DOI:
10.1039/C5RA01594D
(Review Article)
RSC Adv., 2015,
5, 35116-35137
Synthesis of boron nitride nanotubes via chemical vapour deposition: a comprehensive review
Received
27th January 2015
, Accepted 10th April 2015
First published on 10th April 2015
Abstract
Boron nitride nanotubes (BNNTs) have been synthesized by various methods over the last two decades. Among the various growth techniques, chemical vapor deposition (CVD) is one of the best methods for the synthesis of BNNTs in terms of quality and quantity. It offers relatively easy control of different growth parameters such as growth mechanism, experimental set up, precursor variables, catalyst type and temperature, and hence has become very convenient to grow BNNTs with desired size and morphologies leading to various advanced applications. Here, we present a comprehensive review on BNNT growth by CVD techniques ranging from catalytic to plasma assisted CVD. Moreover, the importance of certain variables for an efficient production of BNNTs, and their effects on the size and morphology of the tubes are also discussed elaborately.
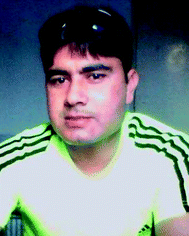 Pervaiz Ahmad | Pervaiz Ahmad obtained his M.Phil. degree in Solid State Physics in 2011 from the Centre of Excellence in Solid State Physic, University of Punjab, PAKISTAN. Currently (Session: 2013–2014) he is pursuing his Ph.D. degree in Physics from the Department of Physics, Faculty of Science, University of Malaya, MALAYSIA. His areas of expertise include: synthesis and characterization of h-BN nanostructures (BNNTs, BNNWs, BNMTs and BNNS etc.), CNTs and iron oxide nanoparticles for their potential application in the field of radiation sensing, biomedical and microelectronic mechanical systems (MEMs), etc. |
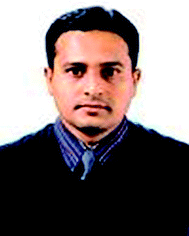 Mayeen Uddin Khandaker | Associate Prof. Dr. Mayeen Uddin Khandaker obtained his Ph.D. from the department of Physics, Kyungpook National University, South Korea. Previously, he served in numerous institutions like the Korea Atomic Energy Research Institute, the International Atomic Energy Agency and American International University-Bangladesh, etc. Currently (2010 – onwards), he is working in the Department of Physics, University of Malaya, Malaysia. His areas of expertise include: synthesis and characterization of nanostructured materials, production of radionuclides for medical and industrial applications, measurement of natural radioactivity in food and environment and development of optical fibre based TLDs for dosimetry applications. |
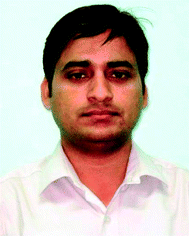 Ziaul Raza Khan | Dr. Ziaul Raza Khan obtained his Ph.D. degree in Physics from Jamia Milia Islamia India. He was a Post-Doctoral Research Fellow at the Low Dimensional Material Research Centre, Department of Physics, University of Malaya, Malaysia. Currently (2015) he is working as an Assistant Professor at the College of Science, University of Hail, Saudi Arabia. His Areas of expertise include: Growth of II–VI semiconductor thin films, Si NWs, SiC thin films and boron nitride nanostructures. |
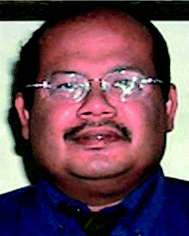 Yusoff Mohd Amin | Prof. Dr. Yusoff Mohd Amin obtained his Ph.D. degree in Physics from Birmingham, UK. Currently (2015), he is working as a Professor at the Department of Physics, University of Malaya, Malaysia. His areas of expertise include: synthesis and characterization of h-BN nanostructures (BNNTs, BNNWs, BNMTs and BNNS, etc.), CNTs, production, detection and applications of radionuclides in nuclear medicine and industry, radiation detection and measurement. |
1 Introduction
BNNTs, cylindrical structures of hexagonal boron nitride (h-BN) having diameter in the range of 1–100 nm and length up to several micrometers,1–3 were theoretically predicted in 1994,4,5 and experimentally discovered in 1995.6 The discovery of BNNTs has opened new ways for making devices with excellent properties. The structure and mechanical properties of BNNTs are similar to carbon nanotubes (CNTs), however CNTs can be conductors or semiconductors depending on the chirality or helicity whereas BNNTs are large band gap semiconductors independent of helicity.7–13 This difference in the electronic structure of these nanostructure materials results in different luminescence emissions. For example BNNTs emit violet or ultraviolet luminescence when excited by electron or photons,14–19 whereas CNTs emit infrared light with wavelength dependent on the chirality of the tube.20–24 Moreover, BNNTs are found to be chemically stable and highly resistant to oxidation.25,26 Therefore it can be effectively utilized in fabrication of different devices that can be used in high temperature environments.26–33
BNNTs have attractive magnetic properties. The theoretical calculations indicated that they (BNNTs) have spontaneous magnetization that can be induced through carbon-doping, which can be achieved by substitution of either B or N atoms.34 BNNTs have intrinsic magnetism that can be induced by their open ends. The magnetic movement thus produced is dependent on the chirality of the tube.35–37 As a result of this magnetism BNNTs produce spin-splitting effect combined with spin-polarization. This property has made it a very important material for different applications in the field of nanoscale spintronics devices e.g. spin polarized emitters etc.38–40 Spontaneous magnetization via fluorination or dopant substitution can also be induced in BNNTs.41 Fluorination of BNNTs can further be used to modify the electronic and transport properties of the BNNTs. The fluorinated BNNTs thus developed have potential applications in nanoelectronics.42
The possible role of BNNTs as insulating protective shield has also been observed in the development of nanocables from semiconducting nanowires.2,43–47 It has been successfully explored for applications in the field of engineering ceramics and polymeric composites.48 It is experimentally observed that the superplasticity of engineering ceramics increases to a great extent with the addition of BNNTs.49 Due to dipolar nature of B–N bond in BNNTs, they result in stronger adsorption of hydrogen. Therefore they are considered a very important material for hydrogen storage applications.50–54 A change in properties in molecular and solid state electronic devices has also been observed due to dipolar nature of the B–N bond. It is further responsible for changing the optical properties of materials in the systems.7,55 Research based on the studies of photoluminescence and cathodoluminescence properties of the materials have shown that BNNTs are effective violet and ultra-violet light emission material. It has been found that the emission peaks normally depend on the samples. On this basis, peaks have been observed at ∼230 nm, ∼279 nm, ∼338 nm and ∼460 nm by different researchers.17,18,56–64
Biocompatibility of any nanomaterial need to be tested before using it within a particular bio-medical application. The biocompatibility tests on BNNTs were first initiated by Ciofani et al.65–68 The obtained results indicated BNNTs to be a very useful material for different biomedical applications such as therapeutic or diagnostic etc. due to their possible non-cytotoxic nature.43–45,69,70 In boron nitride capture therapy, they are proposed to be the boron carriers.71 Upon injection, they are transferred into the tumor cell, produces localized charge particles by the interaction of neutron from an external source. These charge particles may be used to kill the tumor and cure the patient. The uniform distribution of Fe3O4 nanoparticles on the BNNTs surface introduced magnetic behavior in BNNTs. This behavior of BNNTs might be very useful in Micro Electro Mechanical System (MEMS) and targeted drug delivery.71,72
BNNT is a large band gap semiconductor with improved bulk properties, can be effectively used as a neutron sensing element in a solid state neutron detector.73 h-BN has a large cross-section for thermal neutron. In a solid state detector, a layer of semiconductor material is deposited on the top of neutron sensitive material. This layer is used for the production of electron–hole pair due to interaction of thermal neutron.74 Thus, if h-BN is replaced by BNNTs (with 10B-enrichement), the efficiency of the neutron detector is predicted to be far better than its bulk counterpart. BNNTs have highly crystalline multilayers structure that make it more suitable for a solid state neutron detector with higher detection efficiency.75 Being semiconductor, no other semiconductor layer is required to be deposited on BNNTs or h-BN surface. The as produced charge particles (due to interaction of neutron) are utilized within the same material.74 Thus the extra kinetic energy of the charged particles that was previously needed to accelerate them to semiconductor layers can now be saved and utilized for an increasing production of electron–hole pairs. Thus a neutron detector based on BNNTs will have high sensitivity and greater efficiency than any other existed solid state or semiconductor based neutron detector.76
All of the aforementioned BNNTs applications in different fields are dependent on its purity, size and alignment which in turn depend on the synthesis methods. The synthesis methods that are being used for producing BNNTs are arc-discharge, laser ablation, template synthesis, auto clave and ball milling etc.20 Beside high temperature, toxic precursors and complex and lengthy experimental procedures, these techniques were difficult to be followed by other researchers. Furthermore, different precursor's materials were found as impurities in the final product. These impurities are very difficult to separate from the final product.
CVD has been recognized as the most effective technique for the synthesis of high quality and large scale production of CNTs.77 This technique has not only increased the production or quality of the CNTs but has also provided simple mechanism for its growth in vertically aligned format.78 The effectiveness of this technique in CNTs synthesis also attracted the attention of the earlier researchers working on the synthesis of BNNTs. For the first time CVD was introduced to synthesize pure BNNTs from borazine as precursors. Though somehow pure BNNTs were obtained in the temperature range of 1000–1100 °C via this technique,79 however, due to toxic nature of borazine, the as-used technique was not further employed with borazine as precursors. The usefulness of CVD technique for BNNTs synthesis with high quality is further considered by other researchers. In this regard, they not only developed their own CVD techniques but also introduced most effective non-toxic precursors. Thus, the major developments in CVD synthesis of BNNTs can be categorized in three main stages. The first stage is from Tang et al. (2002)80 to Zhi et al. (2005).81 During this stage B, MgO and FeO were developed as effective precursors for high yield synthesis of BNNTs via Boron Oxide CVD (BOCVD) technique in an inductive furnace. The growth of the BNNTs was assumed to have followed “Vapor Liquid Solid (VLS) Growth Mechanism”.82 According to this mechanism (in the case of BNNTs), the reaction of the precursors powders at a particular temperature produce B2O2 vapors and nanosize liquid or partially melted catalysts particles. Later on, when the partial vapor pressures of these catalysts are sufficiently increased they are condensed on the substrate. The as formed B2O2 vapor reacts with N2 from NH3 gas and form BN-species. These species are then diffused in to the condensed catalysts and aggregates with the passage of time until it supersaturates and precipitates in the form of BNNTs.83
The second stage is from Zhi et al.81 (2005) to Lee et al. (2008).84 During this stage, a conventional horizontal tube furnace is used to replace the induction furnace. Furthermore, a particular ratio (2
:
1
:
1, 4
:
1
:
1) of the precursors (B, MgO, FeO) was used to grow pure BNNTs directly on Si/SiO2 substrate in a temperature range of 1100–1200 °C. The whole process thus developed was named as TCVD. The third stage is from Lee et al. (2008)84 to Ahmad et al.85 (2015). In this stage, the TCVD technique is further simplified with the use of argon gas (as a reaction atmosphere) as an alternative for vacuum or evacuation. As a result the process developed is called, “argon supported TCVD”. This simplification has not only reduced the required expertise and cost for the experimental set up but has also helped in growing longer BNNTs due to anti-oxidant quality of argon gas.
The complete description of CVD techniques: it comparison with earlier existed technique, its different types and importance of different experimental parameters in a particular CVD technique is given in the coming sections.
2 BNNTs synthesis methods
After being theoretically predicted in 1994 by A. Rubio and X. Blase et al.,4,5 arc-discharge was the first method employed for the synthesis of BNNTs. Single and multi-walls BNNTs (MWBNNTs) formed by this method had high crystallinity but the final product was found to contain BN onions and cages etc. as impurities.6,20 Due to insulating nature of BN materials, they were not suitable to use as electrodes. Therefore other conductive compounds of boron, such as ZrB2 and Yb6 were used as electrode during the arc-discharge synthesis of BNNTs.47,86 MWBNNTs synthesized via this technique were found to have diameter in the range of 1–3 nm. Metal particles were found at the tips of BNNTs. These particles were assumed to be responsible for the growth and nucleation of BNNTs by this technique.87–89
Laser ablation utilizes almost the same precursors and catalysts at a slightly higher temperature than the arc-discharge but the BNNTs obtained with this technique have the same characteristics as the one obtained with the arc-discharge method.42,90–92 This technique for the BNNTs growth was first introduced by Golberg et al.93 As precursor, single crystal of cubic BN (target) was heated with the help of CO2 laser up to 5000 K to obtain MWBNNTs.93 Later on, h-BN was also used as precursor in this technique94–99 and both SWBNNTs and MWBNNTs were successfully synthesized. It was found that fabrication of BNNTs via this technique can be achieved without catalysts.94 Highly crystalline BNNTs can be produced with the help of laser ablation, however, BN cones, onions and amorphous boron flakes can easily be found as impurities in the final product.
Template synthesis method uses CNTs substitution reaction100–105 or porous alumina anodic as a template106–108 for BNNTs synthesis. In the case of CNTs substitution, B2O3 are reacted with CNTs whereas nitrogen or ammonia is used as a protection gas and a nitrogen source. It is a low temperature method as compared to arc-discharge and laser ablation. As a result, the BNNTs produced by this technique have controlled morphology but has carbon in the lattice as an impurity, which is very difficult to remove from the final product.109–113 In the case of using porous alumina anodic as a template, 2,4,6-trichloroborazine are decomposed at 700–900 °C to fill the pores of alumina with BN material. The product thus obtained showed tubular structures with an average diameter of 280 nm, however, the detailed studies indicated a polycrystalline structure of the obtained BNNTs.20
Auto-clave is another low or room temperature technique for synthesis of BNNTs. In this kind of method for BNNTs synthesis, some special types of auto-clave are designed and used. BNNTs produced via this technique were found to have diameter in the range of 30–300 nm and length up to 5 micrometer. Though, this technique had produced BNNTs in different morphologies with thin walls and large inner space, however it yields poor quality and small quantity of the final product (BNNTs). Furthermore, BN cages and B were found as impurities in the synthesized BNNTs.114–117
Ball-milling technique for BNNTs synthesis was first developed by Chen et al.118 It gives multi-walled BNNTs (MWBNNTs) in a large quantity at a temperature range of 1000–1200 °C but the quality of the synthesized product was very low and reported B, h-BN and BN-fiber etc. as impurities.118–125
CVD is a more advanced and easiest technique for the synthesis of large quantity (gram level) of both CNTs and BNNTs with desired applicable properties. The quality of BNNTs is found to depend on the specific method (CVD types) applied for the synthesis. In all these techniques along with standard CVD, partial CVD is also involved combined with other techniques.6,13,30,42,79–81,116,126–140 Each CVD technique is different from other with respect to the experimental setup, required temperature and types of precursor. A brief summary of all the above techniques is given in the Table 1.
