DOI:
10.1039/C5RA07206A
(Paper)
RSC Adv., 2015,
5, 53819-53828
Eco-friendly superabsorbent composite based on sodium alginate and organo-loess with high swelling properties
Received
21st April 2015
, Accepted 3rd June 2015
First published on 3rd June 2015
Abstract
A novel superabsorbent composite with high swelling properties was synthesized by the grafted co-polymerization of partially neutralized acrylic acid (AA) onto a sodium alginate (NaAlg) backbone in the presence of organo-loess. The FTIR spectra, XRD patterns and SEM micrographs prove that the AA monomers were grafted onto the NaAlg backbone, and the organo-loess dispersed into the polymer matrix that improved the porous structure, which was verified by element mapping. TGA and DSC results indicate that the incorporation of loess enhances the thermal stability of the superabsorbent. Swelling results confirm that proper amount of organo-loess in the superabsorbent can enhance the swelling capability and salt-resistant performance. The maximum equilibrium water absorbency of the superabsorbent composite incorporated with 10 wt% organo-loess in distilled water and 0.9 wt% NaCl aqueous solution were 656 g g−1 and 69 g g−1, respectively. Furthermore, the superabsorbent composite exhibited good buffer ability to external pH in the range from 4 to 10 and water retention ability. According to the performance of the eco-friendly superabsorbent composite, it can be used as a promising candidate for applications in various fields.
1. Introduction
Superabsorbents are moderately crosslinked three-dimensional hydrophilic polymer materials, which can imbibe a large amount of water or aqueous solution and display a slower water-releasing rate than traditional absorbent materials under the same conditions. The superabsorbents not only have a high water absorbency but they also exhibit an excellent water retention (WR) capacity.1 Owing to their excellent properties, superabsorbents are widely used in many fields such as agriculture,2,3 chemical engineering,4 biomedical area,5,6 tissue engineering,7,8 waste-water treatment9 and other environmental fields.10,11 In general, superabsorbents include synthetic, semi-synthetic and natural polymers. Although synthetic superabsorbents have large water absorbing capacities, the consumed polymers have led to serious environmental pollution.12 Thus, the development of eco-friendly natural-based superabsorbent, which incorporates biodegradable and renewable polymers, have drawn much interest owing to their abundant resources, low production cost and good biodegradability.
Recent research focused attention towards the superabsorbent polymers based on natural polysaccharides for their unique properties, including biocompatibility, biodegradability, renewability and nontoxicity. Various polysaccharides, such as carrageenan,13 gum ghatti,14 chitosan,15 guar gum16 and alginate,17 have been investigated on hydrogel formulations. The resultant polymer exhibits quite different characteristics than the individual materials. For example, the electrical conductivity of the resultant hydrogel is compared to that of bare hydrogel.18 Due to these excellent properties, the polymers based on natural polysaccharides have found applications in various fields such as in agriculture, sensors, biomedical and pharmaceutical.13,14,19 Moreover, much attention has also been focused on inorganic clay materials for preparing superabsorbent composites, owing to the environmental advantages and practical applications. Clays, including kaolin,20 vermiculite,21 attapulgite,17 montmorillonite,22 muscovite23 and rectorite,12 have already been incorporated into poly(acrylic acid) and polyacrylamide polymeric network to reduce production costs, improve the network structure and properties of superabsorbents, as well as accelerate the generation of new materials for special application.24
Sodium alginate (NaAlg) is a linear chained anionic natural polysaccharide composed of 1,4-linked β-D-mannuronic acid (M block units) and α-L-guluronic acid (G block units), which are arranged in an irregular blockwise pattern of various proportions of GG, MG, and MM blocks (Scheme 1).25 NaAlg is renewable, abundant, nontoxic, water-soluble, biodegradable and biocompatible, because it is generally extracted from various species of brown algae. It has plentiful free hydroxyl and carboxyl groups distributed along the backbone, which can be easily crosslinked with other multivalent cations such as calcium or organic crosslinkers like glutaraldehyde,26 grafted co-polymerization with hydrophilic vinyl monomers, polymer blending and compounding with other functional components.27 The abovementioned properties make it ideal for industrial applications.
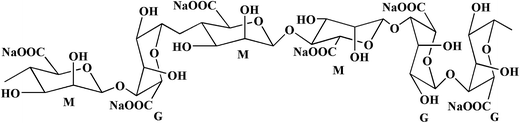 |
| Scheme 1 Chemical structure of NaAlg. | |
Loess is a type of hydrous magnesium aluminum silicate with reactive hydroxyl groups on its surface. Due to its hydrophilic property, abundant reserves and extremely low prices, loess is an ideal inorganic component to improve the network structure and swelling property. Organic modified loess with quaternary ammonium salt can change the surface properties and render a hydrophilic silicate, thereby resulting in the alteration of adhesion and dispersing performances of loess in a polymer matrix.28 Inherent advantages of the inorganic component and the strong interfacial interactions between the dispersed loess and the polymer matrix enhance the thermal stability as well as swelling and adsorption behavior of the virgin polymer.23 To the best of our knowledge, there has been a few reports about the preparation of superabsorbent based on natural loess clay, but there is no literature based on organo-loess. Therefore, the introduction of organo-loess in a superabsorbent is expected to provide a new method to extend the utilization of loess, reduce the cost and improve the biocompatibility and biodegradability of the superabsorbent.
As a further study for organic–inorganic compound superabsorbents, our focus is on providing new strategies for the high-value utilization of cheap natural loess and sodium alginate, improving swelling properties and reducing production cost of the corresponding superabsorbents. The incorporation of biodegradable and renewable natural polysaccharide (NaAlg) can improve the biodegradability of corresponding superabsorbent materials, as well as reduce the dependence on petrochemical derived monomers. In this study, a novel and eco-friendly superabsorbent composite was prepared by the grafted co-polymerization of partially neutralized acrylic acid (AA) onto sodium alginate (NaAlg) backbones in the presence of organo-loess in an aqueous solution. The composite was characterized by Fourier transform infrared (FTIR) spectroscopy, X-ray diffraction (XRD), scanning electron microscopy (SEM), element mapping and thermogravimetric analysis (TGA) and DSC. The effects of organo-loess on the water absorption abilities in distilled water and 0.9 wt% NaCl solutions were discussed. Furthermore, factors such as pH value, surfactants and salines that could affect the swelling ratio of superabsorbent composites and water retention capacity were also systematically investigated.
