DOI:
10.1039/C5RA00760G
(Paper)
RSC Adv., 2015,
5, 25477-25484
Multifold ring closing metathesis reactions in the formation of resorcin[4]arene cavitands
Received
13th January 2015
, Accepted 2nd March 2015
First published on 3rd March 2015
Abstract
The formation of the resorcin[4]arene cavitands using the ring closing metathesis reaction (RCM) in perallylated resorcin[4]arenes was investigated. The formation of resorcinarene cavitands offers unique molecular platforms for host–guest chemistries, sensor development, metal complexation, as well as new polymers and self-assembled systems, and as potential reaction sites, and novel catalytic platforms. In this manuscript we show that cavitand formation by the RCM reaction depends, to a large extent, on the conformation and the substituents on the upper and lower rim of the perallylated resorcin[4]arenes. The perallylation of the octahydroxy compounds disrupted the intramolecular hydrogen bonds causing a dynamic shift in the conformer equilibrium.
Introduction
Encapsulating molecules in closed surface spherical molecules was proposed by D. J. Cram in 1982.1 Calix- and resorcin[4]arene cavitands have since been used as precursors to carceplexes, carcerands, hermicarcerands and for encapsulating guest molecules and ions, largely because of their rigidity, enforced cavities and synthetic viability. The ability of resorcin[4]arenes to act as a platform for the synthesis of a multitude of supramolecular structures has been of growing interest.2 Consequently, resorcin[4]arenes and their derivatives have been used as metal complexing agents, sensors, receptors, molecular reaction vessels and catalytic chambers.3
The conformation of the resorcin[4]arenes can be rigidified into a crown conformation by bridging the hydroxyl groups of the upper rim to a cavitand structure leading up to a conformationally locked encapsulating platform.4 The covalent linkage of the phenolic groups on the adjacent phenyl rings is generally exploited for synthesis of the rigid ‘bowl’ shaped cavity. The methylenedioxy bridged cavitand, formed by the treatment of the octahydroxy resorcin[4]arene with excess CH2ClBr in the presence of a base, is the most common covalent linkage reported.2 The ethylene-, propylene-, dialkylsilicon-, phosphoryl-, heterophenylene-, phosphonate- and quinoxaline-bridged cavitands have also been reported (Fig. 1).5–8 Clearly the bridging reaction can be used to manipulate the cavity size and hence can be used to alter the properties of the cavitand.9
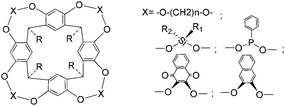 |
| Fig. 1 Bridged-resorcin[4]arenes. | |
Ring-closing metathesis (RCM) has been rapidly established as an efficient methodology for synthesis of medium to large ring systems.10 Remarkable functional group tolerance, high stability, and commercial availability of the catalyst and the operational simplicity have greatly contributed to the popularity of the RCM reaction. Consequently, it has found applicability in the synthesis of novel macrocyclic hosts11 and also in the construction of supramolecular assemblies with specific structures.12 However, the examples of multifold metathesis reactions are still limited for their synthetic challenges.10–12
We reported the synthesis and X-ray crystal structure of the first resorcin[4]arenes cavitand by ring closing metathesis (RCM) reaction.2a The ring closing metathesis reaction on perallylated resorcin[4]arenes, where allyl groups on adjacent phenyl rings serve as acyclic diene precursors for RCM, led to the formation of ethenylenedioxy-bridged resorcin[4]arene cavitands (Scheme 1).2a In this manuscript we report our finding on the multifold RCM reaction with different groups on the upper and lower rim of the resorcin[4]arenes to investigate the formation of the resorcin[4]arene cavitands. Interestingly, the multifold metathesis reaction were dependent on the conformation and the substituents on the upper and lower rim of the resorcin[4]arene.
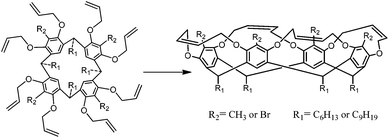 |
| Scheme 1 Ring-closing metathesis of allyloxy resorcin[4]arene to bridged resorcin[4]arene cavitands.2a | |
Results and discussion
Octaallyl resorcin[4]arenes precursors
Condensation of resorcinol, 2-methyl resorcinol or 2-bromo resorcinol with ethanal, 2-ethylbutanal, n-heptanal, n-decanal, benzaldehyde, and 4-bromobenzaldehyde in a refluxing 1
:
1 mixture of ethanol and HCl (37% water) afforded 48–90% yield of the corresponding octahydroxy resorcin[4]arenes, 1–14.2b Resorcin[4]arenes were characterized from their NMR and mass spectral analysis, and whenever possible with the data reported in the literature.4b,8 Although, the resorcin[4]arenes can have four stereoisomers, namely, cis–cis–cis (crown, rccc, C4v), cis–trans–trans (chair, rctt, C2h), cis–cis–trans (diamond, rcct, Cs), and trans–cis–trans (saddle, rtct, D2d) (Fig. 2),4b only two, rccc and rctt, are predominantly formed. Compounds 1, 3, 4, 7, 9, 10, 13 and 14 were isolated as the rccc isomers and compounds 5, 6, 11, and 12 were isolated as the rctt isomers. The resorcin[4]arenes 2 and 8, (resulting upon condensation of 2-ethylbutanal with resorcinol or methyl resorcinol) were observed in the rcct conformation, which has only been reported sparsely in the literature.13 The isomeric forms (rccc, rctt and rcct) were distinguished upon comparison of their 1H-NMR spectra.8 For example, the 1H-NMR spectrum of the rccc isomers showed characteristic single resonance for the resorcin[4]arene aromatic hydrogens while the rctt isomers had two resonances for the resorcin[4]arene aromatic hydrogens. Fig. 3 below shows the representative spectra for compound 1, 5 and 2 which adopt the crown, the chair and the diamond conformations, respectively.
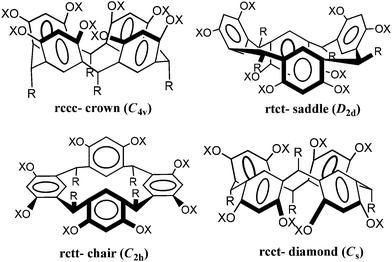 |
| Fig. 2 Stereoisomers of resorcin[4]arenes. | |
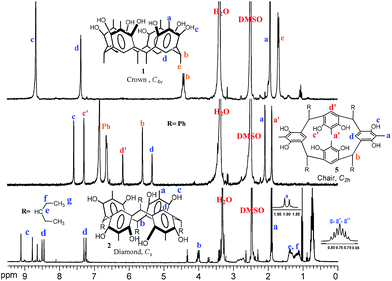 |
| Fig. 3 1H-NMR (250 MHz, DMSO-d6) spectra for conformationally different resorcin[4]arenes 1, 5 and 2. | |
Perallylation of the resorcin[4]arenes
The perallylation of the octahydroxy resorcin[4]arenes was carried out to yield 20–78% of the perallylated products (Scheme 2, Table 1). Importantly, the perallylation in acetone or DMF with allyl bromide using potassium carbonate as a base, under standard reflux conditions did not always give the best yield. The allylation reaction performed in a pressure vessel at increased reaction temperature (120 °C) and pressure gave improved yields. The perallylated compounds 15–28 were characterized from their 1H-, 13C-NMR, and mass spectral analysis. In the 1H-NMR spectra, the allyl group resonances were quantified and the respective integrals confirmed perallylation.