Table 1 A summary of different techniques used for BNNTs synthesis
S. no. |
Technique |
Temperature °C |
Advantages |
Dis-advantages |
References |
1 |
Arc-discharge |
∼3500 |
Highly crystalized, both SWBNNTs and MWBNNTs |
Containing BN onion and cages like morphologies as impurities |
6 and 20 |
2 |
Laser ablation |
∼1200–5000 |
Highly crystalized, both SWBNNTs and MWBNNTs |
Containing BN cones etc. as impurities |
90–92 |
3 |
Template synthesis |
750–1580 |
Having controlled morphology |
Containing carbon in lattice as impurities |
109–113 |
4 |
Auto clave |
600 |
Low temperature |
Bad quality, low yield and containing BN cages and B as impurities |
114–116 |
5 |
Ball milling |
1000–1200 |
Large yield |
Low purity with B, h-BN and BN fiber as impurities |
118–123 |
6 |
CVD |
1100–1200 |
High quality and large quantity up to gram level |
Search for a specific method (CVD type) with specific reaction parameters and precursors |
6, 13, 79, 116 and 126–135 |
It is clear from Table 1 that though high quality and large quantity of BNNTs can be obtained with CVD technique; however a specific CVD technique with easily available precursors and reaction parameters are still need to be studied in full detail. In this regard an up-to-date development in CVD techniques for BNNTs synthesis are discussed in the next section.
3 Classification of CVD for BNNTs synthesis
The BNNTs are synthesized via CVD technique by different groups of researchers. Each group used their own experimental parameters and precursors. As a result the quantity and quality of the synthesized BNNTs were found to vary from group to group. For the purpose of improvement in the results, some of the groups have adopted other techniques combine with CVD.116,141,142 This fact further notifies the importance of CVD techniques in BNNTs synthesis. In this section, the most prominently used CVD techniques for BNNTs are reviewed. The CVD method has been classified into various sub categories on the basis of growth mechanism, precursor's type and catalysts type for BNNTs synthesis. The classification of CVD for BNNTs synthesis is shown in Fig. 1 and discussed in details in the next section.
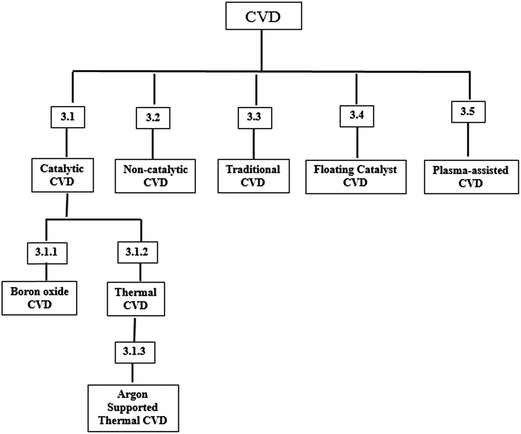 |
| Fig. 1 Block diagram of different CVD methods used for the synthesis of BNNTs. | |
3.1 Catalytic CVD
The first group who used CVD successfully for the synthesis of BNNTs was Lourie et al. (2000). They used borazine (B3N3H6) as the precursor. Ni2B was found as the most appropriate catalyst among all the other tested catalysts with borazine. A schematic of the proposed rout growth mechanism for BNNTs synthesis is shown in the Fig. 2. Silicon wafer substrate and NiB/Ni2B catalysts were placed in the center of a tube furnace. The furnace was then heated from 1000–1100 °C. A carrier gas containing borazine was flown into the system for 30 min. At this temperature borazine decomposed and produced BN and H2. H2 goes outside through the outlet whereas BN diffuses on nickel boride catalyst particle. With the passage of time BN aggregates leading to super saturation, and consequently result in precipitation of BNNTs on catalyst particle. The BNNTs thus formed have bulbous tips, possessed club like or flag like morphology with a length of approximately 5 μm.79
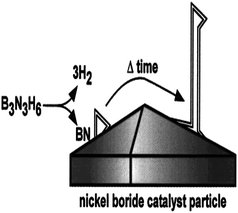 |
| Fig. 2 A depiction of the proposed root growth mechanism.79 | |
The earlier work on the CVD synthesis of CNTs shows that precursors and catalysts are the two main requisites along with the experimental set up and other parameters.143 Majority of these technique differ with respect to types and nature of catalysts and some of the experimental parameters.144–146 For example, CNTs were synthesized via CVD technique with methane gas as a precursor, Fe2O3/Al2O3 as catalyst and Ar gas as a reaction atmosphere to prevent the oxidation of the catalyst. In short, the catalyst was placed in the quartz tube furnace and heated in the presence of Ar gas flow up to 1000 °C. At 1000 °C, the Ar gas flow was replaced with the precursor gas methane and the system was maintained in such a condition for 10 min. After 10 min, the system was brought to room temperature in the presence of Ar gas flow.143 In the first CVD synthesis of BNNTs, NiB/Ni2B were used as catalyst and borazine gas as precursor. The substrate and catalysts were heated up to 1000–1100 °C and maintained for 30 min in the presence of borazine-containing carrier gas. Finally the white color product was obtained on the substrate.79 In order to further improve the quality, quantity and size of the BNNTs via catalytic CVD method, it was necessary to avoid toxic gas precursors. The major breakthrough occurred with the use of B and metal oxides as precursors and induction furnace as an experimental set up.147 Further improvements were sought by the replacement of induction furnace with conventional horizontal tube furnace84
If the above pioneer CVD work79 on the synthesis of BNNTs and the later on major developments81,84,85,147 are compared with the earlier work on CVD synthesis of CNTs,143 it would not be difficult to findout that majority of the concepts used in CVD of CNTs and BNNTs are somehow related or linked with each other. But, since both the materials are different, therefore, different types of precursor's powder or gases are used in each technique with different experimental parameters according to the nature of precursor's materials. On these basis the catalytic CVD is further divided into two new categories. The details are mentioned in the following sub-sections.
3.1.1 Boron Oxide CVD (BOCVD). The BOCVD technique for BNNTs synthesis was first developed by Tang et al. (2002). The use of carbon-free precursors and induction furnace in this method insured high quality of the synthesized BNNTs. As a precursor boron is used (in each experiment) with different metal oxides in different weight ratios. In this method boron powder react with metal oxides catalysts and form BxOy which then reacts with N free radicals from NH3 at certain temperature and forms BNNTs. In this regard, Tang et al. performed different experiment. Each experiment was different from the other by the precursor's type, catalysts, precursor's ratio and the environment in which the precursors and catalysts were used. First, BNNTs were synthesized by heating a mixture of boron and iron oxide powder (1
:
1) in the presence of ammonia gas. It was found that the ratio of the precursors and temperature plays an important role in the structure of the BNNTs.147 Second, BNNTs were synthesized by heating boron and alumina supported nickel boride catalyst in the presence of N2 or NH3 gas at a temperature range of 1100–1300 °C. The BNNTs thus produced have diameter of 5–40 nm and length of several micrometers. Defects in the structure of BNNTs were found at a temperature higher than 1300 °C. However, at a temperature above 1500 °C, no formation of BNNTs was observed.127 Third, BNNTs were synthesized with a large diameter of up to 70 nm and length greater than 10 μm by heating a mixture of boron and magnesium oxide powder in the presence of NH3. Boron and MgO were first mixed in a 1
:
1 ratio and then heated at a certain temperature to form B2O2 and Mg. Mg was evaporated from the final product whereas the synthesized B2O2 reacted with NH3 to form BNNTs according to VLS growth mechanism. BNNTs thus formed were in the pure form. In a smaller diameter of around 10 nm, the nanotubes were straight with concentric tubular structure containing no defects. Defects were found in the nanotubes with a diameter of about 60 nm.80Zhi et al. (2005) further developed the BOCVD technique for high yield synthesis of BNNTs. The details of the synthesis method are mainly similar to the one described elsewhere.118 However, some modifications were done to obtain high quality results. In short, a mixture of B, FeO and MgO was taken in BN boat and heated in an induction furnace up to 1200 °C. As a result, B2O2 vapors were formed. NH3 was introduced from the top of BN boat. At this temperature NH3 decomposed into N2 and H2. H2 reacted with oxygen from B2O2 and formed water vapors whereas N2 reacted with boron and formed BNNTs. In this study, it was found that BNNTs can be produced in a temperature range of 1100–1700 °C with an average diameter of ∼50 nm and almost no impurity present in the final product. The growth of the BNNTs was catalytic based on VLS model.81
Goldberg et al. (2010) achieved large scale production of BNNTs up to 2 grams via BOCVD method80 (see in Fig. 3). For high purity of the BNNTs, the design of the induction furnace was optimized. The basic reason for this optimization was to separate boron and metal oxide precursors for the BNNTs synthesis. The BNNTs have a diameter of approximately 50 nm and a length of up to tens of micrometers synthesized in this way.148
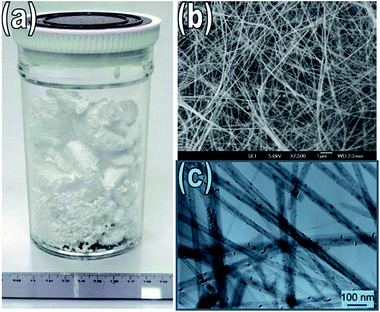 |
| Fig. 3 (a) Image of as synthesized 2 grams BNNTs (b) SEM and (c) TEM image of multiwall BNNTs.148 | |
Huang et al. (2011) introduced B and Li2O as precursor in BOCVD technique, and successfully achieved bulk amount of pure and thin BNNTs. It has been discovered that Li, due to its superior oxidation and promotion effect on the crystallization, played a key role in the large scale synthesis of BNNTs with small diameter. The synthesized BNNTs had an average diameter of sub-10 nm and a length of greater than 10 micrometers.149
Li et al. (2013) tried to enhance the effect of B and Li2O as precursor via further modification in BOCVD technique. The precursors (i.e. B and Li2O149) were first ball milled in the presence of NH3. The ball milled precursors were then moved into a horizontal tube furnace and heated from 2–3 hour in the presence of NH3 flow. White feather like BNNTs was found inside the boat and on the Silicon substrate. During the milling process the catalyst increased B oxidation and nitridation which was considered to be the possible reason for high yield of BNNTs.150
Yamaguchi et al. (2013) successfully synthesized 1 g of multiwall BNNTs via BOCVD technique reported elsewhere.49,151–154 The synthesized BNNTs were long up to 1 to 5 μm and external diameter in the range of 40 to 50 nm. The tubes were then subsequently purified at high temperature in the Ar atmosphere. Later on it was utilized for the reinforcement of lightweight aluminum ribbons.155 The BNNTs synthesized by different researchers via BOCVD technique are summarized in Table 2.
Table 2 Summary of BNNTs grown by various researchers via BOCVD
S. no. |
Year |
Precursors |
Precursors ratio |
Substrate |
Temperature (°C) |
Catalysts |
Growth mechanism |
Final comments |
References |
1 |
2002 |
Boron powder |
Not mentioned |
Without any substrate |
1100–1300 |
NiB/Al2O3 |
VLS |
Diameter 5–40 nm, length several μm with bamboo and polar like BNNTs |
127 |
2 |
2002 |
B, MgO |
1 : 1 |
Without any substrate |
1300 |
Mg. Nanoscale catalyst |
VLS |
Pure, diameter around 10 nm. Defects found in nanotubes with a diameter of about 60 nm |
80 |
3 |
2005 |
B, FeO and MgO |
Not mentioned |
Not mentioned |
1100–1700 |
Fe, Mg |
Catalytic growth based on VLS |
Diameter 50 nm, no impurity |
81 |
4 |
2011 |
B and Li2O |
1 : 1 |
Not mentioned |
1100–1350 |
Li |
Not mentioned |
Diameter sub-10 nm, length greater than 10 μm |
149 |
5 |
2013 |
B and Li2O |
1 : 1 |
Si/SiO2 |
Not mentioned |
Li |
Not mentioned |
White feather like morphology |
150 |
6 |
2013 |
B, FeO and MgO |
1 : 1 : 1 |
Not mentioned |
Not mentioned |
Mg, Fe |
Not mentioned |
Diameter 40–50 nm, length 1–5 μm |
155 |
Though the introduction of BOCVD was a significant progress regarding the mass production of BNNTs, which led the researchers towards its potential application in nanotechnology.16,81 However, this technique requires a specific design of induction furnace for achieving higher temperature with high temperature gradient. Further, it was found that this technique cannot be used for coating of BNNTs on Si based substrates without the assistance of other techniques and precursors.
