DOI:
10.1039/C5RA09130F
(Paper)
RSC Adv., 2015,
5, 56470-56477
Stimuli-responsive fluorescence based on the solid-state bis[2-(2-benzothiazoly)phenolato]zinc(II) complex and its fiber thin film†
Received
15th May 2015
, Accepted 18th June 2015
First published on 18th June 2015
Abstract
While metal-based complexes with single-stimulus-responsive fluorescence have been largely developed recently, examples of dual-stimuli-responsive systems are still relatively limited. In this work, a bis[2-(2-benzothiazoly)phenolato]zinc(II) complex was synthesized through a one-step solution process, and the as-prepared solid-state sample exhibited fluorescent emission changes upon dual external stimuli such as mechanical force and pH. In addition, the related luminescent properties (such as wavelength, fluorescence lifetime, color, and two-photon emission) after mechanical- and pH-stimuli can be easily recovered and recycled several times. Moreover, to develop a film material for easy operation, the Zn(BTZ)2 complex was further incorporated into a poly(vinyl alcohol) (PVA) matrix to obtain a microfiber thin film based on an electrospinning process, and the as-fabricated Zn(BTZ)2@PVA system showed changeable luminescence upon heat treatment. These observations confirm that the Zn(BTZ)2 complex is a stimuli-responsive luminescent system sensitive to pH and force external changes in the powder state, and sensitive to temperature changes in the film state. Therefore, compared with the well-developed noble metal-based complexes with stimuli-responsive properties, this work suggests that the Zn-based complex can provide an alternative to develop potential low-cost luminescent sensors and optical antiforgery systems.
1. Introduction
Fluorescent materials responding to changes in external stimuli are of great interest from both scientific and engineering views,1 since these stimuli-responsive fluorescent systems play an important role in the fields of sensors, security papers, optoelectronic switches, and information storage.2 During the last few years, fluorescent materials sensitive to a single external stimulus (such as pH-,3,4 light-,5 mechanical6 and temperature4,5,7 etc.) have been continuously reported, which have greatly promoted the progress of single-stimulus-responsive materials. However, the development of dual- or multiple-stimuli responsive luminescence remains in an early stage, and examples are relative rare compared with those of single-responsive systems.4,8,9 Moreover, before these stimuli-responsive materials in the solution10 and powdered forms11,12 can be used as smart devices, it is highly desirable to obtain their thin film systems to achieve facile fabrication and easy operation.9,13
Metal-based photofunctional complexes (PC) have received a great deal of attention during the last few decades, because of their chemical stability and high luminescence yields, which have been largely used in the photonic and optoelectronic fields.14 Recently, several PC materials also present interesting stimuli-responsive fluorescent properties,11,15 which have offered them great opportunities as luminescent sensors and switches.16 Zn-organic based complex materials are an important type of PC systems,17 which present well-defined luminescent properties, and have been extensively investigated as solid-state light-emitting devices.18 However, except for the previous work of Mizukami et al.,19 very little attention has been focused on the stimuli-responsive luminescent characteristics of Zn-based PC materials; moreover, compared with the well-documented noble metal complexes (such as Au(I),20 Ag(I),21 Pt(II),22 Ir(III),23 etc.) with stimuli-responsive luminescent properties, the development of noble-metal-free stimuli-responsive PC materials is highly desirable to achieve their low-cost and effective utilization in the next generation of photofunctional materials.