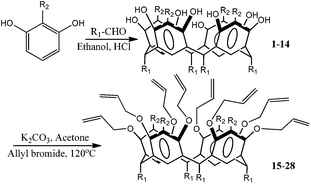 |
| Scheme 2 Perallylation of the resorcin[4]arenes. | |
Table 1 RCM reaction on perallylated resorcin[4]arenes
Resorcinol |
Aldehydes |
Octahydroxy resorcinarenes |
Allyl resorcin-arenesa |
RCM productb |
4-bridge |
2-bridge |
1-bridge |
Acetone/K2CO3 at reflux in a sealed tube. Isolated yield in parenthesis. |
2-Methyl resorcinol |
Ethanal |
1 (67%) |
15 (70%) |
|
|
38 (13%) |
2-Ethylbutanal |
2 (80%) |
16 (40%) |
29 (35%) |
|
|
Heptanal |
3 (88%) |
17 (60%) |
30 (59%) |
|
|
Decanal |
4 (85%) |
18 (55%) |
31 (61%) |
|
|
Benzaldehyde |
5 (88%) |
19 (48%) |
|
|
39 (10%) |
Bromobenzaldehyde |
6 (90%) |
20 (68%) |
|
|
40 (10%) |
Resorcinol |
Ethanal |
7 (48%) |
21 (78%) |
|
|
|
2-Ethylbutanal |
8 (57%) |
22 (15%) |
|
|
|
Heptanal |
9 (78%) |
23 (22%) |
|
34 (32%) |
|
Decanal |
10 (62%) |
24 (20%) |
|
35 (35%) |
|
Benzaldehyde |
11 (56%) |
25 (46%) |
|
36 (22%) |
|
Bromobenzaldehyde |
12 (67%) |
26 (65%) |
|
37 (17%) |
|
2-Bromo resorcinol |
Heptanal |
13 |
27 (68%) |
32 (23%) |
|
|
Decanal |
14 |
28 (35%) |
33 (32%) |
|
|
Cram et al.8a have previously described the conformational dynamics of alkylated resorcin[4]arenes. In hydroxy resorcin[4]arenes the intramolecular hydrogen bonds rigidify the conformer but in perallylated compounds, which lack intramolecular hydrogen bonds, the flexibility of the skeleton increases and an equilibrium exists between the conformers, e.g., for the C4v (crown) and C2v (boat) symmetries, the barrier to interconversion is reportedly 17–19 kcal mol−1,8a increasing with the bulkier alkyl substituents on the lower rim. For example, perallylated resorcin[4]arenes 18 preferred the boat (C2v) conformer in solution (CDCl3); in its 1HNMR spectrum two sets of aromatic resonances at 6.5 and 7.6 ppm were observed (Fig. 4). The single crystal X-ray analysis showed that perallylated resorcin[4]arene 28 exists, in the solid state, in a C2v ‘boat’ conformation.2a
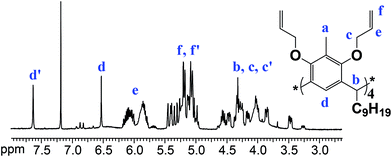 |
| Fig. 4 Partial 1H-NMR (250 MHz, CDCl3) of compound 18. | |
Compound 5, 6, 11, and 12, which exist in the chair conformation (C2h);2b upon perallylation yielded compounds 19, 20, 25 and 26, respectively, which were analyzed from their NMR and mass spectral data and their chair conformation was evident in their 1H NMR spectrum where two resonances for benzylic protons were observed in accordance with C2h symmetry. The X-ray crystal structure of compound 20 confirmed its chair conformation (C2h).2a Likewise, perallylation of the resorcin[4]arenes 2 and 8, resulted in compounds 16 and 22, respectively which maintained the diamond, rcct configuration.
The RCM reaction
The RCM reactions on octaallyl resorcin[4]arenes 15–28 were investigated using 5–10 mol% of generation-I Grubb's catalyst. The reaction was carried out under nitrogen atmosphere in dry dichloromethane as a solvent at room temperature. The reactions were slow and the effect of upper and lower rim resorcin[4]arene substituents was significant on the RCM reaction (Table 1). With progression of the RCM reaction, thin layer chromatography (ethyl acetate and hexane, 1
:
4) showed the appearance of product(s) at lower Rf value compared to the starting perallylated resorcin[4]arene. The product was separated using silica gel column chromatography using an ethyl acetate/hexane gradient system with increasing ethyl acetate in the eluent (6–10%) and isolated products were analyzed by 1H- and 13C-NMR, 2D-NMR spectral data and mass spectrometric measurements.
Formation of the four bridge cavitand
Intramolecular ring closing metathesis in perallylated resorcin[4]arenes 16, 17, 18, 27 and 28 at 25 °C for 96 h using 5 mol% of Grubb's catalyst led to the product cavitands 29–33 in 35–61% yield with all four 2-butylene bridges formed on the upper rim (Scheme 3). Reactions repeated with higher amount of catalyst (up to 8 mol%) did not lead to higher product yield. In a batch addition experiment, an additional amount (5 mol%) of catalyst was added after 48 h, but without higher conversion. The respective four bridge cavitands 29–33 were the only products isolated and the unreacted substrate perallylated resorcin[4]arene 17 was recovered after column chromatography to be reused in the reaction to increase overall yield of the cavitand.
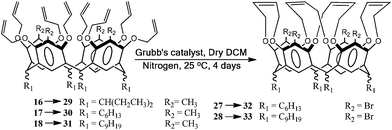 |
| Scheme 3 Ring closing metathesis for formation of four bridge cavitands. | |
The structure of the cavitand 29–33 was established from detailed analysis of the 1H- and 13C-NMR data. In the 1H NMR spectrum, the resonances for the terminal vinylic protons (CH
CH2) observed in the 1H NMR of the starting perallylated resorcin[4]arene were replaced by the resonance of the bridge alkene protons (HC
CH) (Fig. 5). In the 13C NMR spectrum, the resonances that appeared at 116.0–118.0 ppm for terminal vinylic carbons (CH
CH2) disappeared while new resonances for the formed bridge (HC
CH) were at 125.0–130.0 ppm after ring-closing metathesis. The presence of one resonance for a set four carbons in the 13C-NMR spectrum suggested a C4v symmetric crown conformation for the compounds 30–33. The mass spectrum confirmed the molecular integrity of the resulting cavitands. In compound 29, the unsymmetrical conformation was evident in the NMR and the disappearance of the vinylic CH2 in the DEPT NMR and the molecular weight confirmed the formation of the four bridged cavitand.
