DOI:
10.1039/C5RA10002J
(Paper)
RSC Adv., 2015,
5, 65546-65553
Effect of a novel compound as dietary supplement on growth of decapod crustaceans†
Received
27th May 2015
, Accepted 20th July 2015
First published on 20th July 2015
Abstract
Several conventional methods have been used for many years to accelerate the growth in decapod crustaceans but they are not suitable for aquaculture practices due to their harmful effects on the animals. Concern over water quality, environmental hazards, human health and food safety has led us to search for alternative targets, to design growth enhancers which can significantly improve the growth of crustaceans without harmful effects. Among such compounds is CGE-1, an amine compound which has shown excellent molt inducing property. Lobsters treated with CGE-1 exhibited a higher weight gain and greater increment in the carapace length post-molt as compared to the controls even without inhibiting the synthesis and secretion of molt-inhibiting hormone (MIH). This is a significant finding considering the fact that MIH binds specifically to the membrane receptor of y-organs, hence CGE-1 might interfere with this interaction allowing an incessant production of 20-hydroxyecdysone (20E). Here, we summarize our findings on CGE-1 and its effects with particular focus on inclusive growth and vitellogenesis. Our observations and findings suggest that CGE-1 could be a useful growth enhancer that could be used either alone or as a dietary supplement for the rapid growth of decapod crustaceans.
1. Introduction
Antimicrobial agents have been used as an essential part of feed to improve and accelerate livestock production for several years.1 The use of antibiotics at very high concentration specifically in aquaculture has become the driving force for the development of drug resistance microorganisms. There are several incidences of infections caused by these multi-drug resistant (MDR) strains associated with livestock and also on the increase of use of antibiotics in the therapy. These issues also concomitantly cause an economic cost to the production of aquaculture related animals.2,3 Therefore, there is a strong need to better understand the regulatory pathway associated with the growth and development of production animals. The design and development of growth promoters or competitive inhibitors that can cause rapid growth is a relatively rare occurrence. However, there is great interest in research on such growth promoting agents, not only because they might be remarkably effective to promote the growth of various economically important species without causing any adverse effect, but also because they can be useful probes to understand the hormonal regulation of various physiological processes.
Among such economically important marine species, decapod crustaceans specifically play a vital role in the aquaculture and fisheries sector. Hence, understating their physiology can provide significant insights for the development of novel growth enhancers. In decapod crustaceans, the regulation of growth is governed by molt inhibiting hormone, a neurohormone from the crustacean hyperglycemic hormone (CHH) family, synthesized and secreted from the x-organ/sinus gland complex in the eyestalk. CHH family neurohormones form extremely interactive systems to accomplish the integrated function of various physiological processes for decapod crustaceans.4,5 A critical role in the ecdysteroid production is played by a key neuropeptide, known as molt inhibiting hormone. Molt inhibiting hormone is a single-chain 113 amino acid proteohormone composed of a 35 residue signal peptide and a 78 residue mature MIH which play a key role in the regulation of growth and reproduction.6,7 Its amino acid sequence exhibits homologies to other neurohormones including crustacean hyperglycemic hormone and vitellogenin inhibiting hormone and hence is designated as belonging to the CHH family neuropeptides.8 MIH is produced and secreted by the x-organ/sinus gland complex, which acts on the endocrine system, in particular the y-organs, suppressing the production of 20-hydroxyecdysone.9 Therefore, eyestalk ablation leads to an upsurge in the hemolymph ecdysteroid titer and shortening of the intermolt period, whereas eye-stalk ablated animals injected with crude eyestalk extract show significantly lower ecdysteroid level and delayed molting.10 Additionally, in vitro studies have also revealed that recombinant MIH and crude eyestalk extracts inhibit 20-hydroxyecdysone production by y-organs. MIH has dual regulation in crustacean physiology. Functional studies have shown that this peptide exerts a stimulatory effect on reproduction by stimulating vitellogenesis in the hepatopancreas.11 Ecdysteroid hormones profoundly affect growth, differentiation, and metabolism. Regulation of ecdysteroid synthesis, principally achieved by molt inhibiting hormone, is a predominant factor of a complex network that governs the normal growth and proper function of decapod crustaceans.12,13 The three residues asparagine (13), serine (71) and isoleucine (72) of MIH interact with the receptor in the y-organs14,15 and activate the signaling cascade leading to a significant increase cGMP activity and inhibition of the phantom gene expression.16–18 From these observations it is hypothesized that a growth enhancer derived from the residues of MIH responsible for binding to the receptor can lead to a precocious molting and thus stimulates molting.