Bis[2-(2-benzothiazoly)phenolato]zinc(II) (Zn(BTZ)2) is a promising Zn-based PC system, which has been studied in the fields of light-emitting diodes (LEDs) and electrochemistry due to its excellent photonic and electronic properties.24 For example, Zn(BTZ)2 has been used as a white electroluminescence (EL) material. Zhu et al.24b have reported that LED was fabricated with a simple bilayer structure: ITO/N,N′-bis(3-methylphenyl)-N,N′-bis(phenyl)benzidine (TPD)/Zn(BTZ)2/Al. White emission is composed of two parts: one is 470 nm, which originates from exciton emission in Zn(BTZ)2 emitting layer; the other is 580 nm, which originates from exciplexes formation at the interface of TPD and Zn(BTZ)2. Yu et al.24e have studied the electronic structures of Zn(BTZ)2 by using quantum chemical calculations. The highest occupied molecular orbitals of [Zn(BTZ)2]2 units are mainly localized on two non-bridging ligands, whereas the lowest unoccupied molecular orbitals are localized predominantly on two bridging ligands. The results showed that [Zn(BTZ)2]2 appeared to have a better electron-transport property than that of the well-established electron transporter 8-hydroxyquinoline aluminum salt. However, to the best of our knowledge, detailed stimuli-responsive fluorescent properties of this compound have not been detected. In this work, we have synthesized Zn(BTZ)2 complex based on a one-step solution process. It was found that the as-prepared solid-state Zn-based complex presents reversible mechanochromic luminescence, and the two emissive states can also be revealed in their up-conversion fluorescent spectra. The reversible pH-sensitive fluorescence of Zn(BTZ)2 have also been investigated. Moreover, an electrospinning technology has been employed to fabricate continuous micro-sized fibers by incorporation of Zn(BTZ)2 into a poly(vinyl alcohol) (PVA) matrix, which can be further directly assembled into flexible thin film. The thin film exhibited fluorescent red-shift upon heating at 50 °C. Therefore, this work developed new type of stimuli-responsive luminescent system based on a low-cost Zn-based PC, which has incorporated its advantages as potential luminescent sensors towards external mechanical force, pH value and heat.
2. Results and discussion
The Zn(BTZ)2 complex (Scheme 1) was synthesized based on the previous work of Hamada et al.25 The increase of the concentration of the Zn(BTZ)2 solution has no obvious influence on the emissive properties (Fig. S1 in ESI†); the typical fluorescence spectra of both the pristine solution and solid-state powder of Zn(BTZ)2 are located at ca. 464 nm as shown in Fig. 1A, suggesting that the stacking fashion of the Zn(BTZ)2 complexes within the solid is highly isolated with each other. This conclusion is also consistent well with the crystal structure of the Zn(BTZ)2 complex as shown in Fig. 1B. In addition, the XRD pattern of the as-prepared solid-state powder fitted well with that simulated from crystal structure of Zn(BTZ)2 complex (Fig. 1C). The lack of other XRD reflections confirms the high purity of the product.
 |
| Scheme 1 Synthetic route of the Zn(BTZ)2. | |
 |
| Fig. 1 (A) The fluorescence spectra of Zn(BTZ)2 solid and solution in methanol (10−5 mol L−1); (B) crystal structure of Zn(BTZ)2; (C) the comparison between experimental and simulated XRD patterns. | |
The Zn(BTZ)2 complex has a good photostability. The fluorescence emissive wavelength has no change upon exposed to visible or ultraviolet light. Interestingly, it was found that the Zn(BTZ)2 showed fluorescent red-shift to 481 nm upon grinding the solid powder in a mortar just for 15 seconds, accompanied by the increase of the intensity (Fig. 2A). The easy identification of the fluorescence color change can also be detected by the naked eye (shown in the inset of Fig. 2A). Furthermore, the emissive wavelength can be recovered by treating Zn(BTZ)2 using the methanol vapor. The reversible change in luminescence emissive wavelength (λmaxem) of this process can be readily repeated for several times (Fig. 2B).
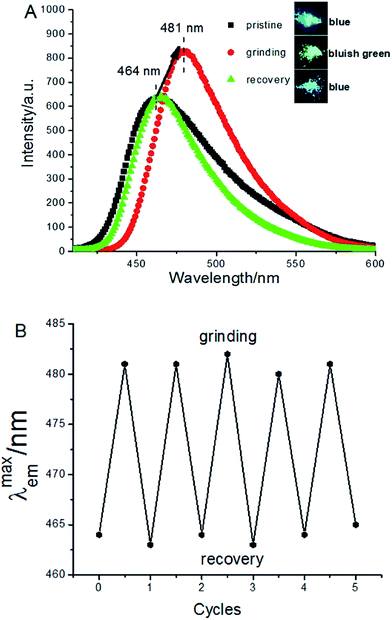 |
| Fig. 2 The fluorescence spectra of the Zn(BTZ)2 solid before and after grinding over one cycle (A), and the reversible fluorescence responses (λmaxem) over 5 consecutive cycles (B). | |
To better understand the excitation state properties of the Zn(BTZ)2 powders before and after grinding, fluorescence lifetime was measured, and the typical fluorescence decay curve before and after grinding was shown in Fig. 3A. It was noted that the fluorescence decay became relative faster after grinding treatment. The corresponding fluorescence lifetime has a decrease trend from 2.75 ns (before) to 2.34 ns (after). The change in fluorescence lifetime is also repeatedly observed on cycling the samples by grinding and methanol vapor treatment (Fig. 3B). To further understand the change in fluorescent properties from a crystal stacking view, XRD measurement was performed on the sample before and after grinding (shown in Fig. 3C). It was observed that there is no obvious change in the positions of the reflection peaks, except that the relative intensity of the characteristic peaks assigned to the (210), (1−21) and (11−2) has an increasing trend. This observation may suggest that a slight alternation of the relative orientation occurs for the solid-state Zn(BTZ)2, and thus results in the change of fluorescence. Additionally, Cd(BTZ)2 was also synthesized based on the same method, but it was found that Cd(BTZ)2 was lack of the grinding-induced luminescent behavior (Fig. 2 in ESI†), indicating the special role of Zn(II) ion during the stimulus-responsive process.