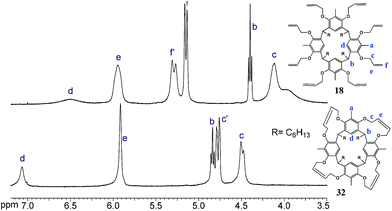 |
| Fig. 5 1H-NMR (250 MHz, CDCl3) of compounds 32 and 18. | |
The compound 30 could be crystallized from a methanol and ethyl acetate (70
:
30) solvent system. The crystal structure showed the formation of all four bridges in the upper rim and confirmed the tetrameric rigid structure with crown conformation (C4v symmetry) and the cis-geometry of the double bond.2a The crystal structure of compound 30 also confirmed that RCM reaction involved two allyl groups on the adjacent phenyl rings rather than those on the same phenyl ring.2a Molecular weight of the compound 30 was determined to be 1032 g mol−1 [observed m/z = 1033 (M + H+)] from it APCI MS analysis.
Formation of the two bridge compounds
RCM reaction catalyzed by the Grubb's generation I catalyst in compounds 23–26 led to partially bridged products 34–37, respectively, with 17–35% yield (Scheme 4). Even at higher concentration of the Grubb's catalyst (8–10 mol%), formation of only two bridges was observed after 4 days of reaction monitoring. Unreacted starting compound could be recovered during column chromatographic separation/isolation and reused to increase the overall yield.
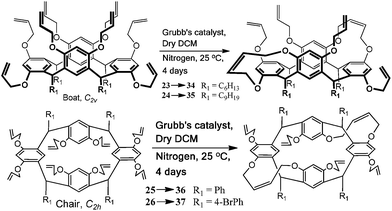 |
| Scheme 4 Ring closing metathesis for formation of A,C-di-bridged cavitands. | |
Compounds 23 and 24, which lack a substituent on the upper rim led to partially bridged products 34 and 35, respectively with 32–35% yield. The structures of compounds 34 and 35 were established by 13C- and DEPT NMR data. The molecular weight of compound 34 was determined to be 1088 g mol−1 [observed m/z = 1089 (M + H+)] and that of compound 35 was determined to be 1256 g mol−1 [observed m/z = 1257 (M + H+)] from APCI MS analysis.
In 1H-NMR spectra of 35 (Fig. 6), two different alkene proton resonances originating from unreacted allyl groups (5.1–5.4 ppm for the
CH2; 6.1 ppm for the
CH) and the alkene bridge (5.9 ppm for HC
CH) were observed. The appearance of only two sets of resonances for the alkenyl protons (unreacted and in the bridge) suggested a two-fold symmetry in compounds 34 and 35. Also, the appearance of only one sharp resonance for the upper rim Ar–H (Ha; 6.4 ppm) confirmed its chemical shift equivalence and the proposed structure as 35. Similar observations could also be made in the 13C NMR data of compound 35, in which the two sets of resonances were observed for the alkenyl carbons (115.0 ppm for the
CH2; 133.0 ppm for the
CH from unreacted allyl group; 127.0 ppm C
C in the bridge) further establishing a two-fold symmetry in the compounds. Thus it was concluded that the compounds 34 and 35 were A,C-di-bridged, i.e., two bridges formed opposite to each other on the upper rim side. Interestingly, Cram et al.5c reported that bridging reaction of an octa hydroxyl resorcinol, prepared from condensation of resorcinol and hexanal, using CH2ClBr–K2CO3–(CH3)2SO, led to the formation of the A,B-di-bridged tetraol along with other mono-, tri- and tetra-bridged compounds but no A,C-di-bridged compound was reported. The A,B-di-bridged, as expected and unlike 34 and 35, showed multiple resonances for the upper and lower rim Ar–H owing to its asymmetry.5c
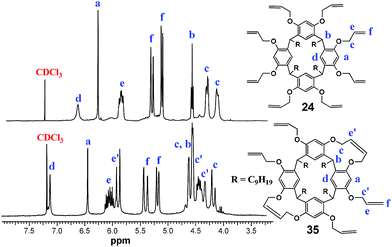 |
| Fig. 6 Regions of the 1H NMRs (250 MHz, CDCl3) of A,C-di-bridged compound 35 and its precursor 24. | |
Perallylated compounds 25 and 26 also led to the formation of two bridges in products 36 and 37 in 17–22% yield. Compounds 25 and 26 prefer the chair conformation (C2h symmetry) in which two adjacent phenyl rings have equatorial orientation and while the other two phenyl rings have axial orientation.2a The chair conformation (C2h symmetry) of the compounds seems to have had significant effect on the ring closing metathesis. Although, the bowl shaped cavitand formation has been accomplished for the compound existing in the chair conformation by the methylenedioxy bridge,4b the steric strain seems to have prevented the formation of a bowl cavitand in the RCM condition investigated in here. The reactions repeated with higher concentration of Grubb's catalyst (10 mol%) only resulted in the two bridge formation even after 4 days (Scheme 4). Unreacted starting compounds 25 and 26 were recovered during column chromatographic separation/isolation. 13C NMR data of 36 and 37 showed that bridged alkene carbons (CH
CH) appeared at 130.0 ppm. Two resonances for the aromatic carbon Ca were observed between 95.0–100.0 ppm and those for Cd were observed between 132.0–133.0 ppm. Unreacted vinylic carbons (–CH
and
CH2) showed resonances at 125.0 ppm and 116.0 ppm respectively. The resonances for allylic carbon (O–CH2–CH
) was observed between 65.0–75.0 ppm. It was concluded that compounds 36 and 37 had two intramolecular 2-butylene bridges but in opposite rim sides of the resorcin[4]arene cavity retaining their chair conformation (C2v symmetry). The molecular weight of compound 36 was determined to be 1056 g mol−1 [observed m/z = 1056 (M+)] and that of compound 37 was determined to be 1368 g mol−1 [observed m/z = 1386 (M + H2O)] from MALDI MS analysis.
Formation of one bridge compound
In allyl compounds 15, 19, and 20 the RCM reaction led to formation of compounds 38, 39, and 40 respectively (Scheme 5) in 10–13% yield. After column chromatography only one compound could be isolated and attempts at increasing the product yield with higher concentration of Grubb's catalyst (10 mol%) and longer reaction times were unsuccessful.