2. Experimental
2.1 Materials
Loess was collected from the WuQuan mountain in Lanzhou city, Gansu province, China. Sodium alginate (NaAlg, Shanghai chemical reagents Co., China, average molecular weight 500
000 and degree of deacetylation 84%), acrylic acid (AA, analytical grade, Tianjin Kaixin Chemical Industrial Co., China), ammonium persulfate (APS, analytical grade, Yantai Shuangshuang Chemical Industrial Co., China), N,N-methylenebisacrylamide (MBA, chemically pure, Sinopharm Chemical Reagent Co., China), sodium dodecyl benzene sulfonate (SDBS, analytical grade, Sinopharm Chemical Reagent Co., China), cetyltrimethyl ammonium bromide (CTAB, analytical grade, Shanghai Chemical Reagent Co., China) and all other reagents used were of analytical grade and all solutions were prepared with distilled water.
2.2 Preparation of organo-loess
Organo-loess was prepared as follows: 10.0 g loess purified by a suspension method was immersed in 100 mL distilled water in a 250 mL flask and heated for 30 min with stirring at 1250 rpm. Then 1.0 g of CTAB was added into the flask. The mixture was stirred vigorously at 85 °C for 90 min. Then, the product was washed and filtered repeatedly until no Br− was detected by 0.1 mol L−1 AgNO3 aqueous solution in the filtrate. The product was dried for several hours to a constant weight at 60 °C in an oven and then milled to the 320 mesh size for further use.
2.3 Preparation of superabsorbent composites
A series of superabsorbent composites with different amounts of organo-loess were prepared according to the following procedure. 1.2 g of NaAlg was dissolved in 30 mL distilled water in a 250 mL four-necked flask equipped with a mechanical stirrer, a reflux condenser, a constant pressure dropping funnel and a nitrogen line. The obtained viscous solution was heated to 60 °C in an oil bath for 1 h to form a homogeneous colloidal slurry. Then, an aqueous solution of initiator APS (0.100 g in 5 mL H2O) was added and maintained at 60 °C for 15 min to generate radicals. After cooling the reactants to 40 °C, 17 mL of the mixed solution containing 7.2 g of AA neutralized with 8.5 mL of NaOH solution (8.0 mol L−1), 0.02 g of crosslinker MBA and a calculated amount of organo-loess (0, 0.45, 0.95, 1.5, 2.15 g) were added to the reaction flask. The reaction temperature slowly rose to 70 °C and was maintained at that temperature for 3 h to complete the polymerization. A nitrogen atmosphere was maintained throughout the reaction period. The obtained samples were spread on a dish to dry to a constant weight at 60 °C in an oven. The dry samples were milled and all samples used for the test had a particle size in the range of 20–50 mesh (230–870 μm). Scheme 2 represents the general procedure for the preparation of NaAlg-g-PAA/organo-loess superabsorbent composites.
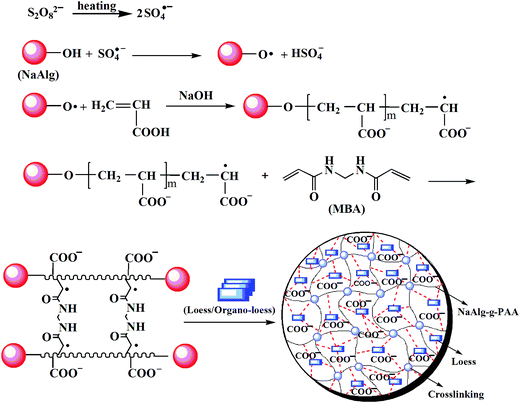 |
| Scheme 2 Proposed mechanism for the formation of a NaAlg-g-PAA/organo-loess (loess) superabsorbent composite. | |
For comparison, the NaAlg-g-PAA and NaAlg-g-PAA/loess composites were prepared under the same conditions.
2.4 Measurements of equilibrium water absorbency
Measurements of equilibrium water absorbency were performed at room temperature according to a conventional filtration method.29 A weighed quantity of the superabsorbent composite (0.10 g) with particles sized between 20 and 50 mesh (230–870 μm) was immersed in 250 mL distilled water or 100 mL of a 0.9 wt% NaCl solution. The samples were allowed to absorb water at room temperature for 4 h to reach swelling equilibrium. Then, the swollen samples were taken out from excess water by filtering through a 100 mesh screen under gravity for 10 min until no redundant water could be removed. After weighing the swollen samples, the equilibrium absorbency (Qeq) of the samples was calculated using the following formula: |
 | (1) |
where m2 and m1 are the weights of the swollen gel and the dried sample, respectively. Qeq was calculated as grams of water per gram of sample.
The determination of water absorbencies at various pH solutions was similar to that of the abovementioned measurement. The pH values of the external solution were adjusted using 0.1 mol L−1 HCl and 0.1 mol L−1 NaOH solutions. The effects of various pH solutions on water absorbency were then achieved. All sample preparations were repeated three times and the average values were reported in this paper.
2.5 Measurements of water absorbency in various saline solutions
Accurately weighed 0.10 g sample was immersed in 250 mL of various saline (NaCl, CaCl2, FeCl3) solutions with different concentrations for 4 h to maintain equilibrium. The swollen samples were filtered through a 100-mesh screen and weighed. The water absorbency in various saline solutions could then be calculated using eqn (1).
2.6 Measurements of water absorbency in surfactant solutions
Water absorbency in different surfactant solutions with various concentrations was determined according to the abovementioned method described in Section 2.5.