Consequently, elucidation of 20-hydroxyecdysone production in y-organs and the development of inhibitors to reverse or modulate the effect of MIH are important issues. Toward these objectives, pharmacophore-based ligand design has been employed to develop specific inhibitors, which has attained a gradually noticeable position within the computer assisted drug discovery process during the past few years.19,20 Our results from the study describe the first specific, highly potent growth promoter which also provides information on regulatory mechanisms that form the basis of the MIH specificity towards its transmembrane receptor on y-organs.
2. Materials and methods
2.1. Design and synthesis of N,N′-ethane-1,2-diylbis(3-bromobenzamide)
Pharmacophore feature generation and in silico screening. The pharmacophore features, virtual screening and manual alterations were performed as described by earlier.21 The HipHop Catalyst approach from Accelrys’ Discovery Studio was used to generate common feature pharmacophores of the binding site of the molt inhibiting hormone from five decapod crustaceans as described previously.22 This approach utilizes a protocol which includes generation of a three dimensional conformation model for each binding site, assigning them as active features and then uses the common feature pharmacophores for drug screening. The pharmacophore features for two residues S71 and I72 were generated using the solution structure of molt inhibiting hormone from Marsupenaeus japonicus [PDB ID: 1J0T] and the 3-D structural libraries were virtually screened. The 3-D pharmacophore geometry serves as a query in screening comprises of four features viz. hydrophobic groups, hydrogen-bond acceptor, hydrogen-bond donor, and ionizable groups. The screened hits from the compiled spatial arrangement of pharmacophores for two amino acids from MIH was then manually modified and then ranked according to their fit value. The best competitive inhibitor with highest fit value was then selected for further studies.
Synthesis of crustacean growth enhancer, CGE-1. The conventional dicyclohexylcarbodiimide (DCC) and 4-dimethylaminopyridine (DMAP) method which is used to synthesize amides was used to synthesize CGE-1 (Scheme 1). The dicyclohexylcarbodiimide (DCC) was used as a coupling agent for the ester synthesis. The structure of CGE-1 was confirmed using 1H and 13C-NMR.
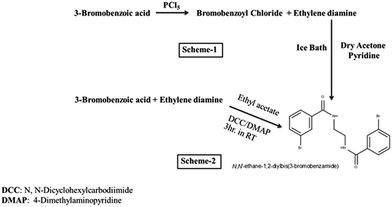 |
| Scheme 1 Route map for the synthesis of growth enhancer (CGE-1). | |
2.2. Analysis of efficacy of CGE-1
Animals. Male and female Panulirus homarus were purchased from local fisherman at Kanyakumari, Tamil Nadu, India. The lobsters were fed with food pellets once in a day during the night. The water temperature was held at 28 ± 2 °C and salinity was maintained at 28‰ under a photoperiod of 12 h light: 12 h dark. Water quality was retained by circulating the complete volume of water through a filter. Panulirus homarus ranging in weight from 55 to 101 g were selected for the study and the length [total length, carapace length and width] of each individual lobster was carefully measured using calipers.To study the effects of CGE-1 on Panulirus homarus, ninety spiny lobsters were divided into nine groups of ten. Molt staging was performed as described previously23 and after acclimatization, the first group which served as controls were injected with lobster saline [460 mM NaCl, 13 mM KCl, 13 mM CaCl2, 10 mM MgCl2, 1.7 mM glucose, 10 mM HEPES (pH 7.4)] and two of the groups were positive controls i.e., bilateral eyestalk ablated and 20-hydroxyecdysone injected animals. Three groups were injected with CGE-1 and the remaining three groups were given CGE-1 orally in increasing increments as low, medium and high doses. The three injected groups were injected with CGE-1 into the intermolt lobsters through the sinus of the fifth walking leg. The high dose group animals were injected with 254.5 μg g−1 of CGE-1 while the medium and low dose group animals were injected with a concentration of 150 μg g−1 and 53.6 μg g−1 of CGE-1 respectively. All the animals were observed for physiological changes in hemolymph for three successive moltings and subsequently the animals in all groups were sacrificed and various parameters were analyzed.