 |
| Fig. 3 (A) The fluorescence decay curves of the Zn(BTZ)2 solid before and after grinding (IRF: instrumental response function), (B) the reversible fluorescence lifetime over 5 consecutive cycles; (C) the XRD patterns before and after grinding of the Zn(BTZ)2 solid as well as the recovery state. | |
Because BTZ-containing compounds may serve as two-photon absorption and emissive chromophores, up-conversion fluorescence measurements were further made on the Zn(BTZ)2 powders. Upon irradiation by a femtosecond pulse laser at 800 nm, Zn(BTZ)2 complex showed a strong up-conversion fluorescence (Fig. 4A) with the emission peak at ca. 464 nm. There is no obvious red- or blue-shift emission compared with those excited at 365 nm UV light, suggesting that the emission for one-photon and two-photon excited states to the ground state may involve a same process. Upon grinding, the up-conversion emission for the Zn(BTZ)2 present a red-shift with the fluorescence peak located at 484 nm (Fig. 4B), and this is consistent with the trend for that excited by UV light. Moreover, the log values of the intensity have a good linear relationship with the log values of incident energy, with the slope close to 2, further confirming that the emissive processes in the two fluorescent states before and after grinding involve a two-photon mechanism.26
 |
| Fig. 4 Fluorescence spectra of powdered Zn(BTZ)2 excited by an 800 nm laser under different pump powers before (A) and after (B) grinding treatment. | |
The Zn(BTZ)2 sample also present pH-sensitive fluorescence emission properties. By treating the Zn(BTZ)2 solid powders at both high and low pH conditions, the fluorescence present red-shift emission. Compared with the Zn(BTZ)2 with the λmaxem located at 464 nm, the sample showed the emission at 481 nm and 509 nm when it was treated by adding a drop of ammonia water (28%, pH = 12) and hydrochloric acid (0.36 g L−1, pH = 2) respectively. Such behaviors may be related to that the H+ and OH− can potentially interact with both the BTZ ligand and central Zn cation, which can further tailor the electronic structures of the Zn(BTZ)2 compound.27 The corresponding color change of the Zn(BTZ)2 can also be easily identified as shown in the inset of Fig. 5A. The pH-responsive emission of the Zn(BTZ)2 at the typical pH conditions (7 and 12) can be recycled for several times as shown in Fig. 5B.
 |
| Fig. 5 The fluorescence spectra of the Zn(BTZ)2 solid before and after treated at different pH conditions (pH = 2, 7 and 12), and the reversible fluorescence responses (λmaxem) over 5 consecutive cycles (B) at pH = 7 and 12. | |
To produce a useable film material, Zn(BTZ)2 complex was incorporated into a PVA matrix to form flexible self-supporting Zn(BTZ)2@PVA film by means of the electrospinning method, and the typical process is illustrated as shown in Fig. 6A. XRD for the Zn(BTZ)2@PVA showed no diffraction peaks from either Zn(BTZ)2 or PVA, suggesting the formation of an amorphous composite material (Fig. 6B). FT-IR spectra (Fig. 6C) showed that the Zn(BTZ)2@PVA system maintains the vibration peaks of the Zn(BTZ)2 without obvious shift, suggesting that the Zn(BTZ)2 units are highly dispersed within the PVA matrix. To probe the morphological features of the electrospinning film, Zn(BTZ)2@PVA system was further studied by the scanning electron microscopy (SEM). It can be observed that the film is constructed from fibers with a width of ca. 2.8 μm (Fig. 7A). Each individual microfiber has an extremely high aspect ratio and length, and the fiber surface is continuous and homogeneous (Fig. 7B). The fiber films feature dense 3D networks composed of different orientations of fiber aggregates, indicating the 1D fiber microstructures undergo self-organization and intertwining processes to afford macro-sized films. Such film morphology is close to that of the pristine electrospinning PVA film (Fig. S3 in ESI†).