2.7 Measurement of water retention (WR)
The determination of the water retention was carried out according to the following procedure.30 Accurately weighed 30 g of fully swollen samples were spread at the bottom of a 250 mL beaker and placed into an oven at 60 and 100 °C. The weight of the swollen samples was determined every 1 h. WR was calculated according to the following formula: |
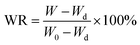 | (2) |
where W0 is the weight of the fully swollen sample, Wd is the weight of the dry sample and W is the weight of the sample heated for different times at a certain temperature.
2.8 Characterization
FTIR measurements were performed on a FTIR-FTS3000 spectrometer. The samples were completely dried before measurement. All spectra collected 40 scans over a wavenumber of 400–4000 cm−1 at 8 cm−1 resolution were obtained from compressed KBr pellets in which the sample concentration was about 3%. The morphologies of the superabsorbent composites were examined using a field emission scanning electron microscope (FESEM, Carl Zeiss Ultra plus, Germany) with an acceleration voltage of 3 kV. Before the SEM observation, the samples were completely dried and coated with a thin layer of gold. The element mapping was carried out using the Elemental Analyzer Vario EL. X-Ray diffraction (XRD) of samples was performed using a Rigaku D/Max-2400 diffractometer with Cu Kα radiation (k = 1.5418 Å) at 40 kV, 100 mA and scanning from 3° to 80° at 5° min−1. Thermogravimetric analysis (TGA) and DSC of synthesized composites have been recorded on a TGA/DSC1 analyzer (Mettler Toledo) at a heating rate of 10 °C min−1 under nitrogen.
3. Results and discussion
3.1 FTIR spectra analysis
The FTIR spectra of (a) loess and (b) organo-loess are shown in Fig. 1. As can be seen, the main characteristic absorption bands of loess at 3619 cm−1 and 3455 cm−1 corresponded to –OH stretching band. The absorption bands at 1622 cm−1, 1024 cm−1, 692 cm−1 and 468 cm−1 were ascribed to bending of –OH, Si–O asymmetric stretching, Si–O–Si symmetric stretching and bending of Si–O (Fig. 1a), respectively.31 Compared with the spectrum of organo-loess, new adsorption peaks at 2919 cm−1 and 2851 cm−1 can be found, which are ascribed to the asymmetric and symmetric stretching vibration of the C–H bonds of alkylammonium chain (Fig. 1b), indicating that the organic cations of CTAB had been exchanged with loess.32
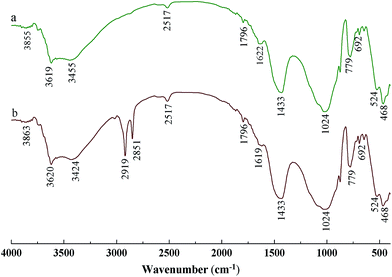 |
| Fig. 1 FTIR spectra of (a) loess and (b) organo-loess. | |
The FTIR spectra of (a) NaAlg, (b) NaAlg-g-PAA, (c) NaAlg-g-PAA/loess, (d) NaAlg-g-PAA/organo-loess are shown in Fig. 2. As can be seen, the characteristic absorption bands of NaAlg at 1093 and 1031 cm−1 (stretching vibration of C–OH groups) are weakened after reaction, which indicate that the –OH groups of NaAlg has participated in a chemical reaction. The new bands at 1559 cm−1 for NaAlg-g-PAA and 1556 cm−1 for NaAlg-g-PAA/organo-loess (asymmetric stretching vibration of –COO− groups), and at 1456 and 1411 cm−1 (symmetric stretching vibration of –COO− groups) are observed in the spectra of NaAlg-g-PAA and NaAlg-g-PAA/organo-loess (Fig. 2b and d), which indicate that PAA chains have been grafted onto the NaAlg backbone.27 The bands at 1569 and 1457–1410 cm−1 are assigned to the asymmetric and symmetric stretching vibration of the –COO− groups, respectively. The –OH absorption bands of organo-loess at 3620 cm−1 can almost not be observed, and the Si–O absorption band at 1024 cm−1 shifted to 1031 cm−1 with a weakened intensity (Fig. 1b and 2d). The abovementioned information confirms that loess and organo-loess have participated in the co-polymerization reaction by its active silanol groups.33
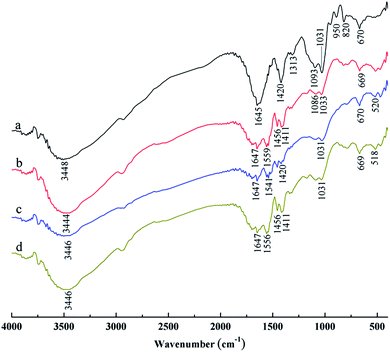 |
| Fig. 2 FTIR spectra of (a) NaAlg, (b) NaAlg-g-PAA, (c) NaAlg-g-PAA/loess and (d) NaAlg-g-PAA/organo-loess. | |
3.2 Morphology analysis
The scanning electron micrographs (SEM) of (a) NaAlg-g-PAA, (b) NaAlg-g-PAA/loess and (c) NaAlg-g-PAA/organo-loess with 10 wt% loess (organo-loess) are presented in Fig. 3. As indicated in Fig. 3, the fracture surface morphology of NaAlg-g-PAA (Fig. 3a) is different from that of NaAlg-g-PAA/loess (Fig. 3b) and NaAlg-g-PAA/organo-loess (Fig. 3c). It can be observed that NaAlg-g-PAA (Fig. 3a) displays a relatively smooth and tight surface. However, the composites containing the loess or organo-loess have an undulant, coarse and rough surface (Fig. 3b and c). However, comparing with the incorporated loess into the composites, the organo-loess makes the pores become smaller and the loess is dispersed more uniformly. This can be attributed to the fact that the long alkyl chains of CTAB can reduce the interaction between hydrophilic groups and enhance the compatibility of loess with the polymeric matrix.34 This fracture surface morphology change is attributed to the introduction of loess, and then may have some influence on the water permeation regions and swelling behaviors of the corresponding superabsorbent composites.
