. Seyeon, K. Hyun-Kyung and H. Y. Chul, FEBS Lett., 2005, 579, 2965–2971 CrossRef PubMed.
- N. M. Khalil, T. C. F. D. Nascimento, D. M. Casa, L. F. Dalmolin, A. C. d. Mattos, I. Hoss, M. A. Romano and R. M. Mainardes, Colloids Surf., B, 2013, 101, 353–360 CrossRef CAS PubMed.
- P. Anand, A. B. Kunnumakkara, R. A. Newman and B. B. Aggarwal, Mol. Pharm., 2007, 4, 807–818 CrossRef CAS PubMed.
- R. A. Sharma, W. P. Steward and A. J. Gescher, Adv. Exp. Med. Biol., 2007, 595, 453–470 CrossRef.
- K. L. Nair, A. K. T. Thulasidasan, G. Deepa, R. J. Anto and G. S. V. Kumar, Int. J. Pharm., 2012, 425, 44–52 CrossRef CAS PubMed.
- N. Ashwanikumar, N. A. Kumar, S. A. Nair and G. S. V. Kumar, Colloids Surf., B, 2014, 122, 520–528 CrossRef CAS PubMed.
- J. Cummings and C. McArdle, Br. J. Cancer, 1986, 53, 835 CrossRef CAS.
- J. Sudimack and R. J. Lee, Adv. Drug Delivery Rev., 2000, 41, 147–162 CrossRef CAS.
- L. Brannon-Peppas and J. O. Blanchette, Adv. Drug Delivery Rev., 2012, 64, 206–212 CrossRef PubMed.
- H. S. Yoo and T. G. Park, J. Controlled Release, 2004, 100, 247–256 CrossRef CAS PubMed.
- G. Deepa, N. Ashwanikumar, J. J. Pillai and G. S. V. Kumar, Curr. Med. Chem., 2012, 19, 6207–6213 CrossRef CAS.
- R. J. Anto, M. Venkatraman and D. Karunagaran, J. Biol. Chem., 2003, 278, 25490–25498 CrossRef CAS PubMed.
- D. Ribble, N. B. Goldstein, D. A. Norris and Y. G. Shellman, BMC Biotechnol., 2005, 5, 12 CrossRef PubMed.
- H. Huang, Q. Yuan, J. Shah and R. Misra, Adv. Drug Delivery Rev., 2011, 63, 1332–1339 CrossRef CAS PubMed.
- S. V. Bava, V. T. Puliappadamba, A. Deepti, A. Nair, D. Karunagaran and R. J. Anto, J. Biol. Chem., 2005, 280, 6301–6308 CrossRef CAS PubMed.
- J. Guo, X. Gao, L. Su, H. Xia, G. Gu, Z. Pang, X. Jiang, L. Yao, J. Chen and H. Chen, Biomaterials, 2011, 32, 8010–8020 CrossRef CAS PubMed.
- F. Alexis, E. Pridgen, L. K. Molnar and O. C. Farokhzad, Mol. Pharm., 2008, 5, 505–515 CrossRef CAS PubMed.
- M. L. Jones and R. J. Greff, Google Patents, US6699222 B1, 2004.
- G. I. Evan and K. H. Vousden, Nature, 2001, 411, 342–348 CrossRef CAS PubMed.
Footnote |
† Authors contributed equally. |
|
This journal is © The Royal Society of Chemistry 2015 |
Click here to see how this site uses Cookies. View our privacy policy here.