Assessment of growth. The carapace length, total length and body weight of every individual organism from the different experimental groups were measured after every successive molting.24 The measurements of growth of molted lobsters were taken two days after ecdysis when the exoskeleton was sufficiently rigid to make sure accurate measurements could be taken.
Biochemical analysis of hemolymph. The hemolymph was collected from the third walking leg and assays were performed at 0, 2, 4, 8, 12 and 24 h post-treatment with CGE-1-injection and then subsequently on day 3, 5, 7, 14, 21, 28 or until the third consecutive molting completed.Hemolymph glucose level plays an important role as an indicator of fractional or complete failure of the physiological response and therefore glucose was quantified using the GOD/POD method to monitor stress in all experimental groups.25 Total protein quantification from hemolymph was used as an index for lobster health and vitality on the use of CGE-1.26 Total protein was quantified using a biuret assay during molt and between the experimental groups. Similarly, the high density lipoprotein (HDL) was quantified using a direct enzymatic method.27
Ecdysteroid assay. The enzyme immunoassay (EIA) was performed as per the manufacturer’s instruction (Cayman Chemical, Ann Arbor, MI) using specific antibodies raised against 20-hydroxyecdysone at different time intervals throughout the molt cycle.28 After three successive moltings the y-organs from all experimental lobsters were dissected in ice cold phosphate buffered saline and the ecdysteroid titer was quantified.
Biochemical analysis of muscle and hepatopancreas. Total carbohydrate,29 total protein26 and HDL27 from both the organs were quantified after three successive molts to monitor the variation due to the CGE-1 treatment.
Analysis of hepatosomatic index. The hepatosomatic index was calculated as the function of HDL absorption and vitellogenin production.30 The hepatosomatic index was calculated by measuring weight of hepatopancreas relative to the total weight of the P. homarus.
Molt mineralization index. The molt mineralization index is defined as the ratio of gastrolith to the total weight of the lobster. The deposition of calcium was observed by using X-ray radiograph analysis (60 kV, exposure time 0.05 s) during premolt and post molt.31
Measurement of cyclic nucleotide (cGMP). After three successive moltings, the y-organs from all the experimental lobsters were excised during intermolt. cGMP quantification was performed with aliquots of the supernatant as per the protocol provided by the manufacturer (Cayman Chemical, Ann Arbor, MI). The data were represented as pmol mg−1 protein.
Analysis of cuticle. After third successive molting, scanning electron microscopy and elemental analysis of cuticle was performed to perceive plausible alterations in the network architecture during the intermolt stage. Elemental analysis was performed to confirm the presence of calcium and phosphate in the exoskeleton. Similarly, Fourier transform infrared spectroscopy (FT-IR) spectra of the cuticle were recorded after the final molt. Calcium carbonate and chitin are the two main components of lobster exoskeleton. Hence, the peaks for both were analyzed using FTIR.31
Histological evaluation of hepatopancreas, muscle, ovary and gills. All the organs were dissected and fixed for eight hours in Bouin’s fluid. The organs were then fixed in paraffin and 5 μm sections were used for histological analysis and the sections were stained with hematoxylin and eosin (HE). The stained sections were washed and then analyzed using light microscopy.32,33
Acute oral toxicity and cytotoxicity analysis. The acute oral toxicity was performed as per the guidelines of the Organization for Economic Co-operation and Development (OECD). Acute oral toxicity was performed using 8–12 weeks old female Wistar rats to ensure the sensitivity of the test.The cytotoxicity was performed using HepG-2 cell lines. Particulars of measuring cell growth inhibition using HepG-2 cells are described elsewhere. Briefly, cells were grown in Dulbecco’s Modified Eagle Medium (DMEM) supplemented with 10% fetal bovine serum (FBS) and 105 cells per well were transferred to a 96-well microtiter plate and incubated for 24 h at 37 °C with 5% CO2. The medium was removed at the end of the incubation and the cells were treated with different concentrations of CGE-1 for 24 h using the settings stated above. The MTT solution was added and incubated for 20 min at room temperature. The percent cell viability was calculated by measuring optical density at 560 nm.