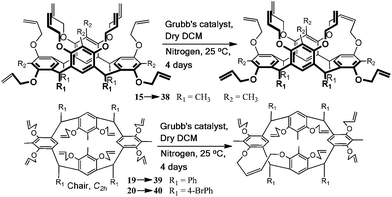 |
| Scheme 5 Ring closing metathesis for formation of mono-bridged cavitands. | |
The 1H-NMR spectra of the mono-bridged compounds 38–41 revealed the molecular structure as being highly unsymmetrical (Fig. 7). We observed four different carbon resonances for the upper rim methyl groups on aromatic ring, and four different resonances for each aromatic Hd proton, two in the shielding zone at 5.6 and 5.8 ppm and two in the deshielding zone at 6.3 and 6.5 ppm. The molecular weight of the compounds also confirmed the formation of the single bridge in compounds 38–41. Molecular weights of the compounds 38 [observed m/z = 893 (M + H+)], 39 [observed m/z = 1163 (M + Na+)]; and 40 [observed m/z = 1480 (M + Na+)] suggested the formation of a single bridge by reaction of the two allyl groups on the adjacent phenyl rings.
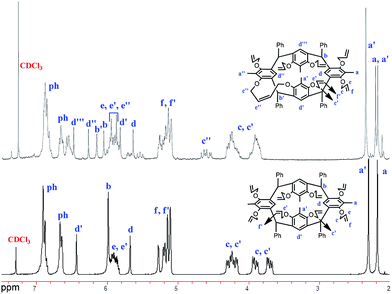 |
| Fig. 7 Regions of the 1H-NMR spectra (250 MHz, CDCl3) of mono bridged compound 39 and its precursor 19. | |
The RCM reaction on 21 and 22 did not led to the isolation of a well-defined product but the TLC chromatography indicated mostly unreacted starting compound and some compound decomposition (streak). The small alkyl subsequent on the lower rim and lack of a substituent on the upper rim in 21 and 22, along with the perallylation, may reduce the energy barrier to the conformer equilibrium to an extent that the RCM reaction was not possible.
Conclusions
In this manuscript, we have investigated the formation of the resorcin[4]arene cavitands using the ring closing metathesis reaction (RCM) in perallylated resorcin[4]arenes. The cavitand formation by the RCM reaction is shown to depend, to a large extent, on the conformation and the upper and the lower rim substituents on the resorcin[4]arenes. It was observed that allylation of the octahydroxy compounds disrupted the intramolecular hydrogen bonds between adjacent phenolic hydroxyl and caused a dynamic shift in equilibrium between the crown and boat conformers. The crown conformation with larger alkyl substituents (pentyl, hexyl or nonyl) on the lower rim and a methyl or bromo substituent on the upper rim were a prerequisite for formation of the tetra bridged bowl shaped cavitand, e.g. 29–33. In compounds with hydrogen (no CH3 or Br) on the upper rim but with longer alkyl chains on the lower rim (hexyl and nonyl) it was observed that only di-bridged compounds could be formed, e.g., 34 and 35. For resorcin[4]arenes with lower rim aromatic substituents, which exist in the chair (C2h) conformation, the crown conformers are energetically much less favored because of the repulsion between equatorial aryl ring and the allyloxy groups. The RCM reaction in the perallylated chair-resorcin[4]arenes with hydrogen on the upper rim resulted in the A,C-di-bridged resorcin[4]arenes 36 and 37, while a CH3 on the upper rim only resulted in a mono-bridged resorcin[4]arenes, i.e., 39 and 41. Though tetra-bridged cavitand formation has been reported in chair-resorcin[4]arenes, most probably a result of ring-inversion to the corresponding cone conformation which is stabilized by the intramolecular hydrogen bonds,4b but such ring inversion in perallylated chair-resorcin[4]arenes is not possible due the size of the allyoxy group. For perallylated compounds with CH3 group on the lower rim the dynamic equilibrium between the conformers appear to quite rapid in the solution and only mono bridged compound (38) could be synthesized when the upper rim had a methyl subsequent; with a hydrogen on the upper rim, i.e., 21 and 22, no well-defined RCM product could be isolated.
Experimental
General information
All solvents and reagents were commercially available. Heptanal was purified by distillation. NMR spectra were recorded on 250 MHz and 400 MHz spectrometers at 398 K. Mass spectra were recorded in APCI mode and MALDI. Compounds 1, 3–7, 9–14, 17–20, 27, 28, 30–33, and 41 have previously been reported in the literature.2a,b,i,4a,5a
Synthesis of octahydroxy resorcin[4]arene (1–14)
Following the literature methods the hydroxy resorcin[4]arenes were synthesized. In a typical procedure, resorcinol, 2-methylresorcinol or 2-bromo resorcinol (8 mmol) was dissolved in anhydrous ethanol (775 ml mol−1) and 37% aqueous HCl (185 ml mol−1). The solution was cooled in an ice bath and the aldehyde (8.1 mmol) was added to above solution slowly over a period of 30 min. Then the mixture was allowed to warm to room temperature. The reaction was then maintained at temperature of 80 °C for nearly 12 h after which the precipitate was filtered through Buchner funnel and washed several times until it turned neutral to pH paper. It was dried and the NMR spectrum was taken and the date was compared with that in reported literature.
Compound (2). 80%; 1H NMR (250 MHz, DMSO-d6) δ 0.77 (m, 24H), 1.22 (m, 16H), 1.35 (m, 4H), 1.93 (s, 12H), 1.95 (s, 6H), 3.99 (m, 4H), 7.27 (s, 2H), 7.33 (s, 2H), 8.48 (s, 2H), 8.54 (s, 2H), 8.82 (s, 4H), 9.14 (s, 4H); 13C NMR δ in ppm 9.9, 10.9, 21.5, 22.0, 56.0, 112.3, 112.6, 123.9, 124.7, 125.1, 129.2, 149.5, 149.9, 150.5. ESI-MS (m/z): M + Na+, found 847.517. C52H72O8Na requires 847.511.
Compound (8). 57%; 1H NMR (250 MHz, DMSO-d6) δ 0.76 (m, 24H), 1.10–1.47 (m, 20H), 3.91–4.05 (m, 4H), 6.09 (s, 2H), 6.18 (s, 2H), 7.34 (s, 2H), 7.44 (s, 2H), 8.85 (s, 2H), 8.93 (s, 2H), 9.10 (s, 2H), 9.25 (s, 2H), 9.59 (s, 2H); DEPT NMR (DMSO-d6) δ in ppm 9.9, 10.8, 21.6, 21.9, 38.2–40.9, 56.2, 103.6–104.3, 129.3, 133.6. ESI-MS (m/z): M + H+, found 769.466. C48H65O8 requires 769.467.
Synthesis of octaallyloxy resorcin[4]arene
Method A. 1.0 g of octahydroxy compound was dissolved in acetone (20 ml mol−1) in a round-bottomed flask. The reaction mixture was cooled to 0 °C in an ice bath and potassium carbonate (30 eq.) was slowly added over a period of half an hour during which the solution changed color to purple. Then the reaction mixture was brought to room temperature and allyl bromide (30 eq.) was added. The reaction mixture was refluxed and monitored using TLC. Upon completion, the reaction mixture was filtered and the filtrate was concentrated in vacuo to yield the crude product. Recrystallization from a mixture of acetone and methanol (50
:
50) resulted in the pure perallylated product.
