DOI:
10.1039/C5RA01889G
(Communication)
RSC Adv., 2015,
5, 18172-18176
Domino transformation of isoxazoles to 2,4-dicarbonylpyrroles under Fe/Ni relay catalysis†
Received
14th January 2015
, Accepted 5th February 2015
First published on 5th February 2015
Abstract
The domino reaction of 5-alkoxy- or 5-aminoisoxazoles, under metal relay catalysis, with symmetric 1,3-diketones gives 4-acylpyrrole-2-carboxylic acid derivatives in high yield. Esters and amides of acylacetic acids react regioselectively, giving derivatives of pyrrole-2,4-dicarboxylic acid as the main products.
Pyrroles, indoles and other fused pyrrole derivatives are viewed by medicinal chemists as privileged structures.1 These heterocyclic fragments are widely present in natural compounds, pharmaceuticals and material molecules.2 Not surprisingly, the development of methods for the synthesis of pyrrole derivatives is still a very active area of research,3 although classical methods for the synthesis of such structures are well known. Straightforward and selective routes to synthesize pyrrole derivatives using cheap and available starting materials are therefore highly attractive. Multicatalysis is currently one of the most promising approaches for the construction of molecular frameworks in a resource-efficient and sustainable manner.4 Application of this approach for the synthesis of compounds with a pyrrole fragment has been utilized, as yet, only for the synthesis of certain indoles. Thus, Ackermann developed a three-component synthesis of substituted indoles by the use of a multicatalytic system consisting of an N-heterocyclic carbene palladium complex and CuI.5 Peng, Wu and coworkers reported the approach to indole derivatives through a multicatalytic one-pot Beckmann rearrangement-intramolecular cyclization–halogenation reaction of 1-(2-alkynylphenyl)ketoxime.6 No syntheses of pyrroles under multicatalysis have been published to the best of our knowledge, and only some pyrrolines were prepared using the multicatalytic concept.7 Maruoka and coworkers found an enantioselective synthesis of the pyrrolidine core structure starting from glycine esters in combination with several different organocatalytic reactions.7a Cernak and Lambert developed a synthesis of pyrrolidinyl ketones by incorporation of a catalytic aminochlorocarbonylation into double and triple catalytic processes involving Friedel–Crafts acylation.7b Wang and coworkers discovered an aza-Michael/carbocyclization reaction of α,β-unsaturated aldehydes and N-tosyl propargylamines under organo/metal cooperative catalysis, leading to chiral pyrrolines.7c
In this communication, we wish to report the first example of a preparation of pyrroles by the domino reaction of isoxazoles and 1,3-dicarbonyl compounds under metal relay catalysis. Auricchio and coworkers8 found that 5–10 mol% of FeCl2·4H2O effectively catalyzes the transformation of 3-aryl-5-methoxy/alkylamino-isoxazoles to derivatives of 3-aryl-2H-azirine-2-carboxylic acid at room temperature. In turn, 2H-azirines react with 1,3-dicarbonyl compounds to give substituted pyrroles.9,10 These reactions usually need the transformation of the 1,3-dicarbonyl compound into the corresponding metal-enolate10a or catalysis by transition metals, Ni(acac)2,10b Cu(acac)2 (ref. 10c–e) or VO(OSiPh3)3.10f We envisioned that the application of the multicatalysis concept would permit the transformation 1 → 3 + 2 → 4 as a domino reaction (Scheme 1), without isolation of 2H-azirines, which are often unstable under isolation and storing.9
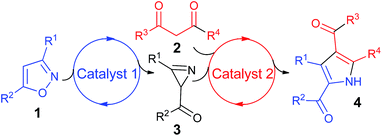 |
| Scheme 1 Relay catalysis scheme for the synthesis of pyrroles 4 from isoxazoles 1. | |
Test experiments were performed with 5-methoxy-3-phenylisoxazole 1a, acetylacetone 2a, and ethyl 3-phenyl-2H-azirine-2-carboxylate 3a as starting materials (Table 1). At the beginning we attempted to obtain pyrrole 4a from azirine 3a and acetylacetone 2a under conditions used by Auricchio and coworkers8 for the transformation of 5-alkoxyisoxazoles 1 into azirines 3 (Table 1, entry 1), but the starting materials were left unchanged even after a longer reaction time.