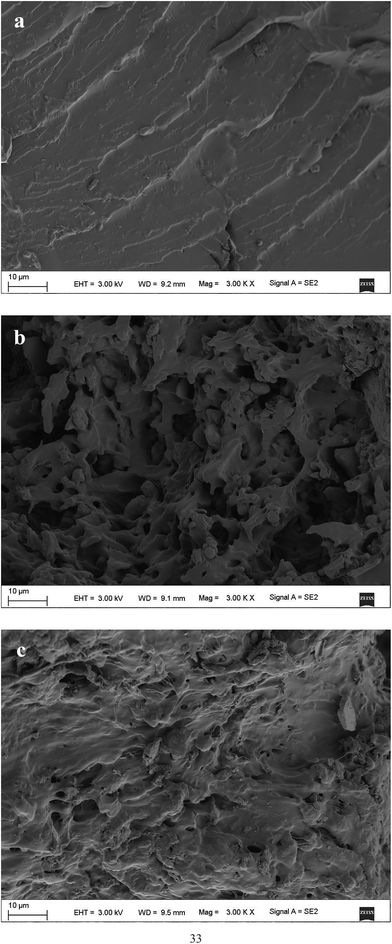 |
| Fig. 3 Scanning electron micrographs of (a) NaAlg-g-PAA, (b) NaAlg-g-PAA/loess and (c) NaAlg-g-PAA/organo-loess. | |
In contrast with NaAlg-g-PAA, NaAlg-g-PAA/organo-loess was further characterized by element mapping images of carbon, oxygen, magnesium, aluminum and silicon to analyze the elemental distribution (Fig. 4). Fig. 4 suggests that magnesium, aluminum and silicon are distributed evenly in carbon and oxygen. That is to say, loess has been evenly distributed in the NaAlg-g-PAA polymeric matrix, because the major ingredient of loess is magnesium, aluminum and silicon.
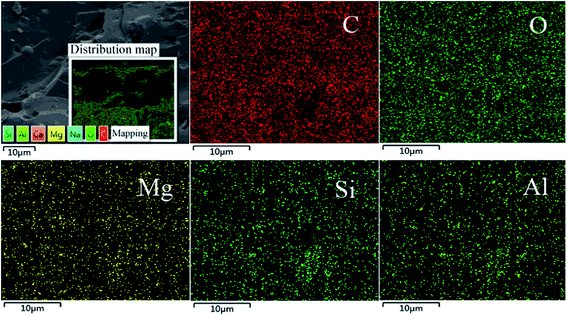 |
| Fig. 4 Element mapping of the NaAlg-g-PAA/organo-loess. | |
3.3 XRD analysis
The reaction between loess and NaAlg-g-PAA was also investigated by XRD. Fig. 5 displays the XRD patterns of the (a) loess, (b) organo-loess, (c) NaAlg-g-PAA/loess and (d) NaAlg-g-PAA/organo-loess superabsorbent composites. The diffraction peaks of loess are observed at 2θ = 8.78° (d = 10.06 Å), 2θ = 19.66° (d = 4.51 Å) and 2θ = 26.56° (d = 3.35 Å), which can be attributed to the characteristic diffraction of mica, quartz and sanidine, respectively. The peaks at 2θ = 12.38° (d = 7.14 Å) and 2θ = 21.96° (d = 4.04 Å) can be attributed to the characteristic diffraction of kaolinite. The abovementioned results indicate the main mineral components of the loess are mica, quartz, sanidine and kaolinite.29 After being organified, the positions of the diffraction peaks of loess had no changes, suggesting that CTAB does not intercalate into the interlayers of loess and is only adsorbed on the surface of loess. However, in the XRD pattern of NaAlg-g-PAA/loess and NaAlg-g-PAA/organo-loess superabsorbent composites, most of the characteristic diffraction peaks of loess cannot be detected and the diffraction peak of loess at 2θ = 26.56° (d = 3.35 Å) has been shifted towards a lower angle at 2θ = 26.42° (d = 3.37 Å). These results indicate that the majority of loess and organo-loess have been exfoliated and dispersed uniformly into the polymer matrix, which should have interacted on the surface of loess rather than be incorporated into the interlayer spacing.