 |
| Fig. 6 (A) Schematic illustration of the incorporation of Zn(BTZ)2 into PVA fibers using an electrospinning method, (B) XRD pattern of the Zn(BTZ)2@PVA fiber, and (C) FT-IR spectra of Zn(BTZ)2, PVA and Zn(BTZ)2@PVA. | |
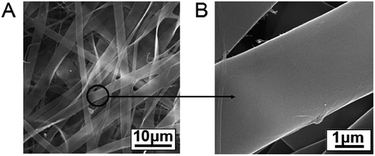 |
| Fig. 7 SEM images (A and B) of the microfibers with different magnifications. | |
It was found that the as-prepared electrospinning film present heat-responsive fluorescence behavior: for the pristine film, the fluorescence is mainly located at around 460 nm, accompanying with a shoulder red-shift peak at 490 nm. By heating the film at 50 °C for 10 minutes, there is an obvious fluorescence red-shift with the main peak occurring at 506 nm. The obvious fluorescence color change is clearly imaged as shown in the inset of Fig. 8A. The fluorescence change of the micro-sized fibers can also be easily detected under a UV fluorescence microscope, which showed a similar color alternation relative to that of the film (Fig. 8B). In addition, the fluorescence lifetime has also reduced after the thermal treatment from 3.95 ns to 3.22 ns (Fig. 9). We have tried to find effective ways to achieve the reversible transfer of the fluorescence, but the changed fluorescence cannot be recovered currently. Although the lack of reversibility for this heat-sensitive system, the Zn(BTZ)2@PVA film can still find the potential applications in fluorescent antiforgery field and/or as history recorder. This is because there is no need to achieve a fluorescence recovering properties for some of the fluorescent antiforgery materials.
 |
| Fig. 8 (A) The fluorescence spectra of the Zn(BTZ)2@PVA before and after thermal treatment; (B) the fluorescence images of the Zn(BTZ)2@PVA microfibers under a UV fluorescence microscopy. | |
 |
| Fig. 9 The fluorescence decay curves of the Zn(BTZ)2@PVA microfibers before and after heat treatment (IRF: instrumental response function). | |
3. Conclusions
In summary, the as-prepared Zn(BTZ)2 complex was found to present both mechanical- and pH-responsive fluorescent behaviors. The related properties (such as fluorescence lifetime, color, and two-photon emission) can be easily recovered by the methanol vapor. These observations confirm the Zn-based complex is a reversible dual-stimuli-responsive luminescent switch toward the external changes in the force and pH conditions. Moreover, based on an electrospinning process, Zn(BTZ)2 complex was incorporated into a PVA matrix to obtain microfiber thin film, which showed changeable luminescence upon heat treatment. Therefore, combination of mechanical, pH and heat as external environments, the Zn(BTZ)2 complex and its thin film can serve as promising stimuli-responsive fluorescent materials. Moreover, due to the low cost and easy preparation, this Zn-based complex may supply an effective way to develop potential luminescent sensor and optical antiforgery system.
4. Experimental section
4.1 Reagents and materials
2-(2-Hydroxyphenyl)benzothiazole and zinc acetate were obtained from Sigma Chemical Co. Ltd., and used without further purification. Polvinyl alcohol (PVA) (Mr = 77
000, 99% hydrolyzed) and hexafluoroisopropanol were purchased from Beijing Chemical Co. Ltd. and used without further purification.
Synthesis of bis[2-(2-benzothiazolyl)phenolato]zinc(II) (Zn(BTZ)2) was prepared as follows: typically, 5 mmol of 2-(2-hydroxyphenyl)benzothiazole was dissolved into 100 mL of methanol, 8 mmol of Zn(OOCCH3)2·2H2O was dissolved into 80 mL of methanol. The Zn(OOCCH3)2 solution was dropwise added into the 2-(2-hydroxyphenyl)benzothiazole solution, which was further stirred at 75 °C. After 3 h, the yellow precipitate can be obtained, which was further deposited and collected by centrifugation.