3. Results and discussion
3.1. Design and synthesis of CGE-1
Pharmacophore based approaches were applied with the aim of designing compounds having similar steric and conformational features to molt inhibiting hormone that would instead optimally bind to the receptor in the y-organs. Ten distinct pharmacophore models were created and significant interactions were considered by hydrophobic, hydrogen-bond donor or acceptor features. The best HypoGen model consisted of six pharmacophore features: one hydrogen bond acceptor, three hydrogen bond donors and two hydrophobic rings. The best pharmacophore model (Hypo1) was employed for virtual screening (3D database searching), including Lipinsiki’s filter, to obtain a pool of more drug like molecules.21 The compound screening was principally focused on providing the variety of comprehensive molecular data desired to facilitate the interaction of these compounds with y-organs. This includes spatial arrangements of atoms as compared to the selected amino acids from MIH, structure and pharmacological data about designed growth modulators. The designed molecules were ranked according to their fit values and N,N′-di-(3-bromobenzoyl)diamineethane (CGE-1) which exhibited the highest fit value of 5.6 was selected for further analysis. CGE-1 is unique, not only because of its structure but also in the level of fit value and depth of coverage of intrinsic features it achieves which is important to competitively inhibit the MIH–receptor interaction in the y-organs of decapod crustaceans. In addition to its extensive coverage of important features such as the presence of hydrophobic groups, hydrogen-bond acceptors and donors, CGE-1 demonstrated excellent pharmacokinetic properties required for its usage as a growth promoter.
After analyzing the various properties of CGE-1, it was synthesized to assess its efficacy and toxicity in vivo. As mentioned in the materials and methods section, two route maps were established to synthesize CGE-1 but contrast to the acid chloride method for the synthesis of amides as depicted in the Scheme-1, Scheme-2 uses a mild environment and hence, avoids the use of toxic solvents for instance pyridine and strong reagents such as thionyl chloride. The compound was purified by column chromatography over silica gel followed by crystallization to give colorless crystals in good yield (65%). As shown in Fig. S1 and S2,† the structure and composition of CGE-1 are confirmed by 1H-NMR, 13C-NMR and it was further used for various biochemical and toxicological analysis.
3.2. Effect of CGE-1 on weight gain, molt frequency and increment
As predicted from the in silico drug design, the efficacy of CGE-1 was directly related to the molting frequency of lobsters. CGE-1 administration triggered substantial changes in molting frequency in a dose-dependent fashion. The most remarkable change was a gradual increase in the molt frequency in injected groups, which initiated around day 5 and the pattern was continued during the course of the experiment. The medium and high dose experimental groups molted three times in 16 days as compared to the control where the three moltings were concluded in 43 days (Fig. 1). In correlation with the molting frequency, a significant increase in total length, carapace length and carapace width were observed in the experimental group. A 3.3 mm increment in carapace length was observed as compared to 2.5 mm in control lobsters. 90% weight gain was observed in the medium and high dose experimental group lobsters and it is incomparable with the control, eyestalk ablated and ecdysteroid injected lobsters where the weight gain ranged between 40–50% (Fig. S3†).