Method B. 1.0 g of octahydroxy compound was taken into high pressure reaction tube and dissolved in acetone (20 ml mol−1). Then potassium carbonate (30 eq.) was added to the reaction tube; the solution color changed to purple and then allyl bromide (30 eq.) was added and the reaction tube was sealed with a threaded Teflon plug. The high pressure reaction tube was then heated to temperature of 80 °C in an oil bath for 24–48 h. Upon completion, the reaction mixture was filtered and the filtrate was concentrated in vacuo to yield the crude product. Recrystallization from a mixture of acetone and methanol (50
:
50) resulted in the pure perallylated product.
Compound (15). 60%; 1H NMR (250 MHz, CDCl3) δ 1.45 (t, 12H, J = 6.6 Hz), 1.85 (bs, 6H), 2.24 (bs, 6H), 3.41 (bs, 8H), 3.82 (bs, 8H), 4.44 (bs, 4H), 4.46–4.51 (m, 8H), 5.01–5.18 (m, 8H), 5.48 (s, 2H), 6.07 (s, 2H); 13C NMR (CDCl3) δ in ppm 10.5, 20.9, 32.8, 73.2, 90.5, 116.12, 123.6, 133.0, 134.5, 155.0. ESI-MS (m/z): M + Na+, found 943, C60H72O8Na requires 943.
Compound (16). 40%; 1H NMR (250 MHz, CDCl3) δ 0.21 (t, 6H, J = 6.6 Hz), 0.58–0.89 (m, 24H), 1.09–1.74 (m, 14H), 2.13 (s, 6H), 2.20 (s, 6H), 3.62–3.69 (m, 2H), 3.96–4.03 (m, 2H), 4.10–4.33 (m, 6H), 4.38–4.56 (m, 5H), 4.67–4.72 (m, 4H), 4.97–5.55 (m, 18H), 5.85–6.29 (m, 8H), 6.72 (s, 2H), 7.85 (s, 2H). 13C NMR δ in ppm 10.4, 11.2, 11.3, 12.7, 22.3, 23.5, 24.3, 36.5, 37.4, 40.3, 45.1, 48.3, 73.1, 73.5, 116.3, 116.5, 116.6, 116.8, 125.9, 127.4, 125.9, 127.4, 134.4, 134.8, 135.1, 135.2. ESI-MS (m/z): M + H+, found 1145.779. C76H105O8 requires 1145.
Compound (21). 78%; 1H NMR (250 MHz, CDCl3) δ 0.89–1.57 (m, 44H), 2.16 (s, 6H), 2.67 (s, 6H), 3.11–4.86 (m, 20H), 4.89–5.51 (m, 16H), 5.72–6.64 (m, 8H), 6.98 (s, 4H); DEPT NMR (CDCl3) δ in ppm 20.9, 32.8, 73.2, 116.1, 123.7, 134.5.
Compound (22). 15%; 1H NMR (250 MHz, CDCl3) δ 0.77 (m, 24H), 1.12–1.61 (m, 16H), 1.88–2.37 (m, 4H), 4.22–4.54 (m, 19H), 5.06–5.47 (m, 16H), 5.84–6.18 (m, 8H), 6.20–6.39 (m, 5H), 7.01–7.28 (m, 4H); 13C NMR (CDCl3) δ in ppm 9.0–11.0, 44.1, 44.6, 68.8–69.9, 73.9, 98.1–99.7, 101.2–101.4, 115.9–117.8, 127.8, 129.8–130.8, 134.0–135.7. ESI-MS (m/z): M + H+, found 1089. C72H97O8 requires 1089.
Compound (23). 22%; 1H NMR (250 MHz, CDCl3) δ 0.73 (t, 12H, J = 7.2 Hz), 1.12–1.23 (m, 32H), 1.72 (m, 8H), 4.11 (d, 8H, J = 12.0 Hz), 4.28 (d, 8H, J = 12.0 Hz), 4.51 (t, 4H, J = 4.0 Hz), 5.11 (d, 8H, J = 8.0 Hz), 5.29 (d, 8H, J = 16.0 Hz), 5.82 (m, 8H), 6.11 (s, 4H), 6.52 (s, 4H); 13C NMR δ in ppm 14.1, 22.8, 28.3, 29.7, 31.9, 34.7, 35.8, 69.6, 99.3, 116.1, 125.9, 126.8, 134.1, 154.8. ESI-MS (m/z): M + H+, found 1145. C76H105O8 requires 1145.
Compound (24). 20%; 1H NMR (250 MHz, CDCl3) δ 0.89 (t, 12H, J = 7.2 Hz), 1.26–1.31 (m, 56H), 1.85 (m, 8H), 4.13 (d, 8H, J = 12.0 Hz), 4.31 (d, 8H, J = 12.0 Hz), 4.59 (t, 4H, J = 4.0 Hz), 5.13 (d, 8H, J = 8.0 Hz), 5.31 (d, 8H, J = 16.0 Hz), 5.94 (m, 8H), 6.35 (s, 4H), 6.65 (s, 4H); 13C NMR δ in ppm 14.1, 22.9, 28.3, 29.4, 29.8, 30.1, 31.9, 34.7, 35.7, 36.8, 69.6, 99.3, 116.1, 126.3, 126.8, 134.1, 154.7. ESI-MS (m/z): M + Na+, found 1335, C88H128O8Na requires 1335.
Compound (25). 46%; 1H NMR (250 MHz, CDCl3) δ 3.81–4.33 (m, 16H), 4.95–5.20 (m, 16H), 5.53–5.76 (m, 8H), 5.76–5.81 (m, 4H), 5.91 (m, 4H), 6.13–6.17 (d, 2H, J = 9.6 Hz), 6.30 (s, 2H), 6.73–6.77 (m, 16H), 6.89–6.96 (m, 4H); 13C NMR (CDCl3) δ in ppm 69.5, 116.2, 125.3, 125.6, 127.7, 128.9, 133.9, 143.5, 155.6. ESI-MS (m/z): M + H+, found 1113, C76H73O8 requires 1113.
Compound (26). 65%; 1H NMR (250 MHz, CDCl3) δ 3.32–4.62 (m, 16H), 4.99–5.33 (m, 16H), 5.62–5.93 (m, 8H), 6.11–6.23 (m, 4H), 6.29–6.36 (m, 4H), 6.45–6.62 (m, 8H), 6.64–6.73 (m, 4H) 7.10–7.31 (d, 8H, J = 7.0 Hz); DEPT NMR (CDCl3) δ 42.7, 69.0, 69.3, 69.6, 116.5, 130.5, 130.7, 132.9, 133.3, 133.5. ESI-MS (m/z): M + H+, found 1425, C76H69Br4O8 requires 1425.