4.2 Preparation of electrospinning solutions
Typically, 0.5 g of PVA was first dispersed into 15 mL of hexafluoroisopropanol for 5 hour to obtain a PVA solution. Then, 0.09 g of Zn(BTZ)2 were added into the PVA solution under vigorous stirring. The resulting clear homogenous solution was used for electrospinning and the fabrication of the Zn(BTZ)2@PVA films (mass concentrations: 18 wt%).
4.3 Electrospinning and casting films
A burette with an inserted Cu rod connected to a high-voltage supply was filled with the Zn(BTZ)2@PVA aqueous solution. An Al sheet connected to the ground was used as the receiver. The distance between the burette tip and receiver was fixed at 17 cm and the high-voltage supply was fixed at 22 kV. The spinning rate was controlled at about 4.5 mL h−1 by adjusting the angle of inclination of the burette. Pieces of Al sheet about 30 cm × 30 cm was placed on the Al sheet for collecting the samples. Then, 0.5 mL of the composite solution was dropped on a clean Al sheet and left to dry under ambient conditions to form thin films.
4.4 Sample characterization
The fluorescence spectra were recorded on RF-5301PC fluorospectrophotometer with an excitation wavelength of 365 nm, with the excitation and emission slits both set to 3.0 nm. The fluorescence decays were measured using LifeSpec-ps spectrometer with a 372 nm laser exciting the films, and the fluorescence lifetimes were calculated with the F900 Edinburgh instruments software. The XRD patterns of the samples were collected on a Shimadzu XRD-6000 diffractometer (40 kV, 30 mA, graphite-filtered CuKα radiation, λ = 0.15418 nm). The morphology of thin films was investigated by using a scanning electron microscope (SEM Zeiss Supra 55) equipped with an EDX attachment, and the accelerating voltage applied was 20 kV. The surface roughness and thickness data were obtained using a Bruker Multimode 8 atomic force microscope (AFM). Two-photon excited fluorescence of the samples was excited by 800 nm laser on a Tsunami-Spitfire-OPA-800C ultrafast optical parameter amplifier (Spectra Physics). The fluorescence images were obtained on an Olympus U-RFLT50 fluorescence microscope.
Acknowledgements
This work was supported by the 973 Program (Grant no. 2014CB932103), the National Natural Science Foundation of China (NSFC), and Beijing Municipal Natural Science Foundation (Grant no. 2152016).
Notes and references
-
(a) Y. Chang, Y. Li, S. Yu, J. Mao, C. Liu, Q. Li, C. Yuan, N. He, W. Luo and L. Dai, Nanotechnology, 2015, 26, 1 CrossRef PubMed;
(b) M. H. Lim and S. J. Lippard, Acc. Chem. Res., 2007, 40, 41 CrossRef CAS PubMed;
(c) C. R. Maldonado, L. Salassa, N. Gomez-Blanco and J. C. Mareque-Rivas, Coord. Chem. Rev., 2013, 257, 2668 CrossRef CAS PubMed;
(d) J. Bu, K. Watanabe, H. Hayasaka and K. Akagi, Nat. Commun., 2014, 5, 3799 CAS;
(e) S. Giri, B. G. Trewyn, M. P. Stellmaker and V. S. Lin, Angew. Chem., Int. Ed., 2005, 44, 5038 CrossRef CAS PubMed;
(f) D. Wang, T. Liu, J. Yin and S. Liu, Macromolecules, 2011, 44, 2282 CrossRef CAS;
(g) R. B. Vasani, S. J. McInnes, M. A. Cole, A. M. Jani, A. V. Ellis and N. H. Voelcker, Langmuir, 2011, 27, 7843 CrossRef CAS PubMed;
(h) Z. Chi, X. Zhang, B. Xu, X. Zhou, C. Ma, Y. Zhang, S. Liu and J. Xu, Chem. Soc. Rev., 2012, 41, 3878 RSC.
-
(a) L. Ionov, S. Sapra, A. Synytska, A. L. Rogach, M. Stamm and S. Diez, Adv. Mater., 2006, 18, 1453 CrossRef CAS PubMed;
(b) A. Balan, D. Baran, G. Gunbas, A. Durmus, F. Ozyurt and L. Toppare, Chem. Commun., 2009, 6768 RSC;
(c) A. Royon, K. Bourhis, M. Bellec, G. Papon, B. Bousquet, Y. Deshayes, T. Cardinal and L. Canioni, Adv. Mater., 2010, 22, 5282 CrossRef CAS PubMed;
(d) M. Zambianchi, L. Favaretto, M. Durso, C. Bettini, A. Zanelli, I. Manet, M. Gazzano, L. Maini, D. Gentili, S. Toffanin, F. Gallino, M. Muccini, M. Cavallini and M. Melucci, J. Mater. Chem. C, 2015, 3, 121 RSC.