Table 1 Optimization of the reaction conditions for the transformation of isoxazoles to pyrrole
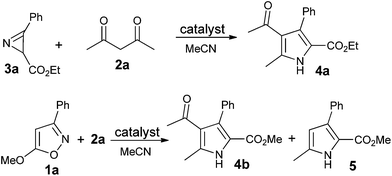
|
Entry |
Reactants |
Catalyst |
Reaction conditions |
Yield of products, % |
1 |
3a + 2a |
10 mol% FeCl2·4H2O |
20 °C, 10 days |
— |
2 |
3a + 2a |
10 mol% Cu(OAc)2·H2O |
20 °C, 10 days |
— |
3 |
3a + 2a |
10 mol% NiCl2·6H2O |
20 °C, 24 h |
4a, 77% |
4 |
1a + 2a |
10 mol% NiCl2·6H2O |
20 °C, 2 weeks |
— |
5 |
1a + 2a |
(1) 10 mol% FeCl2·4H2O; (2) 10 mol% NiCl2·6H2O |
(1) 20 °C, 2 h; (2) 20 °C, 24 h |
4b, 82% |
6 |
1a + 2a |
10 mol% FeCl2·4H2O + 10 mol% NiCl2·6H2O |
20 °C, 24 h |
4b, 82% |
7 |
1a + 2a |
20 mol% FeCl2·4H2O |
65 °C, 20 h |
4b, 56%; 5, 17% |
The use of Cu(OAc)2·H2O was also unsuccessful (Table 1, entry 2), whereas 77% of pyrrole 4a was obtained with NiCl2·6H2O as catalyst (Table 1, entry 3). Attempts to prepare pyrrole 4b by reaction of isoxazole 1a and acetylacetone 2a with NiCl2·6H2O as catalyst were unsuccessful (Table 1, entry 4). Therefore, multicatalysis with FeCl2 and NiCl2 in a sequential (Table 1, entry 5) and simultaneous (Table 1, entry 6) manner was tried and gave pyrrole 4b in good yield. It was also found that the use of 5 and 1 mol% NiCl2·4H2O instead of 10% increases the reaction time by 1.5 and 5 times, respectively. The use of FeCl2 as the lone catalyst also gave pyrrole 4b, but only under heating and in lower yield (Table 1, entry 7). Byproduct, pyrrole 5, also arose from decarbonylation under these conditions. Therefore, the reaction conditions listed in entry 6 of Table 1 were used further for the preparation of a series of pyrrole-2-carboxylic acid derivatives, starting from isoxazoles 1a–h and diones 2a and b. This very simple procedure afforded compounds 4b–e and g–k in good yields, except for compound 4f with a 4-Me2NC6H4 substituent (Table 2). The lowering of the yield in this case was probably caused by the instability of the transient azirine and pyrrole 4f under these reaction conditions.
Table 2 Synthesis of pyrroles 4b–k
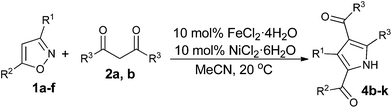
|
Entry |
R1 |
R2 |
R3 |
1 |
2 |
Time, days |
Yield of 4, % |
1 |
Ph |
MeO |
Me |
a |
a |
1 |
b, 82 |
2 |
Ph |
tBuO |
Me |
b |
a |
2 |
c, 81 |
3 |
Ph |
(CH2)4N |
Me |
c |
a |
1 |
d, 71 |
4 |
4-ClC6H4 |
MeO |
Me |
d |
a |
1 |
e, 92 |
5 |
4-Me2NC6H4 |
MeO |
Me |
e |
a |
1 |
f, 23 |
6 |
Ph |
MeO |
Ph |
a |
b |
2 |
g, 90 |
7 |
4-BrC6H4 |
MeO |
Ph |
f |
b |
1 |
h, 64 |
8 |
4-MeOC6H4 |
MeO |
Ph |
g |
b |
2 |
i, 72 |
9 |
Me |
MeO |
Me |
h |
a |
2 |
k, 87 |
The structures of compounds 4b–k were verified by 1H and 13C NMR, IR spectroscopy, and mass-spectrometry. The spectral and physical data for known compounds 4b and g corresponds to published data.11,12
Because of the important bioactivity of compounds with a tetrahydroindolone skeleton13 the multicatalysis approach for the domino preparation of these indole derivatives was investigated. Reaction of dimedone 6 with isoxazoles 1 under the conditions used for the preparation of pyrroles 4b–k was too slow at room temperature. It was found that heating at 65 °C makes the rates of the reactions acceptable and tetrahydroindolones 7a–d were obtained in good yields from 3-arylisoxazoles 1 (Table 3). 3-Methylisoxazole 1h gave tetrahydroindolones 7e in low yield, probably due to the instability both of the isoxazole and the corresponding transient azirine at 65 °C.