Ring closing metathesis reaction synthesis of bridged-resorcin[4]arene
To a stirred solution of octaallyloxy resorcin[4]arene (1.0 g, 1.086 mmol) in dry methylene chloride (114 ml) was added Grubb's catalyst (8 mol%, 0.071 gm, 0.087 mmol) in dry methylene chloride (25 ml) at room temperature. The reaction mixture was stirred and monitored by TLC. When no increase in the amount of the product was observed over 8 h, the reaction mixture was concentrated in vacuo to remove solvent. The crude product was column chromatographed over silica gel using ethyl acetate
:
hexane (1
:
9) eluent.
Compound (29). 35%; 1H NMR (400 MHz, CDCl3) δ 0.06 (t, 6H, J = 7.3 Hz), 0.68 (m, 18H), 0.94 (t, 6H, J = 7.5 Hz), 1.25–1.31 (m, 10H), 1.47–1.74 (m, 4H), 2.20 (s, 6H), 2.28 (s, 6H), 4.08–4.16 (m, 4H), 4.37–4.48 (m, 4H), 4.54–4.57 (m, 4H), 4.70–4.86 (m, 6H), 5.24 (t, 2H, J = 8.4 Hz), 5.63–5.73 (m, 2H), 5.92–6.21 (m, 7H), 7.00 (s, 2H), 8.02 (s, 2H); 13C NMR (CDCl3) δ in ppm 8.8, 8.9, 9.2, 10.4, 10.5, 10.6, 10.7, 20.3, 20.8, 21.2, 21.9, 22.2, 36.4, 40.3, 42.2, 43.5, 48.6, 50.5, 67.2, 67.3, 67.9, 72.3, 122.5, 122.6, 122.7, 124.4, 126.6, 129.9, 130.0, 131.0, 131.4, 132.0, 131.1, 132.6, 153.6, 153.8, 154.1, 154.6, 155.0; ESI-MS (m/z): M + H+, found 1033, C68H89O8 requires 1033.
Compound (34). 32%; 1H NMR (250 MHz, CDCl3) δ 0.77 (t, 12H, J = 6.2 Hz), 1.13–1.73 (m, 32H), 1.85 (m, 8H), 4.25 (m, 16H), 4.55 (d, 2H, J = 2.5 Hz), 4.62 (s, 2H), 5.18 (dd, 4H, J = 1.2 Hz, J = 10.5 Hz), 5.41 (dd, 4H, J = 1.5 Hz, J = 17.5 Hz), 5.86 (s, 2H), 5.93 (s, 2H), 6.12 (m, 4H), 6.45 (s, 4H), 7.12 (s, 4H); 13C NMR (CDCl3) δ in ppm 13.0, 21.7, 27.2, 28.7, 30.9, 33.4, 34.5, 63.6, 68.9, 96.8, 98.2, 115.3, 122.3, 125.3, 125.6, 127.0, 128.2, 133.3, 152.0, 153.1; ESI-MS (m/z): M + H+, found 1090, C72H97O8 requires 1089.
Compound (35). 35%; 1H NMR (250 MHz, CDCl3) δ 0.79 (t, 12H, J = 6.2 Hz), 1.12–1.75 (m, 56H), 1.98 (m, 8H), 4.34 (m, 16H), 4.68 (s, 2H), 4.77 (s, 2H), 5.19 (dd, 4H, J = 1.2 Hz, J = 10.2 Hz), 5.43 (dd, 4H, J = 1.5 Hz, J = 17.0 Hz), 5.88 (s, 2H), 5.95 (s, 2H), 6.11 (m, 4H), 6.45 (s, 4H), 7.12 (s, 4H); 13C NMR (CDCl3) δ in ppm 13.0, 21.6, 27.2, 28.3, 28.7, 29.0, 30.9, 33.4, 34.4, 34.5, 63.5, 68.9, 96.8, 98.1, 115.3, 122.7, 125.3, 125.6, 127.0, 128.7, 133.3, 152.0, 153.2; ESI-MS (m/z): M + H+, found 1257, C84H121O8 requires 1257.
Compound (36). 22%; H-NMR (250 MHz, CDCl3) δ in ppm 4.22–4.62 (m, 16H), 5.04–5.20 (m, 12H), 5.72–5.91 (m, 10H), 6.16–6.25 (m, 4H), 6.44 (s, 2H), 6.83–7.03 (m, 22H); C-NMR (CDCl3) δ in ppm 41.8, 63.8, 68.6, 96.2, 101.2, 115.3, 124.4–125.9, 126.8, 127.3, 127.9, 128.1, 132.1, 132.7, 140.9, 141.7, 143.1, 143.9, 151.8, 154.4; DEPT NMR (CDCl3) δ in ppm 41.8, 63.8, 68.6, 96.2, 98.9, 115.3, 124.3, 126.6, 126.8, 127.3, 127.9, 128.1, 132.1, 132.7, 140.9, 141.7, 151.8, 154.4; ESI-MS (m/z): M + H+, found 1057, C72H65O8 requires 1057.
Compound (37). 17%; 1H NMR (400 MHz, CDCl3) δ 4.19–4.71 (m, 16H), 4.87–5.31 (m, 8H), 5.53–5.84 (m, 6H), 5.98–6.24 (m, 4H), 6.39 (s, 2H), 6.52–6.71 (m, 8H), 7.21–7.56 (m, 16H). DEPT NMR (CDCl3) δ in ppm 41.2, 63.8, 68.3, 96.4, 98.1, 115.5, 123.3, 123.5, 127.2, 127.3, 129.7, 130.2, 131.5, 132.4, 140.9, 141.7, 151.8, 154.4; ESI-MS (m/z): M+ + H2O, found 1385 (100%), 1386 (80%), C72H62Br4O9 requires 1386.
Compound (38). 13%; 1H NMR (400 MHz, CDCl3) δ 0.89 (t, 3H, J = 6.6 Hz), 1.26–1.37 (m, 9H), 1.78–2.38 (m, 12), 4.16 (m, 2H), 4.22–4.64 (m, 16H), 4.82 (m, 2H), 4.99–5.34 (m, 12H), 5.62–6.21 (m, 8H); 13C NMR (CDCl3) δ 10.2, 20.2–23.8, 29.8, 32.1, 69.2, 69.9, 71.8, 72.4, 115.9–116.4, 122.0–126.0, 130.1, 132.2, 134.4, 136.2; MALDI m/z 893 (M + H+), C58H69O8 requires 893.