-
(a) H. S. Park, J. E. Lee, M. Y. Cho, Y. W. Noh, M. H. Sung, H. Poo, K. S. Hong and Y. T. Lim, Nanotechnology, 2011, 22, 465603 CrossRef PubMed;
(b) Y. Zhang, Q. Song, K. Wang, W. Mao, F. Cao, J. Sun, L. Zhan, Y. Lv, Y. Ma, B. Zou and C. Zhang, J. Mater. Chem. C, 2015, 3, 3049 RSC.
- S. Su, J. Wei, K. Zhang, J. Qiu and S. Wang, Colloid Polym. Sci., 2015, 293, 1299 CAS.
-
(a) B. M. Budhlall, M. Marquez and O. D. Velev, Langmuir, 2008, 24, 11959 CrossRef CAS PubMed;
(b) X. Yan and X. An, Nanoscale, 2013, 5, 6280 RSC;
(c) R. Gao, D. Cao, Y. Guan and D. Yan, ACS Appl. Mater. Interfaces, 2015, 7, 9904 CrossRef CAS PubMed.
-
(a) H. Ito, T. Saito, N. Oshima, N. Kitamura, S. Ishizaka, Y. Hinatsu, M. Wakeshima, M. Kato, K. Tsuge and M. Sawamura, J. Am. Chem. Soc., 2008, 130, 10044 CrossRef CAS PubMed;
(b) D. Yan, H. Yang, Q. Meng, H. Lin and M. Wei, Adv. Funct. Mater., 2014, 24, 587 CrossRef CAS PubMed.
-
(a) T. Han, X. Feng, J. Shi, B. Tong, Y. Dong, J. W. Y. Lam, Y. Dong and B. Z. Tang, J. Mater. Chem. C, 2013, 1, 7534 RSC;
(b) X. Zhao, S. Zhu, Y. Song, J. Zhang and B. Yang, RSC Adv., 2015, 5, 15187 RSC;
(c) D. P. Yan, J. Lu, J. Ma, M. Wei, D. G. Evans and X. Duan, Angew. Chem., Int. Ed., 2011, 50, 720 CrossRef CAS PubMed;
(d) X. Chen, H. Jiang, Y. Wang, G. Zou and Q. Zhang, Mater. Chem. Phys., 2010, 124, 36 CrossRef CAS PubMed.
-
(a) G. Wu, S. C. Chen, C. L. Liu and Y. Z. Wang, ACS Nano, 2015, 9, 4649 CrossRef CAS PubMed;
(b) L. Zhao, W. Li, A. Plog, Y. Xu, G. Buntkowsky, T. Gutmann and K. Zhang, Phys. Chem. Chem. Phys., 2014, 16, 26322 RSC.
- C. G. Schäfer, M. Gallei, J. T. Zahn, J. Engelhardt, G. P. Hellmann and M. Rehahn, Chem. Mater., 2013, 25, 2309 CrossRef.
-
(a) J. Hu, C. Li and S. Liu, Langmuir, 2010, 26, 724 CrossRef CAS PubMed;
(b) J. Deng, P. Yu, Y. Wang and L. Mao, Anal. Chem., 2015, 87, 3080 CrossRef CAS PubMed;
(c) Y. Sagara, T. Komatsu, T. Ueno, K. Hanaoka, T. Kato and T. Nagano, Adv. Funct. Mater., 2013, 23, 5277 CrossRef CAS PubMed;
(d) A. Balamurugan and H. I. Lee, Macromolecules, 2015, 48, 1048 CrossRef CAS.
- T. Tsukuda, M. Kawase, A. Dairiki, K. Matsumoto and T. Tsubomura, Chem. Commun., 2010, 46, 1905 RSC.
-
(a) N. Kameta, M. Masuda and T. Shimizu, ACS Nano, 2012, 6, 5249 CrossRef CAS PubMed;
(b) N. Zhao, M. Li, Y. Yan, J. W. Y. Lam, Y. L. Zhang, Y. S. Zhao, K. S. Wong and B. Z. Tang, J. Mater. Chem. C, 2013, 1, 4640 RSC.