Table 3 Synthesis of tetrahydroindolones 7a–e
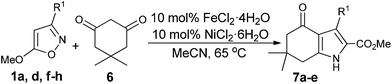
|
Entry |
R1 |
1 |
Time, h |
Yield of 7, % |
1 |
Ph |
a |
8 |
a, 86 |
2 |
4-ClC6H4 |
d |
10 |
b, 70 |
3 |
4-BrC6H4 |
f |
8 |
c, 70 |
4 |
4-MeOC6H4 |
g |
36 |
d, 76 |
5 |
Me |
h |
12 |
e, 18 |
The reactions of isoxazoles 1 with 3-oxopropanoates 8 are also slower than with acyclic diketones 2 and therefore were carried out with heating (Table 4).
Table 4 Synthesis of derivatives of pyrrole-2,4-dicarboxylic acid 9a–e
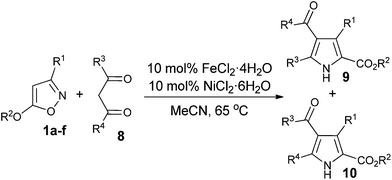
|
Entry |
R1 |
R2 |
R3 |
R4 |
1 |
8 |
Time, h |
Yield of 9, % |
Yield of 10, % |
Room temperature. |
1 |
Ph |
Me |
Ph |
EtO |
a |
a |
72a |
a, 52 |
a, 12 |
2 |
4-ClC6H4 |
Me |
Ph |
EtO |
d |
a |
10 |
b, 47 |
b, 17 |
3 |
4-MeOC6H4 |
Me |
Ph |
EtO |
g |
a |
10 |
c, 33 |
c, 11 |
4 |
Ph |
tBu |
Me |
EtO |
b |
b |
9 |
d, 34 |
— |
5 |
Ph |
Me |
Me |
EtO |
a |
b |
9 |
e, 58 |
— |
6 |
4-ClC6H4 |
Me |
Me |
EtO |
d |
b |
8 |
f, 40 |
d, 4 |
7 |
4-BrC6H4 |
Me |
Me |
EtO |
f |
b |
8 |
g, 41 |
e, 11 |
8 |
4-Me2NC6H4 |
Me |
Me |
EtO |
e |
b |
8 |
h, 36 |
— |
9 |
4-MeOC6H4 |
Me |
Me |
EtO |
g |
b |
8 |
i, 51 |
— |
10 |
Ph |
Me |
Me |
PhHN |
a |
c |
6 |
k, 50 |
— |
11 |
4-BrC6H4 |
Me |
Me |
PhHN |
f |
c |
6 |
l, 65 |
— |
In this case the reaction can proceed via the two different carbonyl groups of 8 to give two isomeric pyrroles 9 and 10. Derivatives of pyrrole-2,4-dicarboxylic acid 9 were the main products (yields of 33–65%). Minor products, 5-ethoxypyrroles 10a–c, were formed in all the reactions performed with ethyl 3-oxo-3-phenylpropanoate 8a, and 5-ethoxypyrroles 10d, e were formed in reactions of 4-halophenyl-substituted isoxazoles 1d, f with ethyl acetoacetate 8b. Replacing the ester group by the less electrophilic amide group (8b → 8c) makes the reaction regioselective, giving only derivatives 9 even with isoxazole 1f.