3.1.2 Thermal CVD (TCVD). Lee et al. (2008) reported a simple TCVD for the synthesis of BNNTs directly on Si/SiO2 substrate. The system thus developed is almost the same as previously used for the synthesis of CNTs.156 However, for obtaining high quality of BNNTs, some changes have been brought in the existed technique. Instead of gaseous precursors and deposited catalysts on the substrate, a mixer of powder precursors and catalysts was used as a starting material. For the first time in BNNTs synthesis, a new concept of growth vapor trapping (GVT) was introduced with the use of one end closed inner test tube. The precursors used were: B, MgO and FeO in a molar ratio of 2
:
1
:
1. All the precursors were put in alumina combustion boat. The boat was placed in one end closed quartz tube. The quartz tube was then pushed inside a quartz chamber. The quartz chamber was first evacuated to ∼30 mTorr and then heated up to 1200 °C. At this temperature NH3 gas was introduced into the chamber at a rate of 200 sccm for one hour. The BNNTs were formed in pure form on the inner walls of the boat and partially vertically aligned on silicon substrate placed on the boat. A view of the as-grown BNNTs is shown in the Fig. 4. The diameter of the tubes was in the range of 10–100 nm with a length of greater than 10 μm. The as-synthesized, partially vertically aligned BNNTs, were not powerful enough to stand against even a small mechanical compression which make them laid horizontally on silicon substrate.84
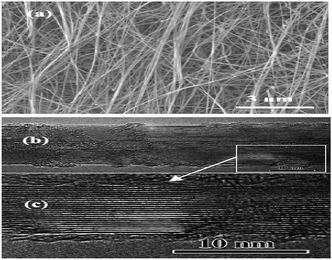 |
| Fig. 4 (a) SEM (b) low and (c) high magnification TEM images of the as-synthesized BNNTs by TCVD.84 | |
Lee et al. (2010) further used his own designed TCVD and obtained pattern growth of BNNTs. Transmission electron microscope (TEM) copper grids were used as pattern. The used precursors were the same as the one mentioned elsewhere,81,84 but with a different weight ratio of 4
:
1
:
1. The system was heated in a range of 1100–1200 °C in the presence of NH3 flow, at a rate of 200–350 sccm for approximately 30 min. The purpose of one end closed small quartz tube was to obtain growth vapor trapping (GVT). For the patterned growth, TEM copper grid was first mounted with the help of adhesive tape on the silicon substrate. Pulse Laser Deposition (PLD) was used to deposit a thin layer of Al2O3 film of 30 nm, followed by MgO, Ni or Fe film of 10 nm thicknesses on silicon substrate. After deposition, the TEM grid was removed and the substrate was transferred to the CVD system for the synthesis of BNNTs. The BNNTs formed in this way (have followed base growth mechanism according to VLS model) have diameter of 60 nm and average length of greater than 10 μm, as shown in Fig. 5.157 The idea used in the initial treatment of the substrate for obtaining the pattern growth157 is somehow similar to the one used for the synthesis vertically aligned CNTs. However, in case of CNTs Co, Ni or their alloys (Co–Ni) are first deposited at the substrate and then etch with NH3 to produce nucleation sites for the synthesis of vertically aligned CNTs.158 Whereas, in the pattern growth of BNNTs Al2O3 film, MgO, Ni or Fe film were deposited at the substrate surface. No separate NH3 etching was employed to produce nucleation sites. Therefore, the BNNTs product thus obtained was partially vertically aligned and was not strong enough to stand a slight mechanical compression.157
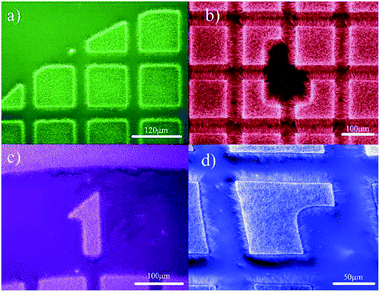 |
| Fig. 5 Partially vertically aligned BNNTs grown in a particular patterned.157 | |
Ferreira et al. (2011) used a novel synthesis route based on TCVD technique and successfully synthesized BNNTs from a mixer of amorphous boron powder, ammonium nitrate and hematite. Ammonium nitrate and hematite were used as catalyst. The characterization results showed that the synthesized BNNTs were filled with Fe nanoparticles. Such BNNTs were predicted to be useful in some of the biomedical applications.159
Pakdel et al. (2012) comprehensively studied the CVD growth of BNNTs in a horizontal electric tube furnace, using B, MgO, and FeO as precursors with different weight ratio of 1
:
1
:
1, 2
:
1
:
1, and 4
:
1
:
1, at a temperature of 1200 °C, 1300 °C and 1400 °C, respectively. The effects of different transition metals or their compounds have been tested as catalysts for the growth of BNNTs. However the results of their reaction with boron were not satisfied. Therefore the precursors were selected following the earlier work by Zhi et al. (2005). It was found that the growth temperature and metal oxides content have a significant effect on the structure, morphology and characteristics of the BNNTs. An increase of the temperature above 1200 °C resulted an increase in the tube diameter, and further increase to 1400 °C resulted in a secondary growth of very thin nanotubes. Nanotubes synthesized by using 2
:
1
:
1 ratio of the precursors at 1200 °C and 1300 °C has an average outer diameter of approximately 30 nm and 60 nm, respectively and were uniformly dispersed. At 1400 °C the tubes grown have flower like morphology with a diameter of ∼20 nm and ∼100 nm. For 1
:
1
:
1 and 4
:
1
:
1 ratio of the precursors, no appreciable result was found at 1200 °C and 1400 °C. However at 1300 °C, the 1
:
1
:
1 ratio has a diameter in the range of ∼100–500 nm with flower like morphology, and 4
:
1
:
1 ratio has diameter in the range of ∼50–150 nm with entangled curve like morphology. Tip, base-growth or mix-growth mechanism (based on VLS mechanism) was proposed for the growth of the tubes.83
Seo et al. (2012) combined TCVD with ball milling annealing process and successfully synthesized relatively pure BNNTs (according to VLS mechanism). During this process, the production of impurities was a great concern in the residual material. It was concluded that the reaction parameters such as precursors, temperature and annealing time can sufficiently modify the final product.160
Özmen et al. (2012) adopted a TCVD method in which BNNTs were synthesized by heating a mixture of boron and iron oxides powder over 900 °C in the presence of NH3. The temperature and the ratio of B to Fe2O3 were found to have a great effect on the crystallinity of the synthesized materials. N2 produced during the decomposition of NH3, reacted with FeXBY, (produced by the reaction of boron with iron oxide) and synthesized BNNTs. Characterization of the samples showed bamboo and hollow cylindrical morphology of the BNNTs with inner diameter in the range of 7 nm to 38 nm.161 Growth mechanism and physical properties of BNNTs grown via TCVD is shown in Table 3.
Table 3 Growth mechanisms and physical properties of BNNTs grown by TCVD
S. no. |
Year |
Precursors |
Precursors ratio |
Substrate |
Temperature (°C) |
Catalysts |
Growth mechanism |
Final comments |
References |
1 |
2008 |
B, MgO and FeO |
2 : 1 : 1 |
Si/SiO2 |
1200 |
FeO, MgO |
VLS |
Diameter 10–100 nm, length greater than 10 μm |
84 |
2 |
2010 |
B, MgO and FeO |
4 : 1 : 1 |
Si/SiO2 |
1100–1200 |
MgO, Ni, or Fe |
Base growth according to VLS |
Diameter 60 nm, average length greater than 10 μm |
157 |
3 |
2011 |
B, NH4NO3 Fe2O3 |
15 : 15 : 1 |
Not mentioned |
1300 |
Fe2O3 |
Not mentioned |
BNNTs were filled with Fe nanoparticles |
159 |
4 |
2012 |
B, MgO, FeO |
1 : 1 : 1, 2 : 1 : 1, 4 : 1 : 1 |
Si/SiO2 |
1200–1400, 1200–1400, 1200–1400 |
Mg, Fe |
Tip-growth. Base growth, mix growth according to VLS mechanism |
Diameters ∼30 and ∼60 nm at 1200 and 1300 with straight morphology. ∼10 nm and ∼100 nm at 1400 °C with flower-like morphology |
83 |
5 |
2012 |
B, MgO, FeO |
2 : 1 : 1 |
Not mentioned |
1200 |
Mg, Fe |
VLS |
Pure and quantity can be increased via milling-annealing + TCVD |
160 |
6 |
2012 |
B and Fe2O3 |
15 : 1, 5 : 20 |
Not mentioned |
900–1400 |
Fe |
Not mentioned |
BNNTs with bamboo and hollow cylindrical morphology |
161 |
Though TCVD is a simple technique to grow BNNTs directly on Si/SiO2 substrate. However, this technique was unable to give a specific idea to get BNNTs with an increasing length. Furthermore, vacuum or evacuation is need as a reaction atmosphere inside the chamber, which require vacuum pump an additional accessory with the conventional horizontal tube furnace. The requirement of the vacuum pump not only increases the cost of the experimental set up but also needed further study about vacuum and extra expertise to run the vacuum pump.
3.1.3 Argon supported TCVD. Ahmad et al. (2015) synthesized BNNTs via argon supported TCVD technique. Argon gas flow was employed as an alternative for vacuum or evacuation. Ar gas being inert does not react with any material. Its flow through the system helps in the removal of oxygen and dust particles and thus helps in creating a suitable atmosphere for the desired chemical reaction to occur. So it was sought to be a good alternative for the vacuum or evacuation in CVD. Two minutes before the experiment, Ar gas was passed through the system and then a continuous flow was maintained at a rate of 100–200 sccm up to 1100 °C. After the completion of the reaction time Ar gas was again introduced into the system that flew away the water vapors, hydrogen, oxygen and BN species (left behind during the BNNTs synthesis) that might affect the final product quality.It was found that the flow of Ar gas is playing a significant role in a simple and cost effective synthesis of BNNTs. As an alternative for vacuum, argon gas flow has eliminated the use of vacuum pump, which in other words have made the experimental set up ∼18% cost effective as compared to the conventional set up. Thus no extra knowledge or expertise is needed about vacuum or vacuum pump. Furthermore, the anti-oxidant nature of the argon gas prevents the oxidation of the as produced Mg and Fe catalysts, which in other words helps in growing longer BNNTs.85
3.2 Non-catalytic CVD
Ma et al. (2001) synthesized BNNTs via CVD technique, without using any catalyst. Melamine (C3N6H6) and boric acid (H3BO3) were used as starting materials. These starting materials were first mixed in 2
:
1 ratios to form a hot aqueous solution. This hot solution crystallized into melamine diborate (C3N6H6·2H3BO3) as the CVD system cooled down. After annealing melamine diborate in N2 environment, B–N–O was formed. It was then heated rapidly in N2 gas stream to form BNNTs. The multiwalls BNNTs synthesized by this method have 12 concentric layers with inner diameter of 5.2 nm and outer diameter of 13.1 nm. The as-synthesized nanotubes were found to have a length of few micrometers with bulbous tips at the end. These tips were then used to grow the nanotubes.162
BNNTs were also synthesized by using α-Al2O3 micrometer size particles as the substrate. The purpose of that work was to check the effect of substrate on the final product. The as-synthesized BNNTs were found to have inner diameter of at least 5 nm larger than the one previously described,162 with the same layers. Thus the substrate was found to affect the diameter of BNNTs within CVD technique. BNNTs filled with amorphous B–N–O materials were also observed in the final product.163 Later on it was pointed out by the same group with the help of further experimental work that employing the same CVD technique result in different morphologies of the nanostructures depending on the experimental parameters such as temperature and content of oxygen in the precursor.164 A summary of BNNTs synthesized via non-catalytic CVD is shown in Table 4.
Table 4 Summary of BNNTs synthesized by non-catalytic CVD
S. no. |
Year |
Precursors |
Precursors ratio |
Substrate |
Temperature (°C) |
Catalysts |
Growth mechanism |
Final comments |
References |
1 |
2001 |
Melamine (C3N6H6) and boric acid (H3BO3) |
1 : 2 |
Without any substrate |
1200 |
Without any catalyst |
Tip growth |
BNNTs bulbous tips at the end |
162 |
2 |
2001 |
Melamine (C3N6H6) and boric acid (H3BO3) |
1 : 2 |
α-Al2O3 |
1200 |
Without any catalyst |
Not mentioned |
BNNTs filled with amorphous B–N–O materials |
163 |
3 |
2002 |
BN powder |
Not mentioned |
Without any substrate |
1700 |
Not mentioned |
Not mentioned |
Morphology depends on modification in CVD set up w.r.t. precursor, temperature |
164 |
3.3 Traditional CVD
Traditional CVD technique was used to synthesize F-doped BNNTs. A diagram of the experimental setup along experimental parameters and precursors variables is shown in Fig. 6. In this method a Basic BN crystal was first synthesized by using BF3/NH3 as precursor. Then at the growth stage, F-atoms were introduced to form F-doped BN crystal. The synthesized F-doped BN crystal had nanotubes like morphology. The length and diameter of the tubes were estimated from the scale shown in the SEM micrograph of the sample, whereas the F-doped BNNTs had a length of few micrometers and diameter in tens of nanometers.165
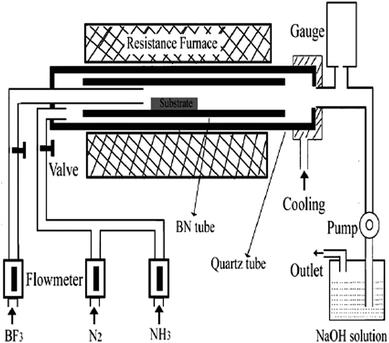 |
| Fig. 6 Traditional CVD setup used in the synthesis of F-doped BNNTs.165 | |
3.4 Floating catalyst CVD
Multiwalls and double-wall BNNTs have been synthesized by floating catalyst CVD with the help of floating nickel catalyst, using two different molecular precursors; amine borane borazine and polyhedral-borane decaborane are separately used in NH3 atmosphere at 1200 °C and 1300 °C respectively. Both precursors resulted in different size and morphologies of the BNNTs. Double-walls BNNTs synthesized from borazine at 1200 °C were found to be 0.2 μm in length and about 2 nm in diameter. The size of multiwall BNNTs synthesized from decaborane was found to be affected by the temperature. The one synthesized at 1200 °C had inner diameters from ∼4–5 nm and outer diameters from ∼12–14 nm with a length of ∼0.2 μm, whereas the other synthesized at 1300 °C had a length of ∼0.6 μm.166
Another important CVD technique based on floating catalyst was also introduced128 later on to obtain good quality and large quantity of BNNTs. It has been successfully used for synthesis of double walled BNNTs. It is found that this technique might be an optimized step toward the continuous growth of BNNTs by CVD technique.128
3.5 Plasma-assisted CVD
Microwave plasma-assisted CVD technique was used to synthesized BNNTs at low substrate temperature by using transition metals as catalyst, and diborane and ammonia as precursors. The nanotubes thus formed have a diameter of less than 10 nm. The morphology and the structure of the nanotubes were found to be strongly affected by the growth condition. Micro-sized Ni dots were used to obtain patterned growth of BNNTs. This patterned growth was considered to be one of the possible ways for the synthesis of BNNTs in the aligned orientation.167
Large scale synthesis of BNNTs at low substrate temperature has also been reported by plasma-assisted CVD technique. Diborane and ammonia were used as precursors whereas Fe (powder) was used as supporting catalyst. The yield of BNNTs was found to be increased by the addition of O2 in the synthesis process. A growth model has also been proposed (as shown in Fig. 7) to properly explain the role of metal catalysts during the synthesis or growth of BNNTs. It was suggested on the basis of the obtained results that this technique can be used to achieve large scale and high quality of BNNTs at low temperature.168
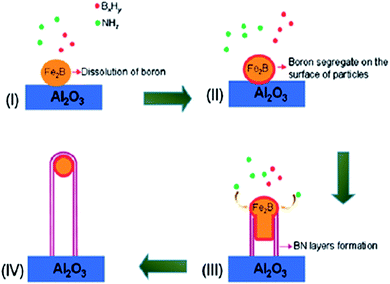 |
| Fig. 7 Different steps involved during the growth of BNNTs via plasma-assisted CVD technique.168 | |
The study of all the above CVD techniques showed that the quality, quantity, size and morphology of the BNNTs depend on certain variables like precursor's types, their ratio, temperature, catalyst and growth mechanism etc. Therefore all these variables and their importance in different CVD techniques regarding their effect on the final BNNTs products are fully discussed in the next section.
4 Discussion
BNNTs have been synthesized by different CVD techniques. Each technique is different from the other w.r.t. the used experimental set up, precursors, their ratios, substrate, and temperature, catalyst and growth mechanism.79–81,163 Therefore the final product differ with respect to quality, quantity, size and morphology. The importance of all these parameters is fully discussed in the following sections:
4.1 Importance of B, MgO and FeO as precursors for BNNTs synthesis
BNNTs have been synthesized in different sizes and morphologies by using different precursor's materials. The precursors utilized by the initial groups20 have although produced pure BNNTs in different sizes and morphologies, however the toxic nature of some of the precursors have made it difficult for other researchers to reproduce BNNTs by the same methods.