-
(a) S. Kim, S. J. Yoon and S. Y. Park, J. Am. Chem. Soc., 2012, 134, 12091 CrossRef CAS PubMed;
(b) H. Ma, R. Gao, D. Yan, J. Zhao and M. Wei, J. Mater. Chem. C, 2013, 1, 4128 RSC.
-
(a) P. Paoprasert, J. E. Laaser, W. Xiong, R. A. Franking, R. J. Hamers, M. T. Zanni, J. R. Schmidt and P. Gopalan, J. Phys. Chem. C, 2010, 114, 9898 CrossRef CAS;
(b) W. Y. Wong and C. L. Ho, Coord. Chem. Rev., 2006, 250, 2627 CrossRef CAS PubMed;
(c) W. Y. Wong, Coord. Chem. Rev., 2005, 249, 971 CrossRef CAS PubMed;
(d) B. Schlicke, P. Belser, L. De Cola, E. Sabbioni and V. Balzani, J. Am. Chem. Soc., 1999, 121, 4207 CrossRef CAS.
-
(a) G. G. Shan, H. B. Li, H. T. Cao, H. Z. Sun, D. X. Zhu and Z. M. Su, Dyes Pigm., 2013, 99, 1082 CrossRef CAS PubMed;
(b) B. C. Tzeng, T. Y. Chang and H. S. Sheu, Chem.–Eur. J., 2010, 16, 9990 CrossRef CAS PubMed.
-
(a) K. J. Franz, N. Singh, B. Spingler and S. J. Lippard, Inorg. Chem., 2000, 39, 4081 CrossRef CAS;
(b) M. L. Cable, J. P. Kirby, H. B. Gray and A. Ponce, Acc. Chem. Res., 2013, 46, 2576 CrossRef CAS PubMed;
(c) X. Su and I. Aprahamian, Chem. Soc. Rev., 2014, 43, 1963 RSC;
(d) S. Blair, M. P. Lowe, C. E. Mathieu, D. Parker, P. K. Senanayake and R. Kataky, Inorg. Chem., 2001, 40, 5860 CrossRef CAS PubMed;
(e) X. Zhang, Z. Chi, Y. Zhang, S. Liu and J. Xu, J. Mater. Chem. C, 2013, 1, 3376 RSC.
-
(a) L. Chen and B. Yan, Dalton Trans., 2014, 43, 14123 RSC;
(b) M. Fujitsuka, H. Shimakoshi, Y. Tei, K. Noda, S. Tojo, Y. Hisaeda and T. Majima, Phys. Chem. Chem. Phys., 2013, 15, 5677 RSC.
-
(a) Y. Huo, J. Lu, T. Lu, X. Fang, X. Ouyang, L. Zhang and G. Yuan, New J. Chem., 2015, 39, 333 RSC;
(b) K. Wang, F. Zhao, C. Wang, S. Chen, D. Chen, H. Zhang, Y. Liu, D. Ma and Y. Wang, Adv. Funct. Mater., 2013, 23, 2672 CrossRef CAS PubMed;
(c) S.-G. Roh, Y.-H. Kim, K. D. Seo, D. H. Lee, H. K. Kim, Y.-I. Park, J.-W. Park and J.-H. Lee, Adv. Funct. Mater., 2009, 19, 1663 CrossRef CAS PubMed.
-
(a) S. Mizukami, S. Okada, S. Kimura and K. Kikuchi, Inorg. Chem., 2009, 48, 7630 CrossRef CAS PubMed;
(b) N. Wakiya, K. Shimizu, S. Mizukami, K. Shinozaki and N. Mizutani, Appl. Phys. Lett., 2004, 85, 3772 CrossRef CAS PubMed.
-
(a) S. H. Lim, J. C. Schmitt, J. Shearer, J. Jia, M. M. Olmstead, J. C. Fettinger and A. L. Balch, Inorg. Chem., 2013, 52, 823 CrossRef CAS PubMed;
(b) Z. Wu and R. Jin, Nano Lett., 2010, 10, 2568 CrossRef CAS PubMed;
(c) Q. Zhao, F. Li and C. Huang, Chem. Soc. Rev., 2010, 39, 3007 RSC;
(d) A. Kishimura, T. Yamashita and T. Aida, J. Am. Chem. Soc., 2005, 127, 179 CrossRef CAS PubMed.