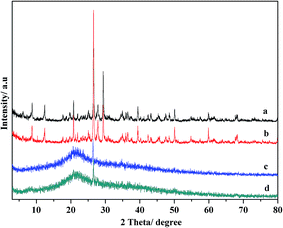 |
| Fig. 5 XRD spectra of the (a) loess, (b) organo-loess, (c) NaAlg-g-PAA/loess and (d) NaAlg-g-PAA/organo-loess. | |
3.4 Thermal stability analysis
The effect of introducing loess on the thermal stability of the synthesized superabsorbent composite was investigated using the TGA technique. It can be observed from Fig. 6 that the NaAlg-g-PAA, NaAlg-g-PAA/loess (10 wt%) and NaAlg-g-PAA/organo-loess (10 wt%) exhibit three-stage thermal decomposition processes, and the weight loss rate of NaAlg-g-PAA is obviously faster than NaAlg-g-PAA/loess and NaAlg-g-PAA/organo-loess. At the initial stage, the weight loss of about 20.1% from 29.5 to 291 °C (for NaAlg-g-PAA) and about 18.8% from 29.1 to 307.2 °C (for NaAlg-g-PAA/organo-loess) may correspond to the loss of adsorbed and bound water. The weight loss of about 30% between 291 and 431 °C for NaAlg-g-PAA is attributed to the dehydration of saccharide rings, the breaking of C–O–C bonds in the chain of NaAlg and the elimination of the water molecule from the two neighboring carboxylic groups of the polymer chains due to the formation of anhydride.23 However, this process is delayed and showed a weight loss of 14.4% in the temperature range of 306–410.6 °C for NaAlg-g-PAA/organo-loess. The successive weight loss of about 20.3% from 406 to 800 °C for NaAlg-g-PAA and about 30.8% in the range of 411.5–800 °C for NaAlg-g-PAA/organo-loess can be attributed to the destruction of carboxylic groups and CO2 evolution, main chain scission and the breakage of the crosslinked network structure.32 Based on the abovementioned information, it can be found that NaAlg-g-PAA/loess and NaAlg-g-PAA/organo-loess shows lower weight loss rate and smaller total weight loss compared with NaAlg-g-PAA, which indicates that the incorporation of loess is helpful to improve the thermal stability of NaAlg-g-PAA. This result is attributed to effects such as a decreased permeability due to a “tortuous path” effect of loess that delays the permeation of oxygen and the escape of volatile degradation products.15 A similar clay effect on the thermal resistance of hydrogel composites was also reported in the literature.29
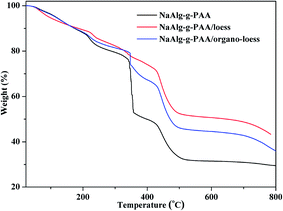 |
| Fig. 6 TGA spectra of the superabsorbent composites. | |
3.5 DSC analysis
The DSC curves of NaAlg-g-PAA, NaAlg-g-PAA/loess (10 wt%) and NaAlg-g-PAA/organo-loess (10 wt%) are given in Fig. 7. It can be observed that NaAlg-g-PAA shows an endothermic peak at 72 °C and an exothermic peak at 210 °C, whereas the NaAlg-g-PAA/loess composite shows an endothermic peak at 77 °C and an exothermic peak at 223 °C. The initial peak at 77 °C corresponds to the oxidation of the water content.35 Compared with NaAlg-g-PAA, the glass transition temperature (Tg) of the composite incorporated in loess tends to move to higher values. This phenomenon can be explained by the coupling model theory; the segmental motion of polymers below Tg is a cooperative process and had to overcome the resistance from the surrounding segments in order to accomplish the transformation between configurations. As more segments are restricted by the presence of loess, the activation threshold for the motion of some segments became higher.36 As a consequence, NaAlg-g-PAA/loess and NaAlg-g-PAA/organo-loess exhibited a higher Tg than NaAlg-g-PAA. In addition, the glass transition temperature of a cross-linked polymer is proportional to the effective cross-link chain density. The abovementioned results indicate that the synthesized superabsorbent composites have a good cross-link density.37
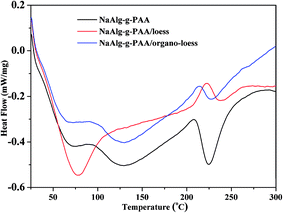 |
| Fig. 7 DSC spectra of the superabsorbent composites. | |
3.6 Effect of loess content on water absorbency
The water absorbencies of NaAlg-g-PAA/loess and NaAlg-g-PAA/organo-loess series superabsorbent composites in distilled water and 0.9 wt% NaCl aqueous solutions are presented in Fig. 8. The content of loess is an important influencing factor on the water absorbencies of the superabsorbent composites. The water absorbencies of the NaAlg-g-PAA/loess and NaAlg-g-PAA/organo-loess composites increased with increasing the content of loess or organo-loess when the content of them is lower than 10 wt%. This tendency may be due to the fact that loess powder participated in the formation of a three-dimensional network structure, and the introduction of loess greatly decreases the hydrogen bonding interaction among hydrophilic groups and restrained the entanglement of polymer chains, thus the physical crosslinking degree is decreased.27 As a result, the swelling capacity can be evidently enhanced. However, with further increasing the content of loess or organo-loess from 10 to 20 wt%, more crosslinking points are generated, which decreases the elasticity of the polymer chains. In addition, the excess of loess also decreases the hydrophilicity as well as the osmotic pressure difference, resulting in the decrease of water absorbency.22 The highest water absorbency for the composite incorporated with 10 wt% organo-loess is 656 g g−1 in distilled water and 69 g g−1 in 0.9 wt% NaCl solution. Furthermore, the changes of water absorbencies for the introduction of organo-loess are more obvious than the introduction of loess both in distilled water and in 0.9 wt% NaCl solutions in the range of loess content investigated. This phenomenon may be attributed to the fact that the organo-loess improve the polymeric network to a higher extent compared with that of the doped with loess. The loess is exchanged by the CTBA ion and the long alkyl chains of CTBA attached onto the surface of loess microparticles, which improve the polymeric network by forming tiny hydrophobic regions and also weaken the hydrogen bonding interaction among hydrophilic groups.38
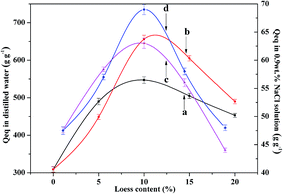 |
| Fig. 8 Effect of loess content in the superabsorbent composites on water absorbencies. (a) NaAlg-g-PAA/loess and (b) NaAlg-g-PAA/organo-loess composite in distilled water; (c) NaAlg-g-PAA/loess and (d) NaAlg-g-PAA/organo-loess composite in 0.9 wt% NaCl solution. | |
These results reveal that the incorporation of moderate amount of loess into a polymer matrix can enhance the water absorbencies of the superabsorbent as well as reduce its dependence on petrochemical-derived monomers. This will provide a novel way to produce low-cost and eco-friendly superabsorbent materials, and render them with great potential for applications in the fields of agriculture and horticulture.