A novel method based on VLS169 mechanism has been proposed and developed by Tang et al.127,132,170 In this method B and MgO were used as precursors. Though pure BNNTs were synthesized from these precursors however to increase the quantity at higher temperature, it resulted in bulk BN flakes. Also the growth of the BNNTs was very slow due to slow catalytic activity of Mg. Furthermore, B and MgO precursors become chemically inactive due to the formation of Mg2B2O5 after first experimental run which cannot be used for further synthesis. Thus a major portion of these precursors had been wasted by this way, and only a small portion was utilized for BNNTs synthesis.81
Due to the common use of transition metals as catalysts and need of oxygen for B2O2 production, FeO has been used with boron powder to obtain a high quality result. However, it was discovered that the chemical reaction effectiveness of B and FeO was not satisfying and very little amount of B2O2 was produced in this reaction at a higher temperature. In order to combine the effect of both MgO and FeO, they were collectively used with boron powder, and BNNTs were produced in a wide temperature range of 1100 °C to 1700 °C. No notable impurity or bulk BN flakes were observed in the final product. During this method the growth of the BNNTs stop only when the precursor in the boat is blocked by the initially formed BNNTs at their top. The blockage of the precursors keeps the underneath precursors unreactive. Therefore, when the BNNTs top surface is removed, the remaining precursors can be used for several syntheses. This is the most important advantage of the above precursors (B, MgO, and FeO). It was also found that the quantity of the final product can easily be increased by using a larger size boat.81
Later on, some other researchers83,84 used the above precursors with different weight ratios (1
:
1
:
1, 2
:
1
:
1 and 4
:
1
:
1). The best results i.e. BNNTs with diameter from 10–100 nm and length of greater than 10 μm were obtained with 2
:
1
:
1 ratio of the precursors.83,84
Further developments regarding quantity and quality of BNNTs (when B, MgO and FeO are used as precursors) were sought by the addition of ball-milling and annealing along with TCVD. In this method the precursors i.e. B, MgO and FeO were first ball-milled and then annealed in the presence of reactant gases (nitrogen and ammonia). The obtained results suggested that this combine method (TCVD and ball milling and annealing) can be used to increase the yield of BNNTs and reduces the residual impurities left in the boat which was normally observed by Lee et al.84 by controlling the parameters like temperature, annealing time, ratio of the precursors and types of the reaction gases etc.160 Though efforts have done to brought improvements in the existed method, however the adopted procedure was quite lengthy and relatively difficult to be followed by other researchers relative to the already existed methods.81,83,84
Again boron and iron oxide (Fe2O3) were used as precursors to synthesized BNNTs. Different weight ratios of B to Fe2O3 i.e. 15
:
1 and 5
:
1 were used. It was found that the ratio of the precursors has a great effect on the crystallinity of the final product. The crystallinity increases by increasing the weight ratio of boron to iron oxide. The surface area of the as synthesized BNNTs was also found to be affected by the weight ratio of B to Fe2O3 and increases by increasing this ratio. It was further found that the color of the final product become darker by decreasing the weight ratio of B to Fe2O3.161 Recently, BNNTs have been synthesized from B, MgO and FeO, according to the procedure mentioned elsewhere.49,151–154 The as-produced product can easily be functionalized for its potential applications in optical devices.171–175
Thus the synthesis of the BNNTs by the above mentioned methods emphasizes the fact that B, MgO and FeO are the most effective precursors for the synthesis of pure BNNTs. This fact has been summarized in the Table 5. According to the analysis shown in the Table 5, there are some disadvantages of using B, MgO and FeO as precursors; however it is also clear that these disadvantages are so minor that they can easily be eliminated.
Table 5 Analysis of B, MgO and FeO as an effective precursor for BNNTs synthesis
S. no. |
Year |
Precursors |
Advantages |
Dis-advantages |
1 |
2001 (ref. 147), 2005 (ref. 81), 2012 (ref. 161) |
B and Fe2O3 (ref. 147), B and FeO,81 B and Fe2O3 (ref. 161) |
1. High yield of BNNTs by varying precursor ratio from 5 : 1 to 15 : 1 (ref. 161) |
1. Contained α-Fe as impurities147 |
2. Increased crystallinity with increasing ratio of B to Fe2O3 (ref. 161) |
2. Formation of BN bamboo with high concentration of Fe2O3 (ref. 147) |
3. Change of BNNTs color with changing ratio of precursor and become darker by reducing the B to Fe2O3 ratio161 |
3. Produces little B2O2 at higher temperature due to unsatisfied chemical reaction effectiveness between B and FeO that result in slow growth rate and lower amount of BNNTs81 |
2 |
2002, 2002, 2004 |
B and MgO127,132,170 |
1. Carbon free method132 |
1. Results in bulk BN flakes at higher temperature |
2. Produces pure BNNTs127,132,170 |
2. Slow growth due to very slow catalytic activity of Mg |
3. B and MgO are effective source of B2O2 (ref. 81) |
3. B and MgO precursors become chemically inactive due to formation Mg2B2O5 after first run81 |
3 |
2005 (ref. 81), 2008 (ref. 84), 2010 (ref. 157), 2012 (ref. 83) |
B, MgO and FeO81 |
1. Pure BNNTs with diameter ∼50 nm can be achieved81 |
1. The ratio of the used precursors is not clearly mentioned81 |
2. Temperature has no effect on the purity and diameter of the BNNTs81 |
2. Need nanoscale catalyst to grow in nano-size diameter84 |
3. Precursors can be used for several synthesis81 |
3. Possibility of forming ternary compounds (small amount) like: MgN2, MgB2 and Mg–B–N etc., when MgO is used as a catalyst157 |
4. A larger boat size is helpful in increasing the final product81 |
|
5. Need a very sample experimental set up84,157 |
|
6. Diameter of the tube varies from 10–100 nm with length greater than 10 μm84 |
|
7. Pattern growth is possible in the desire format157 |
|
8. Allow control growth of BNNTs157 |
|
9. Desired morphology and diameter can be achieved by changing the precursors ratio and temperature83 |
|
4.2 Importance of experimental set up
Experimental set up has a key role in a good quality and large quantity of BNNTs synthesis. Different experimental set up have been developed and used for the CVD synthesis of BNNTs. All of these experimental set up consist of either lengthy and complex procedures,168 or high temperature.162 Due to which they have not been followed by other researchers. A significant progress has been made in this regard by the development of BOCVD technique.80,81 This technique employs an induction furnace as an experimental set up to synthesize BNNTs within a temperature range of 1100 to 1700 °C.81 The major drawback with the induction heating was the effect of temperature to the surrounding growth area, where the increase of the temperature can greatly modify the size and morphology of the BNNTs. This fact has been realized later on, therefore efforts were made on the design of most simple experimental set up where not only temperature can be controlled at the specific growth area but also be used to analyze the growth with increasing temperature.83,84,157 In this regard the first experimental set up was designed using a conventional horizontal tube furnace with a quartz tube vacuum chamber. It further consisted of a one end closed quartz tube that was placed inside the quartz tube chamber. Precursors were put inside the one end closed quartz tube for the growth of the BNNTs. The system was then sealed and evacuated to a certain level. The precursors were then heated up to 1200 °C and kept for 1 hour at this temperature in the presence of NH3 flow. BNNTs thus formed were observed inside the boat and on the silicon substrate.84
A sketch of such an experimental set up and the BNNTs product obtained is shown in the Fig. 8. It has been experimentally verified that the one closed end quartz tube is one of the most important requirement for BNNTs growth by the experimental set up shown in the Fig. 8. In other words, BNNTs growth cannot be achieved if the closed-end quartz tube is replaced with an open-end tube.84 Later on, the pattern growth of BNNTs has been achieved by using the same experimental set up.157 Almost the same experimental set up, precursor type (B, Mg, FeO) and ratio (2
:
1
:
1),84 with some additional treatment (ball-milling) was used for synthesis of BNNTs at a bit higher flow rates of NH3 and N2 gases at 1200 °C for 6 hours.160 Such an experimental set up has also been successful when B and Li2O were used as precursors.149,150
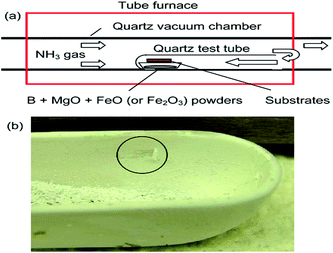 |
| Fig. 8 (a) Sketch of the experimental set up used for BNNTs synthesis at 1200 °C (b) as synthesized BNNT inside alumina boat.84 | |
A relatively different experimental set up with respect to the one reported by Lee et al.,84 has been developed to synthesize BNNTs at 1200 °C, 1300 °C and 1400 °C, by using 1
:
1
:
1, 2
:
1
:
1 and 4
:
1
:
1 ratio of B, MgO and FeO as precursors, respectively. In previous experimental set up (Lee et al.84), the used vacuum chamber and test tube were made of quartz,84 whereas in the present experimental set up these devices were made of alumina.83 However, neither in the previous work nor in the present any effect of quartz or alumina (heat gain or loss) has been observed on the final product. Therefore both of the set up are almost the same except the use of one end closed test tube inside the chamber. In Lee et al.84 experimental set up, one end of the test tube was closed and it has been experimentally verified that BNNTs cannot be synthesized if the closed end test tube is replaced by an open end test tube.84 However in present work, the synthesis of BNNTs have been achieved with an open end test tube,83 which was experimentally denied by Lee et al.84 High vacuum (evacuation of chamber) and high flow rate of NH3 (400 sccm compare to 200 sccm) may be the only possible reason for the present results.83 However none of the two groups have ever considered either the effect of high vacuum or flow rate of NH3 for possible change in the experimental set up or BNNTs product by using the same precursors.83,84 Both types of experimental works have been summarized in Table 6.
Table 6 Summary of BNNTs synthesized from same precursor but in different experimental set up
S. no. |
Year |
Precursors |
Vacuum in Torr |
Temperature (°C) |
NH3 flow rate in sccm |
Conventional horizontal tube furnace as experimental set up |
With one closed-end test tube |
With opened end test tube |
1 |
2008 |
B, MgO, FeO |
∼30 mTorr |
1200 |
200 |
BNNTs synthesized with 10–100 nm diameter and length greater than 10 μm |
BNNTs cannot be synthesized |
2 |
2012 |
B, MgO, FeO |
∼1000 m Torr |
1200 |
400 |
BNNTs synthesis was not reported |
BNNTs synthesized with diameter ∼30 nm |
A sample conventional horizontal quartz tube furnace without any inner one-end closed test tube has been used to synthesize BNNTs at a temperature lower than 800 °C. However the lengthy and time consuming process involved in the synthesis of the precursors materials, and understanding of the chemical reactions have made it difficult to be followed by other researchers.176
The choice of experimental set up changes with change in precursors, and use of Ar gas as an alternative for vacuum. Such changes have been observed by different researchers like Özmen et al.161 Therefore, it is possible that in the near future the above mentioned experimental set up,83,84 may easily be used with Ar gas as an alternative for vacuum (or evacuation). The use of Ar gas as an alternative for vacuum may not only further simplify the experimental set up but will also make it safe for the user.
4.3 Importance of temperature
Temperature is the most important factor during the synthesis and growth of BNNTs in a CVD technique. Specifically, size and morphology of the BNNTs varies with temperature.166 The initial group synthesized BNNTs in a temperature range of 1100–1200 °C. The as synthesized BNNTs have flag like morphology with bulbous tip and length of about 5 μm.79 The yield, diameter and morphology of BNNTs synthesized in a temperature range of 1200–1500 °C changes with increase in temperature. At a temperature below 1350 °C, the BNNTs have wool like structure with diameter 7 to 20 nm. The yield increases when the reaction temperature is fixed at 1250 °C. At this temperature a grey sponge like BNNTs can also be found with diameter 12 to 40 nm.147 A change in morphology from straight177 to bamboo,178 and fiber179 like has been observed with the change in temperature.164 Defects were found in the BNNTs structure at a temperature higher than 1300 °C. When the temperature was increased from 1400 °C to 1500 °C, bamboo and polar like morphologies were observed. However, when the temperature was further increased beyond 1500 °C, no formation of BNNTs was reported.127
The effect of temperature also depend on the type of precursor used for BNNTs synthesis.81,149 When B and MgO were used as precursor, good quality of BNNTs was obtained at a temperature below 1100 °C. The yield increased along with the tube diameter at higher temperature. The diameter reached to micron size with further increase in temperature. At a temperature higher than 1250 °C, BN flakes were observed in the final product. When B, MgO and FeO were used as precursors, BNNTs were synthesized in a wide temperature range of 1100 °C to 1700 °C. Higher temperature was found to have no effect on the purity and diameter of as synthesized BNNTs.81 When B and Li2O were used as precursors, BNNTs with a smaller diameter were formed at a temperature of 1100 °C. An increase in temperature above 1100 °C resulted in a thick and non-uniform diameter of BNNTs.149 The temperature effect also varies with precursor's ratio along with precursor's type. For B, MgO and FeO as precursors with 1
:
1
:
1 and 4
:
1
:
1 ratios, no synthesis of BNNTs was reported at 1200 °C and 1400 °C. The synthesis of BNNTs from 1
:
1
:
1 and 4
:
1
:
1 ratio was reported only at 1300 °C. At this temperature the as synthesized BNNTs from 1
:
1
:
1 ratio had semi erect flower like morphology with diameter ∼100–500 nm. Whereas the one synthesized from 4
:
1
:
1 ratio had diameter ∼50–150 nm with entangled curve like morphology. The BNNTs synthesized from 2
:
1
:
1 ratio of the precursors at 1200 °C had diameter ∼30 nm, and were straight and randomly oriented in different directions. When the temperature was increased from 1200 °C to 1300 °C, the morphology remained the same but the diameter increased from ∼30 nm to ∼60 nm. With further increase in temperature up to 1400 °C both diameter and morphology of the BNNTs changed. At this temperature the BNNTs synthesized have diameter ∼20 to ∼100 nm with straight flower like morphology.83 This analysis of BNNTs with increasing temperature83 is somehow contradicts with the work of Zhi et al.,81 in which higher temperature was found to have no effect on purity and diameter of the as synthesized BNNTs. Change in the experimental set up and ratio of the precursor used may be one of the possible reason for this conflict. However, none of these researchers81,83 had ever mentioned the experimental set up (to be responsible) to bring a change in size or morphology with the change in temperature.
Temperature is also found to affect the crystallinity and color of the final product in BNNTs synthesis. The crystallinity of the product was found to increase with an increase in temperature. Change in the color and structure of the product was observed with increasing temperature. At 900 °C and 1000 °C, the material was in a particular structure with light brown color. When the temperature was increased to 1100 °C, the product was converted into powder form with brown color. The color changes from brown to grey with particular structure at 1200 °C. A mixer of grey and white color was found at 1300 °C which was converted to only white color at 1400 °C.161 A concrete description of the temperature effects on size and morphology are summarized in Table 7.