Compound (39). 10%; 1H NMR (250 MHz, CDCl3) δ 2.12 (s, 3H), 2.15 (s, 3H), 2.29 (s, 6H), 3.80–3.96 (m, 6H), 4.10–4.28 (m, 6H), 4.49–4.65 (m, 4H), 5.08–5.26 (m, 12H), 5.62 (s, 1H), 5.81 (s, 1H), 5.83–5.99 (m, 8H), 6.05 (s, 1H), 6.15 (s, 1H), 6.26 (s, 1H), 6.48 (s, 1H), 6.50–6.57 (m, 3H), 6.63–6.68 (m, 4H), 6.80–6.93 (m, 12); 13C NMR (CDCl3) δ in ppm 10.8, 10.9, 24.9, 36.8, 44.7, 44.9, 73.3, 73.4, 73.7, 73.9, 116.4, 116.6, 116.8, 117.0, 123.8, 124.1, 125.3, 125.8, 125.9, 126.3, 127.4, 127.8, 128.3, 129.0, 129.2, 130.1, 131.2, 132.7, 132.8, 133.1, 133.2, 134.1, 134.2, 134.3, 134.4, 141.4, 143.6, 154.3, 154.8, 154.9, 155.0. ESI-MS (m/z): M + Na+, found 1163. C78H76O8Na requires 1163.
Compound (40). 10%; 1H NMR (250 MHz, CDCl3) δ 2.16 (s, 6H), 2.27 (s, 6H), 3.81–3.97 (m, 6H), 4.08–4.34 (m, 6H), 4.40–4.65 (m, 4H), 5.10–5.29 (m, 12H), 5.52 (s, 1H), 5.67 (s, 1H), 5.79 (s, 1H), 5.82–5.96 (m, 6H), 6.00 (s, 1H), 6.09 (s, 1H), 6.12 (s, 1H), 6.37–6.53 (m, 8H), 7.04–7.13 (m, 8H); 13C NMR (CDCl3) δ in ppm 10.9, 11.0, 14.4, 29.9, 43.7, 44.3, 70.8, 73.5, 73.8, 74.5, 110.0, 116.6, 116.8, 117.3, 117.8, 120.0, 120.1, 124.3, 125.8, 126.4, 128.2, 130.6, 130.9, 131.4, 132.1, 132.4, 132.5, 133.8, 134.0, 140.5, 142.9, 143.6, 154.3, 154.8, 155.0, 155.4. ESI-MS (m/z): M + Na+, found 1479 (100%) 1480 (80%). C78H72Br4O8Na requires 1480.
Acknowledgements
Financial support for this work from the Herman Frasch Foundation, and the USF internal awards is greatly appreciated.
Notes and references
-
(a) D. J. Cram, Science, 1983, 219, 1177–1183 CAS;
(b) J. R. Moran, S. Karbach and D. J. Cram, J. Am. Chem. Soc., 1982, 104, 5826 CrossRef CAS.
-
(a) S. N. Parulekar, K. Muppalla, F. R. Fronczek and K. S. Bisht, Chem. Commun., 2007, 4901–4903 RSC;
(b) E. E. Dueno and K. S. Bisht, Tetrahedron, 2004, 60, 10859 CrossRef CAS PubMed;
(c) B. C. Gibb, R. G. Chapman and J. C. Sherman, J. Org. Chem., 1996, 61, 1505–1509 CrossRef CAS;
(d) D. J. Cram and J. M. Cram, Container Molecules and their Guests, ed. J. F. Stoddart, Royal Society of Chemistry, Cambridge, 1994 Search PubMed;
(e) J. C. Sherman, Tetrahedron, 1995, 51, 3395 CrossRef CAS;
(f) Calixarenes, ed. Z. Asfari, V. Bohmer, J. Harrowfield, J. Vicens and M. Saadioui, Kluwer Academic Publishers, Dordrecht, 2001 Search PubMed;
(g) C. D. Gutsche, Calixarenes, Royal Society of Chemistry, Cambridge, 1989 Search PubMed;
(h) P. Timmerman, W. Verboom and D. N. Reinhoudt, Tetrahedron, 1996, 52, 2663–2704 CrossRef CAS;
(i) M. Hedidi, S. Hamdi, T. Mazari, B. Boutemeur, C. Rabia, F. Chemat and M. Hamdi, Tetrahedron, 2006, 62, 5652–5655 CrossRef CAS PubMed.
-
(a) A. G. S. Högberg, J. Am. Chem. Soc., 1980, 102, 6046–6050 CrossRef; A. G. S. Högberg, J. Org. Chem., 1980, 45, 4498–4500 Search PubMed;
(b) E. Roman, C. Peinador, S. Mendoza and A. E. Kaifer, J. Org. Chem., 1999, 64, 2577–2578 CrossRef CAS;
(c) D. J. Cram, S. Karbach, Y. H. Kim, L. Baczynskyj and G. W. Kalleymeyn, J. Am. Chem. Soc., 1985, 107, 2575–2576 CrossRef CAS;
(d) A. R. Mezo and J. C. Sherman, J. Org. Chem., 1998, 63, 6824–6829 CrossRef CAS PubMed;
(e) E. Dueno and K. S. Bisht, Tetrahedron, 2004, 60, 10859–10868 CrossRef CAS PubMed;
(f) R. Wu, T. Al-Azemi and K. S. Bisht, Chem. Commun., 2009, 1822 RSC;
(g) R. Wu, T. Al-Azemi and K. S. Bisht, RSC Adv., 2014, 4, 16864–16870 RSC.
-
(a) L. M. Tunstad, J. A. Tucker, E. Dalcanale, J. Weiser, J. A. Bryant, J. C. Sherman, R. C. Helgeson, C. B. Knobler and D. J. Cram, J. Org. Chem., 1989, 54, 1305–1312 CrossRef CAS;
(b) O. Middel, W. Verboom, R. Hulst, H. Kooijman, A. L. Spek and D. N. Reinhoudt, J. Org. Chem., 1998, 63, 8259–8265 CrossRef CAS;
(c) G. Rumboldt, V. Bohmer, B. Botta and E. F. Paulus, J. Org. Chem., 1998, 63, 9618–9619 CrossRef CAS;
(d) B. W. Purse and J. Rebek, Proc. Natl. Acad. Sci. U.S.A., 2005, 102, 10777–10782 CrossRef CAS PubMed; R. Pinalli, M. Suman and E. Dalcanale, Eur. J. Org. Chem., 2004, 451–462 Search PubMed; D. M. Rudkevich and J. Rebek, Eur. J. Org. Chem., 1999, 9, 1991–2005 Search PubMed.
-
(a) D. J. Cram, S. Karbach, H. Kim, C. B. Knobler, E. F. Maverick, J. L. Ericson and R. C. Halgeson, J. Am. Chem. Soc., 1988, 110, 2229–2237 CrossRef CAS;
(b) P. Timmerman, H. Boerrigter, W. Verboom, G. J. Van Hummel, S. Harkema and D. N. Reinhoudt, J. Inclusion Phenom., 1995, 18, 1 Search PubMed;
(c) D. J. Cram, L. M. Tunstad and C. B. Knobler, J. Org. Chem., 1992, 57, 528 CrossRef CAS;
(d) T. N. Sorrell and F. C. Pigge, J. Org. Chem., 1993, 58, 784–785 CrossRef CAS.