3.7 Effect of cross-linking density on water absorbency
The cross-linking density of the hydrogel network in the synthesized composites is an important swelling control factor and depends upon the amount of crosslinking agent used. In the present study, the variation of MBA amount (0.016–0.024 g) was investigated according to water absorbencies of superabsorbent composites (Fig. 9). The water absorbency increased with increasing the content of crosslinking agent from 0.016 to 0.02 g and the maximum water absorbency was achieved when the amount of crosslinking agent was 0.02 g. This phenomenon is due to the fact that the amount of soluble material increased and the three-dimensional network of the superabsorbent composites could not be formed efficiently when the amount of crosslinking agent was lower than 0.016 g, which resulted in a smaller swelling ratio of the superabsorbent composites. When the amount of crosslinker increased and exceeded 0.02 g, more crosslink points were produced during polymerization and caused the higher cross-linking density and decreased the space of the polymer three-dimensional network, and consequently, it was not beneficial to expand the structure and hold a large quantity of water.18,22
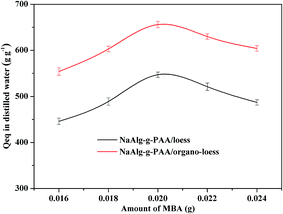 |
| Fig. 9 Effect of MBA amount on the water absorbencies. | |
3.8 Effect of various pH solutions on swelling behaviors
The swelling behaviors of NaAlg-g-PAA, NaAlg-g-PAA/loess (10 wt% loess) and NaAlg-g-PAA/organo-loess (10 wt% organo-loess) superabsorbent composites were investigated in various pH solutions ranging from 2 to 12 (Fig. 10). The solution pH was adjusted by NaOH (pH = 13.0), HCl (pH = 1.0) and distilled water to reach the desired value. The water absorbencies for all tested samples drastically increased in the pH range from 2 to 4 and decreased in the pH range from 10 to 12. This is because most of the –COO− groups change into –COOH groups below a pH value of 4; moreover, the repulsion between polymeric chains decreased, which led to a decrease of water absorbency. When the pH >10, most of the –COOH groups are changed into –COO− groups, and the screening effect of the counter ion (Na+) on the poly-anionic chain is more evident, which also led to a decrease of the water absorbency. This phenomena implies that the buffer action of –COOH and –COO− disappears when a large amount of acid or base is added.39 However, the equilibrium water absorbencies keep roughly constant in the pH range from 4 to 10. This can be attributed to the fact that some of the carboxylate groups are ionized and the ionization degree of the carboxylate groups remains almost constant, which induces a similar osmotic pressure between the hydrogel network and the external solution as well as the electrostatic repulsion among the –COO− groups.
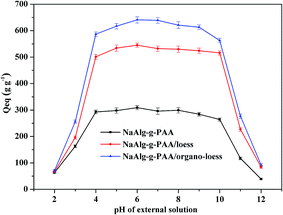 |
| Fig. 10 Water absorbencies of superabsorbent composites as a function of pH values. | |
In addition, the hydrogen bonding interactions among the –COOH groups is partially broken due to the introduction of organo-loess or loess, which widens the mesh size of the network pores and thus enhances the swelling capacity over a wide range of pH values.21 Based on the abovementioned analyses, the superabsorbent composites are very advantageous for use in various soils for agricultural applications.
3.9 Effects of saline solutions on swelling behaviors
The effect of saline solutions on the swelling properties of superabsorbents is of significance to expand their practical applications especially for agriculture and horticulture. In the current section, the influence of saline solution (NaCl, CaCl2, FeCl3) with various concentrations on the swelling properties of NaAlg-g-PAA, NaAlg-g-PAA/loess (10 wt% loess) and NaAlg-g-PAA/organo-loess (10 wt% organo-loess) composites were investigated (Fig. 11a–c). The swelling capacity at equilibrium decreased as the concentration of the external saline solution increased. The reason may be that the increasing saline concentration led to the reduction of the osmotic swelling pressure difference between the polymer matrix and the external solution, which prevented water molecules from penetrating inside the hydrogels.40 It also can be seen that equilibrium swelling capacity of these superabsorbent composites in various saline solutions of the same concentration are in the order NaAlg-g-PAA < NaAlg-g-PAA/loess < NaAlg-g-PAA/organo-loess. This tendency can be explained by the fact that loess is insensitive to saline solution and enhances the osmotic pressure difference between the polymeric network and external saline solutions. Compared with NaAlg-g-PAA/loess, the higher swelling capacity for NaAlg-g-PAA/organo-loess composite in various saline solutions may be due to the fact that the long alkyl chains of organo-loess has interfered the formation of complex between carboxylate groups and cations.38 In addition, the swelling capability of all samples in NaCl solution is higher than in CaCl2 and FeCl3 solutions. This result is mainly caused by the complexing ability difference of the carboxylate group on the superabsorbent network towards different cations. The order of the ability of the carboxylate group to form a complex with the three cations is Na+ < Ca2+ < Fe3+, based on their formation constants with ethylenediamine tetraacetic acid (EDTA).41 These results obtained indicate that the introduction of organo-loess or loess can improve the salt-resistant property of the corresponding superabsorbent composites.
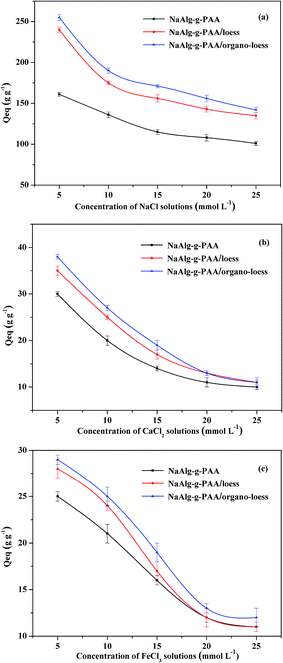 |
| Fig. 11 Equilibrium swelling capacity of the superabsorbent composites in (a) NaCl solutions, (b) CaCl2 and (c) FeCl3 solutions at various concentrations. | |
3.10 Effects of surfactant solutions on swelling behaviors
To investigate the influence of ionic surfactants on the equilibrium swelling behaviors, the swelling behaviors of NaAlg-g-PAA/loess (10 wt% loess) and NaAlg-g-PAA/organo-loess (10 wt% organo-loess) superabsorbent composites in SDBS and CTAB solutions, respectively, with various concentrations were explored(Fig. 12). The swelling capacity of the two types of testing samples all decreased with increasing the concentration of SDBS and CTAB in the solutions. This behavior is attributed to the differences in the counter ions, the ionizable groups and the bound amounts of surfactants.42 However, the decreasing trend in the CTAB solution is more obvious than in the SDBS solution. This may be due to the strong association, binding or interaction of cationic surfactant molecules with the counter ions or ionizable groups of the hydrogels as well as aggregation of the surfactant molecules within or over the networks of hydrogels.43 These factors are responsible for the rapid decreasing rate and low swelling capacity of the composites in the CTAB solution. In the anionic surfactant SDBS solution, owing to the repulsion of the negatively charged –COO− groups with the anions of the polymeric chains, the DBS− moieties barely entered the network of the composite.44 Consequently, the superabsorbent composites exhibited a comparatively higher swelling capacity in the anionic surfactant solution than in the cationic surfactant solution.