Table 7 Summary of temperature effect on size and morphology of BNNTs
S. no. |
Precursor |
Temperature (°C) |
Effect of temperature (°C) |
Size |
Shape or morphology |
References |
1 |
B3N3H6 |
1100–1200 |
Length 5 μm |
Flag like |
79 |
2 |
B and Fe2O3 |
1. Below 1350 |
1. Diameter 7–20 nm |
1. Wool like |
147 |
2. 1250 |
2. Diameter 12–40 nm |
2. Grey sponge like |
3 |
1. BN powder |
1700 |
1. Diameter 10–100 nm and length ∼10 μm |
1. Bamboo like |
164 |
2. B4N3O2H |
2. Diameter 100–200 nm and length ∼5 μm |
2. Straight cylindrical, tree like and fiber like |
4 |
Boron, NiB/Al2O3 |
1400–1500 |
Diameter 5–40 nm and length several micron |
Bamboo and polar like |
127 |
5 |
1. B and MgO |
1. 1250 and above |
1. Diameter 1-micron |
1. Bulk BN flakes |
81 |
2. B, MgO, FeO |
2. 1100–1700 |
2. Diameter ∼50 nm |
2. Straight and randomly aligned |
6 |
B, MgO, FeO |
1. 1200–1300 |
1. Diameter ∼30 to ∼60 nm |
1. Long straight |
83 |
2. 1300–1400 |
2. Diameters of ∼20 and ∼100 nm |
2. Flower like |
4.4 Importance of catalyst
The use of catalysts is also of great significance in CVD growth of BNNTs. Initially BNNTs were synthesized by using NiB2 as a catalyst. It was found that the catalysts not only accelerated the growth process but also helped in growing long size nanotubes. The size of the catalyst is also very important in BNNTs growth. It works as a pattern during the growth of BNNTs, and the diameter of the final product was found to be in the range of catalyst size.79 In other words, the diameter of the tube can be controlled with the help of choosing an appropriate catalyst.180 BNNTs have also been synthesized without using any catalyst, however the BNNTs thus obtained were smaller in size and lower in quality.162 Pure BNNTs were produced by the introduction of MgO as a catalyst with B. However the growth of the BNNTs was very slow due to the slow catalytic activity of MgO at higher temperature. Therefore, other transition metals and their compounds have also been excessively searched and used as catalysts with B for BNNTs synthesis.102,138,181 It has been proved with a series of experiments that the chemical reaction between boron and these catalysts were not effective enough for a satisfied result.81 FeO reacts with B in the presence of NH3 to produce BNNTs but its results were also not satisfied at higher temperature. In order to have good results that can further be improved at higher temperature, both FeO and MgO were collectively used with B. Thus high quality of BNNTs were obtained with this combination of precursor.81 The use of these catalysts was further improved by using them in different ratios with boron in a conventional set up. For the first time the role of MgO and FeO during the BNNTs synthesis and growth was explained in full detail. It was found that MgO and FeO are not only the effective producer of B2O2 but also of Mg and Fe nanoparticles. B2O2 reacts with NH3 to synthesize BNNTs whereas Mg and Fe helped in the growth of as synthesized BNNTs.84,157 The growth stops only when either these catalysts are fully covered by the precursor's material or cut off as a tip from the BNNTs. Later on, the role and effectiveness of these catalysts were further tried by using them in different weight ratio with boron at different temperatures which resulted in different sizes and morphologies of BNNTs.83 Due to an easy and effective use of MgO and FeO as catalysts with B, they have now become the most effective and conventionally used precursors for BNNTs synthesis till date.155,160
Li2O was also tried as a catalyst with B for BNNTs synthesis due to the superior deoxidation capability of Li compared to Mg. BNNTs thus synthesized were in large quantity. However Li and other species were found in the final product as impurities. Since the experimental set up and steps involved during BNNTs synthesis were a bit complex and time consuming (compared to B, MgO and FeO84),149,150 therefore this catalyst has not been excessively used afterwards. Thus the role of the catalyst is very important in any of CVD technique for BNNTs synthesis. They not only control the size and morphology of BNNTs in CVD techniques but also provides a simple way for the synthesis of other two dimensional (2D) nanostructures of h-BN.
4.5 Importance of growth mechanism
Borazine (B3N3H6) was used to successfully synthesized BNNTs for the first time by CVD technique. Root growth mechanism was assumed to have followed during the growth. The full details of this mechanism have already been given along with a figure in Section 3.1.79 Melamine and boric acid were also used to synthesized BNNTs.162 The growth mechanism followed was similar to the tip growth model of CNTs.182,183 Later on BNNTs were synthesized by different CVD techniques. Regardless of the experimental set up and precursor used, BNNTs were assumed to have grown by tip-growth, base-growth or mix-growth model according to VLS mechanism.80,81,83,84,127,157,169
In VLS mechanism,169 the precursor are heated to a certain temperature. At a suitable temperature, the nanosize catalysts either in liquid or partially melted form condensed on the substrate surface. This is usually occurred when their partial vapor pressures is sufficiently enough for this purpose. The as formed BN species then diffuse into the condense catalyst. Which then aggregates with the passage of time and finally becomes supersaturate that leads to the subsequent precipitation of BNNTs.83
Beside all of the above factors, the role of under lying substrate is also very important in CVD growth of BNNTs. It has further been noted that its presence is helpful in the rational synthesis of BNNTs.184,185 Diameter of the as synthesized materials depends on the grain size and morphology of the substrate. By varying the grain size and morphology of the substrate the diameter of the final product can easily be controlled.186
5 Role of CVD in growth of 2D h-BN and other nanostructures
In the above sections it has been proved that CVD is the most prominent and reliable technique for the synthesis of pure BNNTs. This leads to further study of the same technique with different types of precursors and experimental parameters for the synthesis of 2D h-BN. However, the progress in this regard is very slow as compared BNNTs. The reason is the improved and excellent properties of BNNTs for its potential applications due to its reduced size and nanoscale dimension.
CVD has successfully been employed in the growth of atomic layers of hybridized h-BN and graphene domains187 and large scale synthesis of atomic h-BN layers.188 Ammonia-borane was used as precursor, copper foil as a substrate and Ar/H2 as a reaction atmosphere. The film of atomic h-BN layers (consisted of 2–5 layers) was deposited at the top of the substrate at 1000 °C in a growth duration of 30–60 min. The adopted method for the synthesis was thus suggested to help in studying the structure and properties of h-BN thin film for its potential applications.188 To further facilitate the synthesis of h-BN thin film another simple CVD technique is introduced. This technique work at ambient pressure and thus called ambient pressure CVD (APCVD). In this technique, the precursors (B3N3H6) vapor are carried to the substrate (polycrystalline Ni) with the help of N2 gas flow where they are deposited in the form of thin film (h-BN). This was believed to be the low-cost technique in which the growth temperature can be reduced to 400 °C. However, the growth is followed by annealing at 1000 °C that results in the production of high quality h-BN thin film with thickness in the range of 5–50 nm.189 Though this technique was successful to grow a multilayer (5–50 nm) thin film of h-BN, however, it was unable to control the number of layer to a monolayer and failed to give a specific growth mechanism. These issues have been resolved with the development of low pressure CVD (LPCVD). In this technique Cu foil was used as a substrate and borazane as precursors. Growth in the LPCVD was believed to be surface reaction limited and was hoped to be not affected considerably by the gases flow or substrate surface. However, it was found that the geometry of the substrate has a key role in uniformity of the layers.190 In this regard, a suitable substrate with smooth and fine surface was predicted to be of great importance.190
These progress in CVD synthesis of h-BN thin film and BNNTs shows the potential for further developments in CVD technique with respect to precursor's types, reaction atmosphere, growth duration and experimental set up etc. However, the progress is not restricted only to the synthesis of BNNTs or h-BN thin film. The development in CVD for the synthesis of other nanostructures of h-BN cannot be ignored. Ar-supported TCVD was successfully utilized for the synthesis of BNNWs. In this synthesis the relatively short growth duration was considered to be responsible for the synthesis of BNNWs.73 The concept of Ar-supported TCVD also helped in the successful synthesis of BNMTs. During the synthesis of BNMTs,191 the precursor's type, ratio and experimental set up used were the same as in the synthesis of BNNTs via Ar-supported TCVD,85 however, in the synthesis of BNMTs, NH3 gas flow was used as a reaction atmosphere instead of Ar gas. The same concept191 with an increased growth duration of 90 min (instead of 60 min) was used for the synthesis of BNNS without any further modification.192
All these modifications and progress in CVD techniques suggests that CVD is very versatile technique that can be used for the high quality synthesis of any materials or its compounds by carefully optimizing its experimental parameters. This further provides opportunities for the commercialized production of materials and its use for its potential application.
6 Conclusions
Among all the techniques used for BNNTs synthesis, CVD technique has been proven to produce quality and quantity of BNNTs. The quality and quantity of BNNTs depends on the specific type of CVD technique. In each type of CVD technique, experimental set up, types and ratios of the precursor, temperature, catalysts, substrate and growth mechanism are some of the basic experimental parameters that affect the characteristics of the final product. Therefore, optimization of CVD setup for BNNTs synthesis is still an open area of research. The product obtained so far is not yet suitable (regarding quality and quantity) to be effectively used in any application in the real life.
B, MgO and FeO are the most effective precursors and the conventional horizontal tube furnace with one end closed inner test tube is the most commonly used experimental set up. Changes in the precursors (B, MgO and FeO) dimension and home-made experimental set up with Ar (gas) as an alternative for vacuum or evacuation can assist in successful synthesis of longer and cost effective BNNTs via a more simple TCVD technique. In addition, the idea of re-using the precursors (B, MgO and FeO), for several synthesis and suggested increase in the final product with increasing boat size, it is further suggested that the quality and quantity of BNNTs can be increased to any desired level by modifying the experimental set up in such a way that the synthesized BNNTs cannot block the precursor in the boat. For this purpose the boat should be replaced by a device (like the one used for concrete mixing) of appropriate size and shape that can keep or separate the as form BNNTs layer, and continuously make a homogeneous mixture of the rest of the precursors for further synthesis. This will not only prevent the top of precursor from blocking due to formation of BNNTs layer but also help to continue syntheses of BNNTs till the precursor are completely utilized.
Acknowledgements
We are extremely grateful to University of Malaya, 50603 Kuala Lumpur Malaysia, Project Number: RG375-15AFR, for providing funds and facilities for our research work.
References
- X. Chen, X. Gao, H. Zhang, Z. Zhou, W. Hu, G. Pan, H. Zhu, T. Yan and D. Song, Preparation and electrochemical hydrogen storage of boron nitride nanotubes, J. Phys. Chem. B, 2005, 109, 11525–11529 CrossRef PubMed.
- Y. Zhang, K. Suenaga, C. Colliex and S. Iijima, Coaxial nanocable: silicon carbide and silicon oxide sheathed with boron nitride and carbon, Science, 1998, 281, 973–975 CrossRef.
- S. Chattopadhyay, A. Ganguly, K.-H. Chen and L.-C. Chen, One-dimensional group III-nitrides: growth, properties, and applications in nanosensing and nano-optoelectronics, Crit. Rev. Solid State Mater. Sci., 2009, 34, 224–279 CrossRef.
- A. Rubio, J. L. Corkill and M. L. Cohen, Theory of graphitic boron nitride nanotubes, Phys. Rev. B: Condens. Matter Mater. Phys., 1994, 49, 5081–5084 CrossRef.
- X. Blase, A. Rubio, S. Louie and M. Cohen, Stability and band gap constancy of boron nitride nanotubes, Europhys. Lett., 1994, 28, 335–340 CrossRef.
- N. G. Chopra, R. Luyken, K. Cherrey, V. H. Crespi, M. L. Cohen, S. G. Louie and A. Zettl, Boron nitride nanotubes, Science, 1995, 269, 966–967 CrossRef PubMed.
- B. Akdim, R. Pachter, X. Duan and W. W. Adams, Comparative theoretical study of single-wall carbon and boron-nitride nanotubes, Phys. Rev. B: Condens. Matter Mater. Phys., 2003, 67, 245404–245408 CrossRef.
- Z. H. Dong and Y. Song, Transformations of Cold-Compressed Multiwalled Boron Nitride Nanotubes Probed by Infrared Spectroscopy, J. Phys. Chem. C, 2010, 114, 1782–1788 Search PubMed.
- R. S. Lee, J. Gavillet, M. L. de la Chapelle, A. Loiseau, J. L. Cochon, D. Pigache, J. Thibault and F. Willaime, Catalyst-free synthesis of boron nitride single-wall nanotubes with a preferred zig-zag configuration, Phys. Rev. B: Condens. Matter Mater. Phys., 2001, 64, 121405 CrossRef.
- C. Y. Zhi, Y. Bando, T. Terao, C. C. Tang, H. Kuwahara and D. Golberg, Towards Thermoconductive, Electrically Insulating Polymeric Composites with Boron Nitride Nanotubes as Fillers, Adv. Funct. Mater., 2009, 19, 1857–1862 CrossRef PubMed.
- M. Ishigami, J. D. Sau, S. Aloni, M. L. Cohen and A. Zettl, Observation of the giant stark effect in boron-nitride nanotubes, Phys. Rev. Lett., 2005, 94, 056804 CrossRef.
- W. L. Wang, Y. Bando, C. Y. Zhi, W. Y. Fu, E. G. Wang and D. Golberg, Aqueous noncovalent functionalization and controlled near-surface carbon doping of multiwalled boron nitride nanotubes, J. Am. Chem. Soc., 2008, 130, 8144 CrossRef PubMed.
- M. Ishigami, S. Aloni and A. Zettl, Properties of boron nitride nanotubes, in AIP Conference Proceedings, 2003, pp. 94–99 Search PubMed.
- J. Lauret, R. Arenal, F. Ducastelle, A. Loiseau, M. Cau, B. Attal-Tretout, E. Rosencher and L. Goux-Capes, Optical transitions in single-wall boron nitride nanotubes, Phys. Rev. Lett., 2005, 94, 037405 CrossRef.
- P. Jaffrennou, F. Donatini, J. Barjon, J.-S. Lauret, A. Maguer, B. Attal-Trétout, F. Ducastelle and A. Loiseau, Cathodoluminescence imaging and spectroscopy on a single multiwall boron nitride nanotube, Chem. Phys. Lett., 2007, 442, 372–375 CrossRef PubMed.
- C. Zhi, Y. Bando, C. Tang, D. Golberg, R. Xie and T. Sekigushi, Phonon characteristics and cathodoluminescence of boron nitride nanotubes, Appl. Phys. Lett., 2005, 86, 213110 CrossRef PubMed.
- C. Tang, Y. Bando, C. Zhi and D. Golberg, Boron–oxygen luminescence centres in boron–nitrogen systems, Chem. Commun., 2007, 4599–4601 RSC.
- J. Wu, W.-Q. Han, W. Walukiewicz, J. Ager, W. Shan, E. Haller and A. Zettl, Raman Spectroscopy and Time-Resolved Photoluminescence of BN and BxCyNz Nanotubes, Nano Lett., 2004, 4, 647–650 CrossRef CAS.
- P. Jaffrennou, J. Barjon, T. Schmid, L. Museur, A. Kanaev, J.-S. Lauret, C. Zhi, C. Tang, Y. Bando and D. Golberg, Near-band-edge recombinations in multiwalled boron nitride nanotubes: cathodoluminescence and photoluminescence spectroscopy measurements, Phys. Rev. B: Condens. Matter Mater. Phys., 2008, 77, 235422 CrossRef.
- C. Zhi, Y. Bando, C. Tang and D. Golberg, Boron nitride nanotubes, Mater. Sci. Eng., R, 2010, 70, 92–111 CrossRef PubMed.