The plausible mechanism for the metal-catalyzed reaction of isoxazoles with 1,3-dicarbonyl compounds is presented on Scheme 2. We assume that isoxazole 1 reacts with FeCl2, giving the unstable azirinium complex 11, which undergoes transmetalation with formation of Ni-complex 12. The latter reacts with the enol-form (or metal-enolate) 13 of compounds 2, 6 or 8, giving intermediate 14/15 (14
15 in the case of compounds 2, 6 with R3 = R4). The attack of the aziridine nitrogen on the quasi protonated/metalated C
O+H/M group of 14/15 leads to intermediate 16/17. Intermediates 16/17 give pyrroles 4, 7, 9/10 via aziridine ring opening and elimination of H2O. The regioselectivity of the reaction in the case of compounds 8 in favour of pyrrole 9 is obviously caused by a shift of the equilibrium 14 ⇆ 15 to the 14 side (R4 = OEt, NHPh).
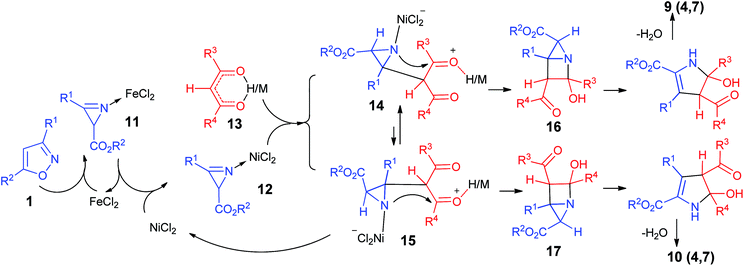 |
| Scheme 2 Plausible mechanism for the relay metal catalyzed reaction of isoxazoles and 1,3-dicarbonyl compounds. | |
Conclusions
A simple procedure for the preparation of derivatives of 4-acylpyrrole-2-carboxylic acid, 4,5,6,7-tetrahydro-4-oxo-1H-indole-2-carboxylic acid, and pyrrole-2,4-dicarboxylic acid by domino reaction of 5-alkoxy- or 5-aminoisoxazoles with 1,3-dicarbonyl compounds under Fe(II)/Ni(II) relay catalysis was developed. The approach is especially effective using symmetric 1,3-diketones as starting materials. Esters and amides of acylacetic acids react regioselectively giving derivatives of pyrrole-2,4-dicarboxylic acid as the main products, but in lower yields.
Acknowledgements
We gratefully acknowledge the financial support of the Russian Foundation for Basic Research (grant no. 14-03-00187) and Saint Petersburg State University (grant no. 12.50.1565.2013, 12.38.239.2014, 12.38.217.2015). This research used resources of ‘Research resource center for Magnetic Resonance’ and ‘Center for Chemical Analysis and Material Research’ of Saint Petersburg State University.
Notes and references
-
(a) M. E. Welsch, S. A. Snyder and B. R. Stockwell, Curr. Opin. Chem. Biol., 2010, 14, 347 CrossRef CAS PubMed;
(b) R. W. DeSimone, K. S. Currie, S. A. Mitchell, J. W. Darrow and D. A. Pippin, Comb. Chem. High Throughput Screening, 2004, 7, 473 CrossRef CAS;
(c) L. Costantino and D. Barlocco, Curr. Med. Chem., 2010, 5, 381 CAS.
- For recent review, see:
(a) D. L. J. Clive and P. Cheng, Tetrahedron, 2013, 69, 5067 CrossRef CAS;
(b) Y.-J. Wu, Heterocycles and Medicine: A Survey of Heterocyclic Drugs Approved by U.S. FDA from 2000 to Present, in Progress in heterocyclic chemistry, ed. G. W. Gribble and J. A. Joule, 2012, vol. 24, pp. 1–54 Search PubMed;
(c) M. Baumann, I. R. Baxendale, S. V. Ley and N. Nikbin, Beilstein J. Org. Chem., 2011, 7, 442 CrossRef CAS PubMed;
(d) K.-I. Shinohara, T. Bando and H. Sugiyama, Anti-Cancer Drugs, 2010, 21, 228 CrossRef CAS PubMed;
(e) A. Hagfeldt, G. Boschloo, L. Sun, L. Kloo and H. Pettersson, Chem. Rev., 2010, 110, 6595 CrossRef CAS PubMed;
(f) I. S. Young, P. D. Thornton and A. Thompson, Nat. Prod. Rep., 2010, 27, 1801 RSC;
(g) H. Nishide and K. Oyaizu, Science, 2008, 319, 737 CrossRef CAS PubMed.