-
(a) Y. B. Dong, G. X. Jin, M. D. Smith, R. Q. Huang, B. Tang and H. C. zur Loye, Inorg. Chem., 2002, 41, 4909 CrossRef CAS PubMed;
(b) Y. Kang, C. Seward, D. Song and S. Wang, Inorg. Chem., 2003, 42, 2789 CrossRef CAS PubMed;
(c) V. J. Catalano and S. J. Horner, Inorg. Chem., 2003, 42, 8430 CrossRef CAS PubMed;
(d) K. Matsumoto, T. Shindo, N. Mukasa, T. Tsukuda and T. Tsubomura, Inorg. Chem., 2010, 49, 805 CrossRef CAS PubMed.
-
(a) R. P. Brinas, T. Troxler, R. M. Hochstrasser and S. A. Vinogradov, J. Am. Chem. Soc., 2005, 127, 11851 CrossRef CAS PubMed;
(b) V. W. W. Yam, K. M. C. Wong and N. Zhu, J. Am. Chem. Soc., 2002, 124, 6506 CrossRef CAS PubMed;
(c) T. J. Wadas, Q. M. Wang, Y. J. Kim, C. Flaschenreim, T. N. Blanton and R. Eisenberg, J. Am. Chem. Soc., 2004, 126, 16841 CrossRef CAS PubMed.
-
(a) S. Lamansky, P. Djurovich, D. Murphy, F. Abdel-Razzaq, H. E. Lee, C. Adachi, P. E. Burrows, S. R. Forrest and M. E. Thompson, J. Am. Chem. Soc., 2001, 123, 4304 CrossRef CAS PubMed;
(b) P. Ivanov, R. Tomova, P. Petrova, S. Stanimirov and I. Petkov, J. Phys.: Conf. Ser., 2014, 514, 012038 CrossRef;
(c) S. Lamansky, P. Djurovich, D. Murphy, F. Abdel-Razzaq, R. Kwong, I. Tsyba, M. Bortz, B. Mui, R. Bau and M. E. Thompson, Inorg. Chem., 2001, 40, 1704 CrossRef CAS PubMed.
-
(a) X. Huixia, X. Bingshe, F. Xiaohong, C. Liuqing, W. Hua and H. Yuying, J. Photochem. Photobiol., A, 2011, 217, 108 CrossRef PubMed;
(b) F. J. Zhu, Y. L. Hua, S. G. Yin, J. C. Deng, K. W. Wu, X. Niu, X. M. Wu and M. C. Petty, J. Lumin., 2007, 122–123, 717 CrossRef CAS PubMed;
(c) Z. Li, A. Dellali, J. Malik, M. Motevalli, R. M. Nix, T. Olukoya, Y. Peng, H. Ye, W. P. Gillin, I. Hernandez and P. B. Wyatt, Inorg. Chem., 2013, 52, 1379 CrossRef CAS PubMed;
(d) R. Wang, L. Deng, M. Fu, J. Cheng and J. Li, J. Mater. Chem., 2012, 22, 23454 RSC;
(e) G. Yu, S. Yin, Y. Liu, Z. Shuai and D. Zhu, J. Am. Chem. Soc., 2003, 125, 14816 CrossRef CAS PubMed;
(f) X. M. Wu, Y. L. Hua, Z. Q. Wang, J. J. Zheng, X. L. Feng and Y. Y. Sun, Chin. Phys. Lett., 2005, 22, 1797 CrossRef CAS;
(g) J. T. Lim, N. H. Lee, Y. J. Ahn, G. W. Kang and C. H. Lee, Curr. Appl. Phys., 2002, 2, 295 CrossRef.
- Y. Hamada, T. Sano, H. Fujii, Y. Nishio, H. Takahashi and K. Shibata, Jpn. J. Appl. Phys., 1996, 35, L1339 CrossRef CAS.
- R. Gao, M. J. Zhao, Y. Guan, X. Y. Fang, X. H. Li and D. P. Yan, J. Mater. Chem. C, 2014, 2, 9579 RSC.
- W. Zhang, Y. L. Yan, J. M. Gu, J. N. Yao and Y. S. Zhao, Angew. Chem., Int. Ed., 2015, 54, 7125 CrossRef CAS PubMed.
Footnotes |
† Electronic supplementary information (ESI) available. See DOI: 10.1039/c5ra09130f |
‡ The two authors contribute equal to this work. |
|
This journal is © The Royal Society of Chemistry 2015 |
Click here to see how this site uses Cookies. View our privacy policy here.