- H. Harutyunyan, T. Gokus, A. A. Green, M. C. Hersam, M. Allegrini and A. Hartschuh, Defect-induced photoluminescence from dark excitonic states in individual single-walled carbon nanotubes, Nano Lett., 2009, 9, 2010–2014 CrossRef CAS PubMed.
- S. Lebedkin, K. Arnold, F. Hennrich, R. Krupke, B. Renker and M. M. Kappes, FTIR-luminescence mapping of dispersed single-walled carbon nanotubes, New J. Phys., 2003, 5, 140 CrossRef.
- Y. Murakami, B. Lu, S. Kazaoui, N. Minami, T. Okubo and S. Maruyama, Photoluminescence sidebands of carbon nanotubes below the bright singlet excitonic levels, Phys. Rev. B: Condens. Matter Mater. Phys., 2009, 79, 195407 CrossRef.
- M. J. O'connell, S. M. Bachilo, C. B. Huffman, V. C. Moore, M. S. Strano, E. H. Haroz, K. L. Rialon, P. J. Boul, W. H. Noon and C. Kittrell, Band gap fluorescence from individual single-walled carbon nanotubes, Science, 2002, 297, 593–596 CrossRef PubMed.
- B. Yan, C. Park, J. Ihm, G. Zhou, W. Duan and N. Park, Electron Emission Originated from Free-Electron-like States of Alkali-Doped Boron-Nitride Nanotubes, J. Am. Chem. Soc., 2008, 130, 17012–17015 CrossRef CAS PubMed.
- A. Zettl, C. Chang and G. Begtrup, A new look at thermal properties of nanotubes, Phys. Status Solidi B, 2007, 244, 4181–4183 CrossRef CAS PubMed.
- Y. Chen, J. Zou, S. J. Campbell and G. Le Caer, Boron nitride nanotubes: pronounced resistance to oxidation, Appl. Phys. Lett., 2004, 84, 2430–2432 CrossRef CAS PubMed.
- D. Golberg, Y. Bando, K. Kurashima and T. Sato, Synthesis and characterization of ropes made of BN multiwalled nanotubes, Scr. Mater., 2001, 44, 1561–1565 CrossRef CAS.
- Z. Xu, D. Golberg and Y. Bando, In situ TEM-STM recorded kinetics of boron nitride nanotube failure under current flow, Nano Lett., 2009, 9, 2251–2254 CrossRef CAS PubMed.
- N. Koi, T. Oku, M. Inoue and K. Suganuma, Structures and purification of boron nitride nanotubes synthesized from boron-based powders with iron particles, J. Mater. Sci., 2008, 43, 2955–2961 CrossRef CAS PubMed.
- J. Yu, B. C. Li, J. Zou and Y. Chen, Influence of nitriding gases on the growth of boron nitride nanotubes, J. Mater. Sci., 2007, 42, 4025–4030 CrossRef PubMed.
- L. H. Li, Y. Chen and A. M. Glushenkov, Boron nitride nanotube films grown from boron ink painting, J. Mater. Chem., 2010, 20, 9679–9683 RSC.
- J. Li, G. Zhou, Y. Chen, B. L. Gu and W. H. Duan, Magnetism of C Adatoms on BN Nanostructures: Implications for Functional Nanodevices, J. Am. Chem. Soc., 2009, 131, 1796–1801 CrossRef CAS PubMed.
- R. Wu, L. Liu, G. Peng and Y. Feng, Magnetism in BN nanotubes induced by carbon doping, Appl. Phys. Lett., 2005, 86, 122510 CrossRef PubMed.
- F. Li, Z. Zhu, M. Zhao and Y. Xia, Ab initio calculations on the magnetic properties of hydrogenated boron nitride nanotubes, J. Phys. Chem. C, 2008, 112, 16231–16235 CAS.
- T. Kolodiazhnyi and D. Golberg, Paramagnetic defects in boron nitride nanostructures, Chem. Phys. Lett., 2005, 413, 47–51 CrossRef CAS PubMed.
- T. Oku and M. Kuno, Synthesis, argon/hydrogen storage and magnetic properties of boron nitride nanotubes and nanocapsules, Diamond Relat. Mater., 2003, 12, 840–845 CrossRef CAS.
- S. Hao, G. Zhou, W. Duan, J. Wu and B.-L. Gu, Tremendous spin-splitting effects in open boron nitride nanotubes: application to nanoscale spintronic devices, J. Am. Chem. Soc., 2006, 128, 8453–8458 CrossRef CAS PubMed.
- G. Zhou and W. Duan, Spin-polarized electron current from carbon-doped open armchair boron nitride nanotubes: Implication for nano-spintronic devices, Chem. Phys. Lett., 2007, 437, 83–86 CrossRef CAS PubMed.
- K.-S. Ryu, Y.-I. Kim, J. K. Jung, Y. Chen and C. H. Lee, Dynamics of Natural Spin Defects in Boron Nitride Nanotubes, J. Nanosci. Nanotechnol., 2008, 8, 5193–5195 CrossRef CAS PubMed.
- Z. H. Zhang and W. L. Guo, Tunable Ferromagnetic Spin Ordering in Boron Nitride Nanotubes with Topological Fluorine Adsorption, J. Am. Chem. Soc., 2009, 131, 6874–6879 CrossRef CAS PubMed.
- Z. Zhou, J. Zhao, Z. Chen and P. V. R. Schleyer, Atomic and electronic structures of fluorinated BN nanotubes: computational study, J. Phys. Chem. B, 2006, 110, 25678–25685 CrossRef CAS PubMed.
- C. Tang, Y. Bando, T. Sato and K. Kurashima, Uniform boron nitride coatings on silicon carbide nanowires, Adv. Mater., 2002, 14, 1046–1049 CrossRef CAS.
- W. S. Jang, S. Y. Kim, J. Lee, J. Park, C. J. Park and C. J. Lee, Triangular GaN–BN core–shell nanocables: synthesis and field emission, Chem. Phys. Lett., 2006, 422, 41–45 CrossRef CAS PubMed.
- L. W. Yin, Y. Bando, Y. C. Zhu, D. Golberg and M. S. Li, A Two-Stage Route to Coaxial Cubic–Aluminum–Nitride–Boron–Nitride Composite Nanotubes, Adv. Mater., 2004, 16, 929–933 CrossRef CAS PubMed.
- Y.-C. Zhu, Y. Bando, D.-F. Xue, F.-F. Xu and D. Golberg, Insulating tubular BN sheathing on semiconducting nanowires, J. Am. Chem. Soc., 2003, 125, 14226–14227 CrossRef CAS PubMed.
- K. Suenaga, C. Colliex, N. Demoncy, A. Loiseau, H. Pascard and F. Willaime, Synthesis of nanoparticles and nanotubes with well-separated layers of boron nitride and carbon, Science, 1997, 278, 653–655 CrossRef CAS.
- N. P. Bansal, J. B. Hurst and S. R. Choi, Boron Nitride Nanotubes-Reinforced Glass Composites, J. Am. Ceram. Soc., 2006, 89, 388–390 CrossRef CAS PubMed.
- Q. Huang, Y. Bando, X. Xu, T. Nishimura, C. Zhi, C. Tang, F. Xu, L. Gao and D. Golberg, Enhancing superplasticity of engineering ceramics by introducing BN nanotubes, Nanotechnology, 2007, 18, 485706 CrossRef.
- A. Leela Mohana Reddy, A. E. Tanur and G. C. Walker, Synthesis and hydrogen storage properties of different types of boron nitride nanostructures, Int. J. Hydrogen Energy, 2010, 35, 4138–4143 CrossRef PubMed.
- G. Mpourmpakis and G. E. Froudakis, Why boron nitride nanotubes are preferable to carbon nanotubes for hydrogen storage?: An ab initio theoretical study, Catal. Today, 2007, 120, 341–345 CrossRef CAS PubMed.
- S. Hu, E.-J. Kan and J. Yang, First-principles study of interaction between H molecules and BN nanotubes with BN divacancies, J. Chem. Phys., 2007, 127, 164718 CrossRef PubMed.
- S. Shevlin and Z. Guo, Hydrogen sorption in defective hexagonal BN sheets and BN nanotubes, Phys. Rev. B: Condens. Matter Mater. Phys., 2007, 76, 024104 CrossRef.
- E. Durgun, Y.-R. Jang and S. Ciraci, Hydrogen storage capacity of Ti-doped boron-nitride and B/Be-substituted carbon nanotubes, Phys. Rev. B: Condens. Matter Mater. Phys., 2007, 76, 073413 CrossRef.
- B.-C. Wang, M.-H. Tsai and Y.-M. Chou, Comparative theoretical study of carbon nanotubes and boron-nitride nanotubes, Synth. Met., 1997, 86, 2379–2380 CrossRef CAS.
- T. Oku, N. Koi and K. Suganuma, Electronic and optical properties of boron nitride nanotubes, J. Phys. Chem. Solids, 2008, 69, 1228–1231 CrossRef CAS PubMed.
- H. Chen, Y. Chen, C. P. Li, H. Zhang, J. S. Williams, Y. Liu, Z. Liu and S. P. Ringer, Eu-doped Boron Nitride Nanotubes as a Nanometer Sized Visible Light Source, Adv. Mater., 2007, 19, 1845–1848 CrossRef CAS PubMed.
- B. Berzina, L. Trinkler, V. Korsak, R. Krutohvostov, D. Carroll, K. Ucer and R. Williams, Exciton luminescence of boron nitride nanotubes and nano arches, Phys. Status Solidi B, 2006, 243, 3840–3845 CrossRef CAS PubMed.
- W.-Q. Han, H.-G. Yu, C. Zhi, J. Wang, Z. Liu, T. Sekiguchi and Y. Bando, Isotope effect on band gap and radiative transitions properties of boron nitride nanotubes, Nano Lett., 2008, 8, 491–494 CrossRef CAS PubMed.
- H. Chen, Y. Chen, Y. Liu, C.-N. Xu and J. S. Williams, Light emission and excitonic effect of boron nitride nanotubes observed by photoluminescent spectra, Opt. Mater., 2007, 29, 1295–1298 CrossRef CAS PubMed.
- M. Silly, P. Jaffrennou, J. Barjon, J.-S. Lauret, F. Ducastelle, A. Loiseau, E. Obraztsova, B. Attal-Tretout and E. Rosencher, Luminescence properties of hexagonal boron nitride: cathodoluminescence and photoluminescence spectroscopy measurements, Phys. Rev. B: Condens. Matter Mater. Phys., 2007, 75, 085205 CrossRef.
- R. B. Chen, F. L. Shyu, C. P. Chang and M. F. Lin, Optical properties of boron nitride nanotubes, J. Phys. Soc. Jpn., 2002, 71, 2286–2289 CrossRef CAS.
- P. Jaffrennou, J. Barjon, J. S. Lauret, A. Maguer, D. Golberg, B. Attal Trétout, F. Ducastelle and A. Loiseau, Optical properties of multiwall boron nitride nanotubes, Phys. Status Solidi B, 2007, 244, 4147–4151 CrossRef CAS PubMed.
- B. Berzina, L. Trinkler, R. Krutohvostov, R. Williams, D. Carroll, R. Czerw and E. Shishonok, Photoluminescence excitation spectroscopy in boron nitride nanotubes compared to microcrystalline h-BN and c-BN, Phys. Status Solidi C, 2005, 2, 318–321 CrossRef CAS PubMed.
- G. Ciofani, V. Raffa, A. Menciassi and A. Cuschieri, Cytocompatibility, interactions, and uptake of polyethyleneimine coated boron nitride nanotubes by living cells: confirmation of their potential for biomedical applications, Biotechnol. Bioeng., 2008, 101, 850–858 CrossRef CAS PubMed.
- G. Ciofani, V. Raffa, A. Menciassi and P. Dario, Preparation of boron nitride nanotubes aqueous dispersions for biological applications, J. Nanosci. Nanotechnol., 2008, 8, 6223–6231 CrossRef CAS PubMed.
- G. Ciofani, V. Raffa, A. Menciassi and A. Cuschieri, Boron nitride nanotubes: an innovative tool for nanomedicine, Nano Today, 2009, 4, 8–10 CrossRef CAS PubMed.
- G. Ciofani, V. Raffa, J. Yu, Y. Chen, Y. Obata, S. Takeoka, A. Menciassi and A. Cuschieri, Boron nitride nanotubes: a novel vector for targeted magnetic drug delivery, Curr. Nanosci., 2009, 5, 33–38 CrossRef CAS.
- X. Chen, P. Wu, M. Rousseas, D. Okawa, Z. Gartner, A. Zettl and C. R. Bertozzi, Boron Nitride Nanotubes are Noncytotoxic and can be Functionalized for Interaction with Proteins and Cells, J. Am. Chem. Soc., 2009, 131, 890–891 CrossRef CAS PubMed.
- G. Ciofani, S. Danti, D. D'Alessandro, L. Ricotti, S. Moscato, G. Bertoni, A. Falqui, S. Berrettini, M. Petrini and V. Mattoli, Enhancement of neurite outgrowth in neuronal-like cells following boron nitride nanotube-mediated stimulation, ACS Nano, 2010, 4, 6267–6277 CrossRef CAS PubMed.
- J. Wang, C. H. Lee and Y. K. Yap, Recent advancements in boron nitride nanotubes, Nanoscale, 2010, 2, 2028–2034 RSC.
- M. Liao and Y. Koide, Carbon-based materials: growth, properties, MEMS/NEMS technologies, and MEM/NEM switches, Crit. Rev. Solid State Mater. Sci., 2011, 36, 66–101 CrossRef CAS.
- P. Ahmad, M. U. Khandaker, Z. R. Khan and Y. M. Amin, A simple technique to synthesize pure and highly crystalline boron nitride nanowires, Ceram. Int., 2014, 40, 14727–14732 CrossRef CAS PubMed.
- J. Li, R. Dahal, S. Majety, J. Lin and H. Jiang, Hexagonal boron nitride epitaxial layers as neutron detector materials, Nucl. Instrum. Methods Phys. Res., Sect. A, 2011, 654, 417–420 CrossRef CAS PubMed.
- P. Ahmad, M. U. Khandaker and Y. M. Amin, Synthesis of highly crystalline multilayers structures of 10BNNTs as a potential neutron sensing element, Ceram. Int., 2015, 41, 4544–4548 CrossRef CAS PubMed.
- P. Ahmad, N. M. Mohamed and Z. A. Burhanudin, A review of nanostructured based radiation sensors for neutron, in AIP Conference Proceedings, 2012, p. 535 Search PubMed.
- A. M. Cassell, J. A. Raymakers, J. Kong and H. Dai, Large scale CVD synthesis of single-walled carbon nanotubes, J. Phys. Chem. B, 1999, 103, 6484–6492 CrossRef CAS.
- C. J. Lee, D. W. Kim, T. J. Lee, Y. C. Choi, Y. S. Park, Y. H. Lee, W. B. Choi, N. S. Lee, G.-S. Park and J. M. Kim, Synthesis of aligned carbon nanotubes using thermal chemical vapor deposition, Chem. Phys. Lett., 1999, 312, 461–468 CrossRef CAS.