-
(a) D. J. Cram, K. D. Stewart, I. Goldberg and K. N. Trueblood, J. Am. Chem. Soc., 1985, 107, 2574 CrossRef CAS;
(b) J. A. Tucker, C. B. Knobler, K. N. Trueblood and D. J. Cram, J. Am. Chem. Soc., 1989, 111, 3688–3699 CrossRef CAS.
-
(a) W. Xu, J. V. Jadagese and R. J. Puddephatt, J. Am. Chem. Soc., 1993, 115, 6456 CrossRef CAS;
(b) W. Xu, J. J. Vittal and R. J. Puddephatt, J. Am. Chem. Soc., 1995, 117, 8362–8371 CrossRef CAS;
(c) T. Lippmann, H. Wilde, E. Dalcanale, L. Minlla, G. Mann, U. Heyer and S. Spera, J. Org. Chem., 1995, 60, 235 CrossRef CAS.
-
(a) D. J. Cram, H. J. Choi, J. A. Bryant and C. B. Knobler, J. Am. Chem. Soc., 1992, 114, 7748 CrossRef CAS;
(b) M. Vincenti, E. Dalcanale, P. Soncini and G. Guglielmetti, J. Am. Chem. Soc., 1990, 112, 445–447 CrossRef CAS;
(c) J. R. Moran, J. L. Ericson, E. Dalcanale, J. A. Bryant, C. B. Knobler and D. J. Cram, J. Am. Chem. Soc., 1991, 113, 5707–5714 CrossRef CAS;
(d) J. A. Bryant, J. L. Ericson and D. J. Cram, J. Am. Chem. Soc., 1990, 112, 1255 CrossRef CAS;
(e) I. Pochorovski, C. Boudon, J.-P. Gisselbrecht, M.-O. Ebert, W. B. Schweizer and F. Diederich, Angew. Chem., Int. Ed., 2012, 51, 262–266 CrossRef CAS PubMed;
(f) B. Mettra, Y. Bretonniere, J.-C. Mulatier, B. Bibal, B. Tinant, C. Aronica and J.-P. Dutasta, Supramol. Chem., 2013, 25, 672–681 CrossRef CAS.
- F. C. Tucci, D. M. Rudkevich and J. Rebek, J. Org. Chem., 1999, 64, 4555–4559 CrossRef CAS.
-
(a) A. V. Chuchuryukin, H. P. Dijkstra, B. M. J. M. Suijkerbuijk, R. J. M. Gebbink, G. P. M. van Klink, A. M. Mills, A. L. Spek and G. van Koten, Angew. Chem., Int. Ed., 2003, 42, 228–230 CrossRef CAS PubMed;
(b) A. V. Chuchuryukin, P. A. Chase, H. P. Dijkstra, B. M. J. M. Suijkerbuijk, A. M. Mills, A. L. Spek, G. P. M. van Klink and G. van Koten, Adv. Synth. Catal., 2005, 347, 447–462 CrossRef CAS;
(c) K. H. Song, S. O. Kang and J. Ko, Chem.–Eur. J., 2007, 13, 5129–5134 CrossRef CAS PubMed;
(d) E. C. Constable, C. E. Housecroft, M. Neuburger, P. J. Rösel, S. Schaffner and J. A. Zampese, Chem.–Eur. J., 2009, 15, 11746–11757 CrossRef CAS PubMed;
(e) B. Zhu, H. Chen, W. Lin, Y. Ye, J. Wu and S. Li, J. Am. Chem. Soc., 2014, 136, 15126–15129 CrossRef CAS PubMed.
-
(a) J. M. C. A. Kerckhoffs, M. G. J. ten Cate, M. A. Mateos-Timoneda, F. W. B. van Leeuwen, B. Snellink-Ruël, A. L. Spek, H. Kooijman, M. Crego-Calama and D. N. Reinhoudt, J. Am. Chem. Soc., 2005, 127, 12697–12708 CrossRef CAS PubMed;
(b) M. Pitarch, V. Mckee, M. Nieuwenhuyzen and M. A. McKervey, J. Org. Chem., 1998, 63, 946–951 CrossRef CAS;
(c) Y. Rudzevich, Y. D. Cao, V. Rudzevich and V. Böhmer, Chem.–Eur. J., 2008, 14, 3346–3354 CrossRef CAS PubMed;
(d) A. Bogdan, M. Bolte and V. Böhmer, Chem.–Eur. J., 2008, 14, 8514–8520 CrossRef CAS PubMed;
(e) W. J. Zuercher, M. Hashimoo and R. H. Grubbs, J. Am. Chem. Soc., 1996, 118, 634 CrossRef;
(f) M. A. McKervey and M. Pitarch, Chem. Commun., 1996, 1689 RSC;
(g) C. F. Chen, L. G. Lu, Z. Q. Hu, X. X. Peng and Z. T. Huang, Tetrahedron, 2005, 61, 3853–3858 CrossRef CAS PubMed.
- Some recent examples, see:
(a) J. D. Badjic, S. J. Cantrill and J. F. Stoddart, Angew. Chem., Int. Ed., 2004, 43, 3273–3278 CrossRef CAS PubMed;
(b) O. Molokanova, M. O. Vysotsky, Y. D. Cao, I. Thondorf and V. Böhmer, Angew. Chem., Int. Ed., 2006, 45, 8051–8055 CrossRef CAS PubMed;
(c) S. Hiraoka, Y. Yamauchi, R. Arakane and M. Shionoya, J. Am. Chem. Soc., 2009, 131, 11646–11647 CrossRef CAS PubMed;
(d) J. Cao, Z. P. Song, X. Z. Zhu and C. F. Chen, Tetrahedron Lett., 2010, 51, 3112–3115 CrossRef CAS PubMed.
-
(a) F. Weinelt and H. J. Schneider, J. Org. Chem., 1991, 56, 5527 CrossRef CAS;
(b) M. He, R. J. Johnson, J. O. Escobedo, P. A. Beck, K. K. Kim, N. N. St. Luce, C. J. Davis, P. T. Lewis, F. R. Fronczek, B. J. Melancon, A. A. Mrse, W. D. Treleaven and R. M. Strongin, J. Am. Chem. Soc., 2002, 124, 5000–5009 CrossRef CAS PubMed;
(c) P. Sakhaii, L. Verdier, T. Ikegami and C. Griesinger, Helv. Chim. Acta, 2002, 85, 3895 CrossRef CAS.
|
This journal is © The Royal Society of Chemistry 2015 |
Click here to see how this site uses Cookies. View our privacy policy here.