- For recent review, see:
(a) B. A. Trofimov, A. I. Mikhaleva, E. Yu Schmidt and L. N. Sobenina, Chemistry of Pyrroles, CRC Press Inc., Boca-Raton, 2014, p. 398 Search PubMed;
(b) V. Estévez, M. Villacampa and J. C. Menéndez, Chem. Soc. Rev., 2014, 43, 4633 RSC;
(c) M. A. Yurovskaya and R. S. Alekseyev, Chem. Heterocycl. Compd., 2014, 49, 1400 CrossRef CAS;
(d) L. N. Sobenina, D. N. Tomilin and B. A. Trofimov, Russ. Chem. Rev., 2014, 83, 475 CrossRef;
(e) M. S. Mohamed and S. S. Fatahala, Mini-Rev. Org. Chem., 2014, 11, 477 CrossRef CAS;
(f) F. J. Leepera and J. M. Kelly, Org. Prep. Proced. Int., 2013, 45, 171 CrossRef.
- For recent review, see:
(a) P.-F. Xu and J.-B. Ling, in Catalytic Cascade Reactions, ed. P.-F. Xu and W. Wang, John Wiley & Sons, Inc., 2014, p. 363 Search PubMed;
(b) J. A. Mata, F. E. Hahn and E. Peris, Chem. Sci., 2014, 5, 1723 RSC;
(c) H. Pellisier, Tetrahedron, 2013, 69, 7171 CrossRef;
(d) Z. Du and Z. Shao, Chem. Soc. Rev., 2013, 42, 1337 RSC;
(e) R. C. Wende and P. R. Schreiner, Green Chem., 2012, 14, 1821 RSC;
(f) A. E. Allen and D. W. C. MacMillan, Chem. Sci., 2012, 3, 633 RSC.
-
(a) L. Ackermann, Org. Lett., 2005, 7, 439 CrossRef CAS PubMed;
(b) L. T. Kaspar and L. Ackermann, Tetrahedron, 2005, 61, 11311 CrossRef CAS.
- G. Qiu, Q. Ding, H. Ren, Y. Peng and J. Wu, Org. Lett., 2010, 12, 3975 CrossRef CAS PubMed.
-
(a) Y.-G. Wang, T. Kumano, T. Kano and K. Maruoka, Org. Lett., 2027, 209, 11 Search PubMed;
(b) T. A. Cernak and T. H. Lambert, J. Am. Chem. Soc., 2009, 131, 3124 CrossRef CAS PubMed;
(c) W. Sun, G. Zhu, L. Hong and R. Wang, Chem.–Eur. J., 2011, 17, 13958 CrossRef CAS PubMed.
- S. Auricchio, A. Bini, E. Pastormerlo and A. M. Truscello, Tetrahedron, 1997, 53, 10911 CrossRef CAS.
- For review, see:
(a) F. Palacios, A. M. O. de Retana, E. M. de Marigorta and J. M. de los Santos, Eur. J. Org. Chem., 2001, 2401 CrossRef CAS;
(b) F. Palacios, A. M. O. de Retana, E. M. de Marigorta and J. M. de los Santos, Org. Prep. Proced. Int., 2002, 34, 219 CrossRef CAS;
(c) A. Padwa, in Comprehensive Heterocyclic Chemistry III, ed. A. R. Katritzky, C. A. Ramsden, E. F. V. Scriven and R. J. K. Taylor, Elsevier, Oxford, 2008, vol. 1, pp. 1–104 Search PubMed;
(d) A. F. Khlebnikov and M. S. Novikov, Tetrahedron, 2013, 69, 3363 CrossRef CAS;
(e) C.-Y. Huang and A. G. Doyle, Chem. Rev., 2014, 114, 8153 CrossRef CAS PubMed.