- O. R. Lourie, C. R. Jones, B. M. Bartlett, P. C. Gibbons, R. S. Ruoff and W. E. Buhro, CVD growth of boron nitride nanotubes, Chem. Mater., 2000, 12, 1808–1810 CrossRef CAS.
- C. Tang, Y. Bando, T. Sato and K. Kurashima, A novel precursor for synthesis of pure boron nitride nanotubes, Chem. Commun., 2002, 1290–1291 RSC.
- C. Zhi, Y. Bando, C. Tan and D. Golberg, Effective precursor for high yield synthesis of pure BN nanotubes, Solid State Commun., 2005, 135, 67–70 CrossRef CAS PubMed.
- R. S. Wagner and W. C. Ellis, Vapor Liquid Solid Mechanism of Single Crystal Growth, Appl. Phys. Lett., 1964, 4, 89–90 CrossRef CAS PubMed.
- A. Pakdel, C. Zhi, Y. Bando, T. Nakayama and D. Golberg, A comprehensive analysis of the CVD growth of boron nitride nanotubes, Nanotechnology, 2012, 23, 215601 CrossRef PubMed.
- C. H. Lee, J. Wang, V. K. Kayatsha, J. Y. Huang and Y. K. Yap, Effective growth of boron nitride nanotubes by thermal chemical vapor deposition, Nanotechnology, 2008, 19, 455605 CrossRef PubMed.
- P. Ahmad, M. U. Khandaker and Y. M. Amin, Synthesis of boron nitride nanotubes by argon supported thermal chemical vapor deposition, Phys. E, 2015, 67, 33–37 CrossRef CAS PubMed.
- I. Narita and T. Oku, Synthesis of boron nitride nanotubes by using YB6 powder, Solid State Commun., 2002, 122, 465–468 CrossRef CAS.
- A. Loiseau, F. Willaime, N. Demoncy, G. Hug and H. Pascard, Boron nitride nanotubes with reduced numbers of layers synthesized by arc discharge, Phys. Rev. Lett., 1996, 76, 4737 CrossRef CAS.
- A. Loiseau, F. Willaime, N. Demoncy, N. Schramchenko, G. Hug, C. Colliex and H. Pascard, Boron nitride nanotubes, Carbon, 1998, 36, 743–752 CrossRef CAS.
- J. Cumings and A. Zettl, Mass-production of boron nitride double-wall nanotubes and nanococoons, Chem. Phys. Lett., 2000, 316, 211–216 CrossRef CAS.
- D. Goldberg, Y. Bando, M. Eremets, K. Takemura, K. Kurashima, K. Tamiya and H. Yusa, Boron nitride nanotube growth defects and their annealing-out under electron irradiation, Chem. Phys. Lett., 1997, 279, 191–196 CrossRef.
- R. Arenal, O. Stephan, J. L. Cochon and A. Loiseau, Root-growth mechanism for single-walled boron nitride nanotubes in laser vaporization technique, J. Am. Chem. Soc., 2007, 129, 16183–16189 CrossRef CAS PubMed.
- D. Golberg, A. Rode, Y. Bando, M. Mitome, E. Gamaly and B. Luther-Davies, Boron nitride nanostructures formed by ultra-high-repetition rate laser ablation, Diamond Relat. Mater., 2003, 12, 1269–1274 CrossRef CAS.
- D. Golberg, Y. Bando, M. Eremets, K. Takemura, K. Kurashima and H. Yusa, Nanotubes in boron nitride laser heated at high pressure, Appl. Phys. Lett., 1996, 69, 2045–2047 CrossRef CAS PubMed.
- G. W. Zhou, Z. Zhang, Z. G. Bai and D. P. Yu, Catalyst effects on formation of boron nitride nano-tubules synthesized by laser ablation, Solid State Commun., 1999, 109, 555–559 CrossRef CAS.
- D. Golberg, Y. Bando, M. Eremets, K. Kurashima, T. Tamiya, K. Takemura and H. Yusa, High-resolution analytical electron microscopy of boron nitrides laser heated at high pressure, J. Electron Microsc., 1997, 46, 281–292 CrossRef CAS.
- M. Cau, N. Dorval, B. Attal-Trétout, J. Cochon, B. Cao, L. Bresson, P. Jaffrennou, M. Silly, A. Loiseau and E. Obraztsova, LASER-based diagnostics applied to the study of BN nanotubes synthesis, J. Nanosci. Nanotechnol., 2008, 8, 6129–6140 CrossRef CAS PubMed.
- S. Komatsu, Y. Shimizu, Y. Moriyoshi, K. Okada and M. Mitomo, Preparation of boron nitride nanocapsules by plasma-assisted pulsed laser deposition, J. Appl. Phys., 2002, 91, 6181–6184 CrossRef CAS PubMed.
- V. Naumov, F. Kosyrev, V. Vostrikov, N. Arutyunyan, E. Obraztsova, V. Konov, H. Jiang, A. Nasibulin and E. Kauppinen, Synthesis of boron nitride multi-walled nanotubes by laser ablation technique, Laser Phys., 2009, 19, 1198–1200 CrossRef CAS.
- M. W. Smith, K. C. Jordan, C. Park, J.-W. Kim, P. T. Lillehei, R. Crooks and J. S. Harrison, Very long single-and few-walled boron nitride nanotubes via the pressurized vapor/condenser method, Nanotechnology, 2009, 20, 505604 CrossRef PubMed.
- Y. Wang, Y. Yamamoto, H. Kiyono and S. Shimada, Highly ordered boron nitride nanotube arrays with controllable texture from ammonia borane by template-aided vapor-phase pyrolysis, J. Nanomater., 2008, 2008, 72 Search PubMed.
- D. Golberg, Y. Bando, M. Mitome, K. Kurashima, T. Sato, N. Grobert, M. Reyes-Reyes, H. Terrones and M. Terrones, Preparation of aligned multi-walled BN and B/C/N nanotubular arrays and their characterization using HRTEM, EELS and energy-filtered TEM, Phys. B, 2002, 323, 60–66 CrossRef CAS.
- D. Golberg, Y. Bando, K. Kurashima and T. Sato, Ropes of BN multi-walled nanotubes, Solid State Commun., 2000, 116, 1–6 CrossRef CAS.
- W. Han, P. Redlich, F. Ernst and M. Rühle, Synthesizing boron nitride nanotubes filled with SiC nanowires by using carbon nanotubes as templates, Appl. Phys. Lett., 1999, 75, 1875–1877 CrossRef CAS PubMed.
- X. Wang, Q. Wu, Z. Hu and Y. Chen, Template-directed synthesis of boron nitride nanotube arrays by microwave plasma chemical reaction, Electrochim. Acta, 2007, 52, 2841–2844 CrossRef CAS PubMed.
- W.-Q. Han, W. Mickelson, J. Cumings and A. Zettl, Transformation of BxCyNz nanotubes to pure BN nanotubes, Appl. Phys. Lett., 2002, 81, 1110–1112 CrossRef CAS PubMed.
- K. B. Shelimov and M. Moskovits, Composite nanostructures based on template-grown boron nitride nanotubules, Chem. Mater., 2000, 12, 250–254 CrossRef CAS.
- Y. Wang, S. Shimada, Y. Yamamoto and N. Miyaura, Preparation of h-BN nano-tubes, -bamboos, and-fibers from borazine oligomer with alumina porous template, Mater. Res. Bull., 2008, 43, 251–256 CrossRef CAS PubMed.
- M. Bechelany, S. Bernard, A. Brioude, D. Cornu, P. Stadelmann, C. Charcosset, K. Fiaty and P. Miele, Synthesis of boron nitride nanotubes by a template-assisted polymer thermolysis process, J. Phys. Chem. C, 2007, 111, 13378–13384 CAS.
- W. Han, Y. Bando, K. Kurashima and T. Sato, Synthesis of boron nitride nanotubes from carbon nanotubes by a substitution reaction, Appl. Phys. Lett., 1998, 73, 3085 CrossRef CAS PubMed.
- F. L. Deepak, C. Vinod, K. Mukhopadhyay, A. Govindaraj and C. Rao, Boron nitride nanotubes and nanowires, Chem. Phys. Lett., 2002, 353, 345–352 CrossRef CAS.
- W. Q. Han, P. J. Todd and M. Strongin, Formation and growth mechanism of BN nanotubes via a carbon nanotube-substitution reaction, Appl. Phys. Lett., 2006, 89, 173103 CrossRef PubMed.
- J. Q. Bi, W. L. Wang, Y. X. Qi, Y. J. Bai, L. L. Pang, H. L. Zhu, Y. Zhao and Y. Wang, Large-scale synthesis of BN nanotubes using carbon nanotubes as template, Mater. Lett., 2009, 63, 1299–1302 CrossRef CAS PubMed.
- D. Golberg, Y. Bando, K. Kurashima and T. Sato, MoO3-promoted synthesis of multi-walled BN nanotubes from C nanotube templates, Chem. Phys. Lett., 2000, 323, 185–191 CrossRef CAS.
- J. Dai, L. Xu, Z. Fang, D. Sheng, Q. Guo, Z. Ren, K. Wang and Y. Qian, A convenient catalytic approach to synthesize straight boron nitride nanotubes using synergic nitrogen source, Chem. Phys. Lett., 2007, 440, 253–258 CrossRef CAS PubMed.
- G. Rosas, J. Sistos, J. Ascencio, A. Medina and R. Pérez, Múltiple-walled BN nanotubes obtained with a mechanical alloying technique, Appl. Phys. A: Mater. Sci. Process., 2005, 80, 377–380 CrossRef CAS PubMed.
- L. Xu, Y. Peng, Z. Meng, W. Yu, S. Zhang, X. Liu and Y. Qian, A co-pyrolysis method to boron nitride nanotubes at relative low temperature, Chem. Mater., 2003, 15, 2675–2680 CrossRef CAS.
- P. Cai, L. Chen, L. Shi, Z. Yang, A. Zhao, Y. Gu, T. Huang and Y. Qian, One convenient synthesis route to boron nitride nanotube, Solid State Commun., 2005, 133, 621–623 CrossRef CAS PubMed.
- Y. Chen, L. T. Chadderton, J. F. Gerald and J. S. Williams, A solid-state process for formation of boron nitride nanotubes, Appl. Phys. Lett., 1999, 74, 2960–2962 CrossRef CAS PubMed.
- J. Yu, Y. Chen, R. Wuhrer, Z. Liu and P. Simon, In situ formation of BN nanotubes during nitriding reactions, Chem. Mater., 2005, 17, 5172–5176 CrossRef CAS.
- Y. Chen, M. Conway, J. Williams and J. Zou, Large-quantity production of high-yield boron nitride nanotubes, J. Mater. Res., 2002, 17, 1896–1899 CrossRef CAS.
- H. Chen, Y. Chen, Y. Liu, L. Fu, C. Huang and D. Llewellyn, Over 1.0 mm-long boron nitride nanotubes, Chem. Phys. Lett., 2008, 463, 130–133 CrossRef CAS PubMed.
- S. Singhal, A. Srivastava, R. Pant, S. Halder, B. Singh and A. K. Gupta, Synthesis of boron nitride nanotubes employing mechanothermal process and its characterization, J. Mater. Sci., 2008, 43, 5243–5250 CrossRef CAS PubMed.
- L. Li, C. P. Li and Y. Chen, Synthesis of boron nitride nanotubes, bamboos and nanowires, Phys. E, 2008, 40, 2513–2516 CrossRef CAS PubMed.
- S. Yong Bae, H. Won Seo, J. Park, Y. Sang Choi, J. Chul Park and S. Young Lee, Boron nitride nanotubes synthesized in the temperature range 1000–1200 °C, Chem. Phys. Lett., 2003, 374, 534–541 CrossRef.
- Y. Chen, J. Fitz Gerald, J. Williams and S. Bulcock, Synthesis of boron nitride nanotubes at low temperatures using reactive ball milling, Chem. Phys. Lett., 1999, 299, 260–264 CrossRef CAS.
- T. Terao, Y. Bando, M. Mitome, K. Kurashima, C. Zhi, C. Tang and D. Golberg, Effective synthesis of surface-modified boron nitride nanotubes and related nanostructures and their hydrogen uptake, Phys. E, 2008, 40, 2551–2555 CrossRef CAS PubMed.
- C. Tang, Y. Bando and T. Sato, Catalytic growth of boron nitride nanotubes, Chem. Phys. Lett., 2002, 362, 185–189 CrossRef CAS.
- M. J. Kim, S. Chatterjee, S. M. Kim, E. A. Stach, M. G. Bradley, M. J. Pender, L. G. Sneddon and B. Maruyama, Double-walled boron nitride nanotubes grown by floating catalyst chemical vapor deposition, Nano Lett., 2008, 8, 3298–3302 CrossRef CAS PubMed.
- C. Tang, X. Ding, X. Huang, Z. Gan, S. Qi, W. Liu and S. Fan, Effective growth of boron nitride nanotubes, Chem. Phys. Lett., 2002, 356, 254–258 CrossRef CAS.
- Z. Gan, X. Ding, Z. Huang, X. Huang, C. Cheng, C. Tang and S. Qi, Growth of boron nitride nanotube film in situ, Appl. Phys. A: Mater. Sci. Process., 2005, 81, 527–529 CrossRef CAS PubMed.
- J. Wang, V. K. Kayastha, Y. K. Yap, Z. Fan, J. G. Lu, Z. Pan, I. N. Ivanov, A. A. Puretzky and D. B. Geohegan, Low temperature growth of boron nitride nanotubes on substrates, Nano Lett., 2005, 5, 2528–2532 CrossRef CAS PubMed.
- C. Tang, Y. Bando and D. Golberg, Multi-walled BN nanotubes synthesized by carbon-free method, J. Solid State Chem., 2004, 177, 2670–2674 CrossRef CAS PubMed.
- M. Bechelany, A. Brioude, P. Stadelmann, S. Bernard, D. Cornu and P. Miele, Preparation of BN microtubes/nanotubes with a unique chemical process, J. Phys. Chem. C, 2008, 112, 18325–18330 CAS.
- M. Terauchi, M. Tanaka, K. Suzuki, A. Ogino and K. Kimura, Production of zigzag-type BN nanotubes and BN cones by thermal annealing, Chem. Phys. Lett., 2000, 324, 359–364 CrossRef CAS.
- L. Guo and R. Singh, Selective growth of boron nitride nanotubes by plasma-enhanced chemical vapor deposition at low substrate temperature, Nanotechnology, 2008, 19, 065601 CrossRef CAS PubMed.
- R. Sen, B. Satishkumar, A. Govindaraj, K. Harikumar, G. Raina, J.-P. Zhang, A. Cheetham and C. Rao, B–C–N, C–N and B–N nanotubes produced by the pyrolysis of precursor molecules over Co catalysts, Chem. Phys. Lett., 1998, 287, 671–676 CrossRef CAS.