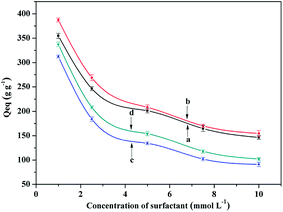 |
| Fig. 12 Effect of different surfactants on the water absorbency of superabsorbent composites: (a) NaAlg-g-PAA/loess and (b) NaAlg-g-PAA/organo-loess composite in various concentrations SDBS solution; (c) NaAlg-g-PAA/loess and (d) NaAlg-g-PAA/organo-loess composites in various concentrations CTAB solution. | |
3.11 Water retention ability
Water retention ability is an important factor for the practical applications of a superabsorbent. The water retention ability of fully swollen NaAlg-g-PAA/loess containing 10% loess and NaAlg-g-PAA/organo-loess containing 10% organo-loess superabsorbent composite at different temperatures (40 and 100 °C) were explored (Fig. 13). The results indicate that the water retention ability had a decreasing tendency with prolonging time, and the water retention curve at 40 °C was flatter than at 100 °C. Moreover, the water retention ability of two types of superabsorbents was very close, and it was more than 69% after 12 h at 40 °C and 100 °C, and could also hold the capacity for approximately 8 h, respectively. This indicates that the superabsorbent composites have excellent water retention ability and are expected to have great potential application for agricultural and horticultural applications, especially for saving water in dry and desert regions.
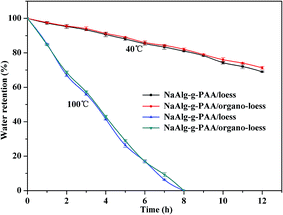 |
| Fig. 13 Water retention of the swollen samples as a function of time at 40 and 100 °C, respectively. | |
4. Conclusions
A novel organic–inorganic superabsorbent composite was successfully synthesized by incorporating organo-loess or loess into a NaAlg-g-PAA polymeric network in an aqueous solution. The FTIR and XRD confirmed that AA had been grafted onto NaAlg backbones and that organo-loess and loess participated in a co-polymerization reaction. SEM and element mapping observation revealed that the surface structure of the superabsorbent composites improved and organo-loess led to a better dispersion. TGA and DSC results indicated that the incorporation of loess improved the thermal stability of the superabsorbent. The superabsorbent composites exhibited excellent water absorbencies and water retention abilities, and the maximum equilibrium water absorbency in distilled water and 0.9 wt% NaCl aqueous solutions were 656 g g−1 and 69 g g−1, respectively. Swelling measurements indicated that the superabsorbent composites had good buffer ability to external pH in the range from 4 to 10, and higher swelling capacity in anionic surfactant (SDBS) than that of cationic surfactant (CTAB) solution. The impact of external saline solutions on the swelling capacity has the following order: Na+ < Ca2+ < Fe3+. Based on the abovementioned description, we can conclude that the incorporation of a suitable amount of loess or organo-loess into the NaAlg-g-PAA polymeric network could not only enhance the swelling capacity of the composites but also improve the buffer ability to the external pH, and the salt-resistant ability of doped with organo-loess is more obvious compared with that of doped with loess, which is an effective approach to improve the performance and extend the application of the superabsorbent composite to drug delivery, hygienic products, agriculture and horticulture.
Acknowledgements
We thank the National Natural Science Foundation of China (no. 21164009), the program for Changjiang Scholars and the Innovative Research Team in University (IRT1177), the Key Laboratory of Eco-Environment-Related Polymer Materials (Northwest Normal University) of Ministry of Education, and the Key Laboratory of Polymer Materials of the Gansu Province.
References
- M. Zhang, Z. Cheng, M. Liu, Y. Zhang, M. Hu and J. Li, J. Appl. Polym. Sci., 2014, 131 DOI:10.1002/APP.40471.
- M. R. Guilherme, A. V. Reis, A. T. Paulino, T. A. Moia, L. H. Mattoso and E. B. Tambourgi, J. Appl. Polym. Sci., 2010, 117, 3146–3154 CAS.
- R. Meena, M. Chhatbar, K. Prasad and A. K. Siddhanta, Polym. Int., 2008, 57, 329–336 CrossRef CAS PubMed.
- G. Jiang, C. Liu, X. Liu, Q. Chen, G. Zhang, M. Yang and F. Liu, Polymer, 2010, 51, 1507–1515 CrossRef CAS PubMed.
- P. K. Murthy, Y. Murali Mohan, K. Varaprasad, B. Sreedhar and K. Mohana Raju, J. Colloid Interface Sci., 2008, 318, 217–224 CrossRef CAS PubMed.
- T. S. Anirudhan and S. R. Rejeena, J. Appl. Polym. Sci., 2014, 131, 40699–40711 CrossRef PubMed.
- F. Brandl, F. Sommer and A. Goepferich, Biomaterials, 2007, 28, 134–146 CrossRef CAS PubMed.
- X. Wang, S. P. Strand, Y. Du and K. M. Vårum, Carbohydr. Polym., 2010, 79, 590–596 CrossRef CAS PubMed.