-
(a) F. Palacios, A. M. O. de Retana and A. V. del Burgo, J. Org. Chem., 2011, 76, 9472 CrossRef CAS PubMed;
(b) P. F. Dos Santos Filho and U. Schuchardt, Angew. Chem., Int. Ed. Engl., 1977, 16, 647 CrossRef;
(c) S. Chiba, Y.-F. Wang, G. Lapointe and K. Narasaka, Org. Lett., 2008, 10, 313 CrossRef CAS PubMed;
(d) X.-X. Qi, Y.-J. Jiang and C.-M. Park, Chem. Commun., 2011, 47, 7848 RSC;
(e) N. V. Rostovskii, M. S. Novikov, A. F. Khlebnikov, S. M. Korneev and D. S. Yufit, Org. Biomol. Chem., 2013, 11, 5535 RSC;
(f) C. R. Alonso-Cruz, R. Freire, M. S. Rodríguez and E. Suárez, Synlett, 2007, 2723 CAS.
-
(a) K. W. Law, T.-F. Lai, M. P. Sammes, A. R. Katritzky and T. C. W. Mak, J. Chem. Soc., Perkin Trans. 1, 1984, 111 RSC;
(b) M. P. Sammes, A. R. Katritzky and K. W. Law, Magn. Reson. Chem., 1986, 827 CrossRef CAS.
- S. Auricchio, A. M. Truscello, M. Lauria and S. V. Meille, Tetrahedron, 2012, 68, 7441 CrossRef CAS.
-
(a) K. Nagarajan, P. K. Talwalker, A. N. Goud, R. K. Shah, S. J. Shenoy and N. D. Desai, Indian J. Chem., Sect. B: Org. Chem. Incl. Med. Chem., 1998, 27B, 1113 Search PubMed;
(b) R. Martínez, A. Clara-Sosa and M. T. Ramírez-Apan, Bioorg. Med. Chem., 2007, 15, 3912 CrossRef PubMed;
(c) E. Vanotti, R. Amici, A. Bargiotti, J. Berthelsen, R. Bosotti, A. Ciavolella, A. Cirla, C. Cristiani, R. D'Alessio, B. Forte, A. Isacchi, K. Martina, M. Menichincheri, A. Molinari, A. Montagnoli, P. Orsini, A. Pillan, F. Roletto, A. Scolaro, M. Tibolla, B. Valsasina, M. Varasi, D. Volpi and C. Santocanale, J. Med. Chem., 2008, 51, 487 CrossRef CAS PubMed;
(d) K. H. Huang, J. M. Veal, R. P. Fadden, J. W. Rice, J. Eaves, J. P. Strachan, A. F. Barabasz, B. E. Foley, T. E. Barta, W. Ma, M. A. Silinski, M. Hu, J. M. Partridge, A. Scott, L. G. DuBois, T. Freed, P. M. Steed, A. J. Ommen, E. D. Smith, P. F. Hughes, A. R. Woodward, G. J. Hanson, W. S. McCall, C. J. Markworth, L. Hinkley, M. Jenks, L. Geng, M. Lewis, J. Otto, B. Pronk, K. Verleysen and S. E. Hall, J. Med. Chem., 2009, 52, 4288 CrossRef CAS PubMed;
(e) C. C. Chiang, Y. H. Lin, S. F. Lin, C. L. Lai, C. Liu, W. Y. Wei, S. C. Yang, R. W. Wang, L. W. Teng, S. H. Chuang, J. M. Chang, T. T. Yuan, Y. S. Lee, P. Chen, W. K. Chi, J. Y. Yang, H. J. Huang, C. B. Liao and J. J. Huang, J. Med. Chem., 2010, 53, 5929 CrossRef CAS PubMed;
(f) R. Gonzalez-Chavez, R. Martinez, M. E. Torre-Bouscoulet, M. Gallo and M. M. Gonzalez-Chavez, Chem. Pharm. Bull., 2014, 62, 16 CrossRef CAS PubMed.
Footnote |
† Electronic supplementary information (ESI) available: Detailed experimental procedures and characterization data. See DOI: 10.1039/c5ra01889g |
|
This journal is © The Royal Society of Chemistry 2015 |