- L. Cao, X. Zhang, H. Tian, Z. Zhang and W. Wang, Boron nitride nanotube branched nanojunctions, Nanotechnology, 2007, 18, 155605 CrossRef.
- T. Oku, N. Koi, K. Suganuma, R. V. Belosludov and Y. Kawazoe, Formation and atomic structure of boron nitride nanotubes with a cup-stacked structure, Solid State Commun., 2007, 143, 331–336 CrossRef CAS PubMed.
- T. Oku, N. Koi and K. Suganuma, Synthesis and nanostructure of boron nitride nanotubes grown from iron-evaporated boron, Diamond Relat. Mater., 2008, 17, 1805–1807 CrossRef CAS PubMed.
- J. Fu, Y. Lu, H. Xu, K. Huo, X. Wang, L. Li, Z. Hu and Y. Chen, The synthesis of boron nitride nanotubes by an extended vapour–liquid–solid method, Nanotechnology, 2004, 15, 727 CrossRef CAS.
- C. Tang, Y. Bando, X. Ding, S. Qi and D. Golberg, Catalyzed collapse and enhanced hydrogen storage of BN nanotubes, J. Am. Chem. Soc., 2002, 124, 14550–14551 CrossRef CAS PubMed.
- A. Ganguly, S. Chattopadhyay, K.-H. Chen and L.-C. Chen, Production and Storage of Energy with One-Dimensional Semiconductor Nanostructures, Crit. Rev. Solid State Mater. Sci., 2014, 39, 109–153 CrossRef CAS.
- J. Kong, A. M. Cassell and H. Dai, Chemical vapor deposition of methane for single-walled carbon nanotubes, Chem. Phys. Lett., 1998, 292, 567–574 CrossRef CAS.
- M. Su, B. Zheng and J. Liu, A scalable CVD method for the synthesis of single-walled carbon nanotubes with high catalyst productivity, Chem. Phys. Lett., 2000, 322, 321–326 CrossRef CAS.
- E. Couteau, K. Hernadi, J. W. Seo, L. Thien-Nga, C. Mikó, R. Gaal and L. Forro, CVD synthesis of high-purity multiwalled carbon nanotubes using CaCO3 catalyst support for large-scale production, Chem. Phys. Lett., 2003, 378, 9–17 CrossRef CAS.
- K.-E. Kim, K.-J. Kim, W. S. Jung, S. Y. Bae, J. Park, J. Choi and J. Choo, Investigation on the temperature-dependent growth rate of carbon nanotubes using chemical vapor deposition of ferrocene and acetylene, Chem. Phys. Lett., 2005, 401, 459–464 CrossRef CAS PubMed.
- C. Tang, M. Lamy de la Chapelle, P. Li, Y. Liu, H. Dang and S. Fan, Catalytic growth of nanotube and nanobamboo structures of boron nitride, Chem. Phys. Lett., 2001, 342, 492–496 CrossRef CAS.
- D. Golberg, Y. Bando, C. Tang and C. Zhi, Functional boron nitride nanotubes, in Nanoelectronics Conference (INEC), 2010 3rd International, IEEE, 2010, pp. 47–48 Search PubMed.
- Y. Huang, J. Lin, C. Tang, Y. Bando, C. Zhi, T. Zhai, B. Dierre, T. Sekiguchi and D. Golberg, Bulk synthesis, growth mechanism and properties of highly pure ultrafine boron nitride nanotubes with diameters of sub-10 nm, Nanotechnology, 2011, 22, 145602 CrossRef PubMed.
- L. Li, X. Liu, L. H. Li and Y. Chen, High yield BNNTs synthesis by promotion effect of milling-assisted precursor, Microelectron. Eng., 2013, 110, 256–259 CrossRef CAS PubMed.
- C. Zhi, Y. Bando, C. Tang, S. Honda, H. Kuwahara and D. Golberg, Boron nitride nanotubes/polystyrene composites, J. Mater. Res., 2006, 21, 2794–2800 CrossRef CAS.
- M. Yamaguchi, D.-M. Tang, C. Zhi, Y. Bando, D. Shtansky and D. Golberg, Synthesis, structural analysis and in situ transmission electron microscopy mechanical tests on individual aluminum matrix/boron nitride nanotube nanohybrids, Acta Mater., 2012, 60, 6213–6222 CrossRef CAS PubMed.
- D. Golberg, P. M. Costa, O. Lourie, M. Mitome, X. Bai, K. Kurashima, C. Zhi, C. Tang and Y. Bando, Direct force measurements and kinking under elastic deformation of individual multiwalled boron nitride nanotubes, Nano Lett., 2007, 7, 2146–2151 CrossRef CAS.
- X. Wei, M. S. Wang, Y. Bando and D. Golberg, Tensile Tests on Individual Multi-Walled Boron Nitride Nanotubes, Adv. Mater., 2010, 22, 4895–4899 CrossRef CAS PubMed.
- M. Yamaguchi, A. Pakdel, C. Zhi, Y. Bando, D.-M. Tang, K. Faerstein, D. Shtansky and D. Golberg, Utilization of multiwalled boron nitride nanotubes for the reinforcement of lightweight aluminum ribbons, Nanoscale Res. Lett., 2013, 8, 1–6 CrossRef PubMed.
- V. K. Kayastha, S. Wu, J. Moscatello and Y. K. Yap, Synthesis of Vertically Aligned Single- and Double-Walled Carbon Nanotubes without Etching Agents, J. Phys. Chem. C, 2007, 111, 10158–10161 CAS.
- C. H. Lee, M. Xie, V. Kayastha, J. Wang and Y. K. Yap, Patterned growth of boron nitride nanotubes by catalytic chemical vapor deposition, Chem. Mater., 2010, 22, 1782–1787 CrossRef CAS.
- C. J. Lee, J. H. Park and J. Park, Synthesis of bamboo-shaped multiwalled carbon nanotubes using thermal chemical vapor deposition, Chem. Phys. Lett., 2000, 323, 560–565 CrossRef CAS.
- T. H. Ferreira, P. R. O. da Silva, R. G. dos Santos and E. M. B. de Sousa, A novel synthesis route to produce boron nitride nanotubes for bioapplications, J. Biomater. Nanobiotechnol., 2011, 2, 426–434 CrossRef CAS.
- D. Seo, J. Kim, S.-H. Park, Y.-U. Jeong, Y.-S. Seo, S.-H. Lee and J. Kim, Synthesis of boron nitride nanotubes using thermal chemical vapor deposition of ball milled boron powder, J. Ind. Eng. Chem., 2012, 19, 1117–1122 CrossRef PubMed.
- D. Özmen, N. A. Sezgi and S. Balcı, Synthesis of Boron Nitride Nanotubes from Ammonia & a Powder Mixture of Boron & Iron Oxide, Chem. Eng. J., 2012, 219, 28–36 CrossRef PubMed.
- R. Ma, Y. Bando and T. Sato, CVD synthesis of boron nitride nanotubes without metal catalysts, Chem. Phys. Lett., 2001, 337, 61–64 CrossRef CAS.
- R. Ma, Y. Bando, T. Sato and K. Kurashima, Thin boron nitride nanotubes with unusual large inner diameters, Chem. Phys. Lett., 2001, 350, 434–440 CrossRef CAS.
- R. Ma, Y. Bando, T. Sato and L. Bourgeois, Novel BN tassel-like and tree-like nanostructures, Diamond Relat. Mater., 2002, 11, 1397–1402 CrossRef CAS.
- C. Tang, Y. Bando, Y. Huang, S. Yue, C. Gu, F. Xu and D. Golberg, Fluorination and electrical conductivity of BN nanotubes, J. Am. Chem. Soc., 2005, 127, 6552–6553 CrossRef CAS PubMed.
- D. Golberg, Y. Bando, C. Tang and C. Zhi, Boron nitride nanotubes, Adv. Mater., 2007, 19, 2413–2432 CrossRef CAS PubMed.
- L. Guo and R. Singh, Catalytic growth of boron nitride nanotubes using gas precursors, Phys. E, 2009, 41, 448–453 CrossRef CAS PubMed.
- C.-Y. Su, W.-Y. Chu, Z.-Y. Juang, K.-F. Chen, B.-M. Cheng, F.-R. Chen, K.-C. Leou and C.-H. Tsai, Large-scale synthesis of boron nitride nanotubes with iron-supported catalysts, J. Phys. Chem. C, 2009, 113, 14732–14738 CAS.
- R. Wagner and W. Ellis, Vapor liquid solid mechanism of single crystal growth, Appl. Phys. Lett., 1964, 4, 89–90 CrossRef CAS PubMed.
- C. Tang, Y. Bando and T. Sato, Synthesis and morphology of boron nitride nanotubes and nanohorns, Appl. Phys. A: Mater. Sci. Process., 2002, 75, 681–685 CrossRef CAS PubMed.
- C. Y. Zhi, Y. Bando, C. C. Tang, R. G. Xie, T. Sekiguchi and D. Golberg, Perfectly dissolved boron nitride nanotubes due to polymer wrapping, J. Am. Chem. Soc., 2005, 127, 15996–15997 CrossRef CAS PubMed.
- C. Zhi, Y. Bando, C. Tang, S. Honda, K. Sato, H. Kuwahara and D. Golberg, Covalent Functionalization: Towards Soluble Multiwalled Boron Nitride Nanotubes, Angew. Chem., Int. Ed., 2005, 44, 7932–7935 CrossRef CAS PubMed.
- C. Y. Zhi, Y. Bando, C. C. Tang, S. Honda, K. Sato, H. Kuwahara and D. Golberg, Purification of boron nitride nanotubes through polymer wrapping, J. Phys. Chem. B, 2006, 110, 1525–1528 CrossRef CAS PubMed.
- C. Zhi, Y. Bando, C. Tang, D. Golberg, R. Xie and T. Sekiguchi, Large-scale fabrication of boron nitride nanohorn, Appl. Phys. Lett., 2005, 87, 063107 CrossRef PubMed.
- W. Meng, Y. Huang, Y. Fu, Z. Wang and C. Zhi, Polymer composites of boron nitride nanotubes and nanosheets, J. Mater. Chem. C, 2014, 2, 10049–10061 RSC.
- B. Saner Okan, Z. L. O. Z. Kocabaş, A. Nalbant Ergün, M. Baysal, I. Letofsky-Papst and Y. Yürüm, Effect of Reaction Temperature and Catalyst Type on the Formation of Boron Nitride Nanotubes by Chemical Vapor Deposition and Measurement of their Hydrogen Storage Capacity, Ind. Eng. Chem. Res., 2012, 51, 11341–11347 CrossRef CAS.
- R. Ma, Y. Bando, T. Sato and K. Kurashima, Growth, morphology, and structure of boron nitride nanotubes, Chem. Mater., 2001, 13, 2965–2971 CrossRef CAS.
- L. Bourgeois, Y. Bando and T. Sato, Tubes of rhombohedral boron nitride, J. Phys. D: Appl. Phys., 2000, 33, 1902 CrossRef CAS.
- T. Ishii, T. Sato, Y. Sekikawa and M. Iwata, Growth of whiskers of hexagonal boron nitride, J. Cryst. Growth, 1981, 52, 285–289 CrossRef CAS.
- I. Willems, Z. Konya, J.-F. Colomer, G. Van Tendeloo, N. Nagaraju, A. Fonseca and J. Nagy, Control of the outer diameter of thin carbon nanotubes synthesized by catalytic decomposition of hydrocarbons, Chem. Phys. Lett., 2000, 317, 71–76 CrossRef CAS.
- R. Ma, D. Golberg, Y. Bando and T. Sasaki, Syntheses and properties of B–C–N and BN nanostructures, Philos. Trans. R. Soc., A, 2004, 362, 2161–2186 CrossRef CAS PubMed.
- H. Dai, A. G. Rinzler, P. Nikolaev, A. Thess, D. T. Colbert and R. E. Smalley, Single-wall nanotubes produced by metal-catalyzed disproportionation of carbon monoxide, Chem. Phys. Lett., 1996, 260, 471–475 CrossRef CAS.
- S. Sinnott, R. Andrews, D. Qian, A. Rao, Z. Mao, E. Dickey and F. Derbyshire, Model of carbon nanotube growth through chemical vapor deposition, Chem. Phys. Lett., 1999, 315, 25–30 CrossRef CAS.
- Z. Zhang, B. Wei, G. Ramanath and P. Ajayan, Substrate-site selective growth of aligned carbon nanotubes, Appl. Phys. Lett., 2000, 77, 3764–3766 CrossRef CAS PubMed.
- B. Wei, R. Vajtai, Z. Zhang, G. Ramanath and P. Ajayan, Carbon nanotube-magnesium oxide cube networks, J. Nanosci. Nanotechnol., 2001, 1, 35–38 CrossRef CAS PubMed.
- Y. C. Choi, Y. M. Shin, Y. H. Lee, B. S. Lee, G.-S. Park, W. B. Choi, N. S. Lee and J. M. Kim, Controlling the diameter, growth rate, and density of vertically aligned carbon nanotubes synthesized by microwave plasma-enhanced chemical vapor deposition, Appl. Phys. Lett., 2000, 76, 2367–2369 CrossRef CAS PubMed.
- L. Ci, L. Song, C. Jin, D. Jariwala, D. Wu, Y. Li, A. Srivastava, Z. Wang, K. Storr and L. Balicas, Atomic layers of hybridized boron nitride and graphene domains, Nat. Mater., 2010, 9, 430–435 CrossRef CAS PubMed.
- L. Song, L. Ci, H. Lu, P. B. Sorokin, C. Jin, J. Ni, A. G. Kvashnin, D. G. Kvashnin, J. Lou and B. I. Yakobson, Large scale growth and characterization of atomic hexagonal boron nitride layers, Nano Lett., 2010, 10, 3209–3215 CrossRef CAS PubMed.
- Y. Shi, C. Hamsen, X. Jia, K. K. Kim, A. Reina, M. Hofmann, A. L. Hsu, K. Zhang, H. Li and Z.-Y. Juang, Synthesis of few-layer hexagonal boron nitride thin film by chemical vapor deposition, Nano Lett., 2010, 10, 4134–4139 CrossRef CAS PubMed.
- K. K. Kim, A. Hsu, X. Jia, S. M. Kim, Y. Shi, M. Hofmann, D. Nezich, J. F. Rodriguez-Nieva, M. Dresselhaus and T. Palacios, Synthesis of monolayer hexagonal boron nitride on Cu foil using chemical vapor deposition, Nano Lett., 2011, 12, 161–166 CrossRef PubMed.
- P. Ahmad, M. U. Khandaker, Y. M. Amin and N. Muhammad, Synthesis and characterization of boron nitride microtubes, Mater. Express, 2015, 5, 249–254 Search PubMed.
- P. Ahmad, M. Khandaker and Y. Amin, Simple technique to synthesise vertically aligned boron nitride nanosheets at 1200 °C, Adv. Appl. Ceram., 2014 DOI:10.1179/1743676114y.0000000225.
|
This journal is © The Royal Society of Chemistry 2015 |
Click here to see how this site uses Cookies. View our privacy policy here.