- W. Kangwansupamonkon, W. Jitbunpot and S. Kiatkamjornwong, Polym. Degrad. Stab., 2010, 95, 1894–1902 CrossRef CAS PubMed.
- Y. Zheng and A. Wang, J. Hazard. Mater., 2009, 171, 671–677 CrossRef CAS PubMed.
- R. Tankhiwale and S. K. Bajpai, Colloids Surf., B, 2009, 69, 164–168 CrossRef CAS PubMed.
- L. Yang, X. Ma, N. Guo and Y. Zhang, Carbohydr. Polym., 2014, 105, 351–358 CrossRef CAS PubMed.
- G. R. Mahdavinia, H. Etemadi and F. Soleymani, Carbohydr. Polym., 2015, 128, 112–121 CrossRef CAS PubMed.
- K. Sharma, V. Kumar, B. S. Kaith, V. Kumar, S. Som, S. Kalia and H. C. Swart, Polym. Degrad. Stab., 2015, 111, 20–31 CrossRef CAS PubMed.
- H. Ferfera-Harrar, N. Aiouaz, N. Dairi and A. S. Hadj-Hamou, J. Appl. Polym. Sci., 2014, 131(1) DOI:10.1002/app.39747.
- W. Wang, J. Zhang and A. Wang, Appl. Clay Sci., 2009, 46, 21–26 CrossRef CAS PubMed.
- Y. Wang, W. Wang, X. Shi and A. Wang, Polym. Bull., 2013, 70, 1181–1193 CrossRef CAS PubMed.
- B. S. Kaith, K. Sharma, V. Kumar, S. Kalia and H. C. Swart, Synth. Met., 2014, 187, 61–67 CrossRef CAS PubMed.
- K. Sharma, V. Kumar, B. S. Kaith, S. Som and V. Kumar, Ind. Eng. Chem. Res., 2015, 54(7), 1982–1991 CrossRef CAS.
- H. Zhu and X. Yao, J. Macromol. Sci., Part A: Pure Appl.Chem., 2013, 50, 175–184 CrossRef CAS PubMed.
- X. Shi, W. Wang and A. Wang, J. Compos. Mater., 2011, 45, 2189–2198 CrossRef CAS PubMed.
- S. Zhang, Y. Guan, G. Q. Fu, B. Y. Chen, F. Peng, C. L. Yao and R. C. Sun, J. Nanomater., 2014, 2 DOI:10.1155/2014/675035.
- T. Wan, Z. Zhou, R. Huang, C. Zou, M. Xu, W. Cheng and R. Li, Appl. Clay Sci., 2014, 101, 199–204 CrossRef CAS PubMed.
- D. Gao, R. B. Heimann, J. Lerchner, J. Seidel and G. Wolf, J. Mater. Sci., 2001, 36, 4567–4571 CrossRef CAS.
- S. Bekin, S. Sarmad, K. Gürkan, G. Keçeli and G. Gürdağ, Sens. Actuators, B, 2014, 202, 878–892 CrossRef CAS PubMed.
- H. S. Samanta and S. K. Ray, Carbohydr. Polym., 2014, 99, 666–678 CrossRef CAS PubMed.
- W. Wang and A. Wang, Carbohydr. Polym., 2010, 80, 1028–1036 CrossRef CAS PubMed.
- J. Wang, Q. Wang, Y. Zheng and A. Wang, Polym. Compos., 2013, 34, 274–281 CrossRef CAS PubMed.
- M. Irani, H. Ismail and Z. Ahmad, J. Appl. Polym. Sci., 2014, 131, 40101–40111 CrossRef PubMed.
- X. Qi, M. Liu, Z. Chen and R. Liang, Polym. Adv. Technol., 2007, 18, 184–193 CrossRef CAS PubMed.
- Q. Tang, J. Lin, J. Wu, Y. Xu and C. Zhang, J. Appl. Polym. Sci., 2007, 104, 735–739 CrossRef CAS PubMed.
- Y. Xie and A. Wang, J. Compos. Mater., 2009, 43, 2401–2417 CrossRef CAS PubMed.
- X. Shi, W. Wang, Y. Kang and A. Wang, J. Appl. Polym. Sci., 2012, 125, 1822–1832 CrossRef CAS PubMed.
- Y. Wang, W. Wang and A. Wang, Chem. Eng. J., 2013, 228, 132–139 CrossRef CAS PubMed.
- S. Menon, M. V. Deepthi, R. R. N. Sailaja and G. S. Ananthapadmanabha, Indian J. Adv. Chem. Sci., 2014, 2(2), 76–83 Search PubMed.
- X. Xia, X. Zeng, J. Liu and W. Xu, J. Appl. Polym. Sci., 2010, 118(4), 2461–2466 CAS.
- Y. Xiang, Z. Peng and D. Chen, Eur. Polym. J., 2006, 42(9), 2125–2132 CrossRef CAS PubMed.
- J. Zhang, H. Chen and A. Wang, Eur. Polym. J., 2005, 41, 2434–2442 CrossRef CAS PubMed.
- J. Zhang, A. Li and A. Wang, Polym. Adv. Technol., 2005, 16, 813–820 CrossRef CAS PubMed.
- Y. Zhou, S. Fu and L. Zhang, Carbohydr. Polym., 2013, 97, 429–435 CrossRef CAS PubMed.
- J. Zhang, H. Chen and A. Wang, Polym. Adv. Technol., 2006, 17, 379–385 CrossRef CAS PubMed.
- T. Caykara and G. Birlik, Macromol. Mater. Eng., 2005, 290, 869–874 CrossRef CAS PubMed.
- Y. M. Mohan, T. Premkumar, D. K. Joseph and K. E. Geckeler, React. Funct. Polym., 2007, 67, 844–858 CrossRef CAS PubMed.
- H. Yang, W. Wang and A. Wang, J. Dispersion Sci. Technol., 2012, 33, 1154–1162 CrossRef CAS PubMed.
|
This journal is © The Royal Society of Chemistry 2015 |
Click here to see how this site uses Cookies. View our privacy policy here.