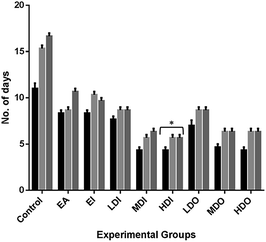 |
| Fig. 1 CGE-1 treatment shortens molt cycle duration in P. homarus. Molt durations were measured in three consecutive molt cycles and results are expressed as mean number of days ± SEM. Asterisk ( ) depicts significant decrease in intermolt stage. The duration of molting is described as first molting ( ), second molting ( ), third molting ( ) for all experimental groups. The groups are represented by: EA (eyestalk ablated), EI (ecdysteroid injected), LDI (low dose injected), MDI (medium dose injected), HDI (high dose injected), LDO (low dose oral), MDO (medium dose oral), HDO (high dose oral). | |
It is rather interesting to note that CGE-1-induced size increment and weight gain was observed even for the low and medium dose experimental groups which had only a little or no effect on the other parameters. Furthermore, the pattern shows that the increase was gradual during the progression of the CGE-1 administration at higher doses of CGE-1. Therefore, in the present study, all CGE-1 supplemented diets or injected doses resulted in a higher growth in Panulirus homarus than the control diets, signifying that the addition of CGE-1 improves the overall growth performance and diminishes the effects of stress factors which cause higher mortality in eye-stalk ablated animals during molting. Similar high mortality was observed by several researchers,34–36 who studied the effect of unilateral and bilateral eyestalk ablation in decapod crustaceans. It is also evident from our study that bilateral eyestalk ablation and ecdysteroid injection has a minor effect on these parameters as compared to the CGE-1 dose groups. In fact, our study revealed that the CGE-1-induced weight gain and molt increment was accompanied with a shortening of intermolt, as observed in all the treated groups.
3.3. Ecdysteroid quantification
Ecdysteroid was quantified initially for molt staging and an ecdysteroid level of 377 ng ml−1 was observed. During the experiment the ecdysteroid titer changes significantly in all the experimental groups at different molting stages. It was observed that the level was significantly higher in medium and high dose experimental groups (479.71 ng ml−1) as compared to saline controls during the premolt stage (Fig. S4†). Individual animals treated with CGE-1 showed a large, transient upsurge of 20E which increased to an extent with the commencement of premolt exhibiting a large premolt peak at this stage and gradually decreased in subsequent morphological stages. The level of 20E remained significantly higher until ecdysis before reaching the basal levels without causing any significant stress to the animals. There was no significant sex biased level of 20E observed in the hemolymph of experimental animals and this was highly correlated with the various molting stages. Also, the 20E quantitative values observed in the hemolymph with dose-supplemented diets were nearly equivalent to the injected group suggesting that CGE-1 exhibits enhanced absorption and distribution kinetics which permits a prompt interaction with the paired y-organs. As expected, there was no significant quantity of ecdysteroid in y-organs dissected from intermolt lobster after the third molt. These results also established that the binding of CGE-1 to the y-organ cells is reversible and it does not cause a continuous uncontrolled growth in the organism.
3.4. Variation in hemolymph glucose and protein
The hemolymph glucose level did not show a significant variation throughout the study of all experimental groups. The results from this observation indicated that the injection causes a transitory hyperglycemia to the lobsters. The variation in the hemolymph glucose occurred only during the first few hours after the injection of CGE-1 and successively reached the basal level for the rest of the time throughout the study. The glucose level was significantly higher after two hours of treatment and subsided after two hours of treatment (Fig. S5†). The changes were similar after every injection and this may be basically due to the time required for lobsters to acclimatize to the CGE-1. It is evident from previous studies that the changes in the glucose level can be caused due to hypoxia, period of immersion and handling of animals. It became relevant to consider that during the experiment the glucose levels and their period represents the acute stress in absolute terms and in all the cases there was no sustained hyperglycemia. Hence, considering these facts the stresses measured in Panulirus homarus as a function of hemolymph glucose level can be attributed predominantly to the handling, use of injection and blood sampling and hence, the CGE-1 in the hemolymph does not induce any stress in the animals. Interestingly, the level of glucose remains low throughout the study on bilaterally eyestalk ablated animals due to the absence of crustacean hyperglycemic hormone (CHH) in the physiological system (Fig. 2). During the experiments, a higher protein concentration is observed during the premolt stage due to the presence of cuticular proteins secreted by hypodermal cells in all the experimental groups. These proteins also assist in the synthesis and hardening of the new exoskeleton during postmolt.
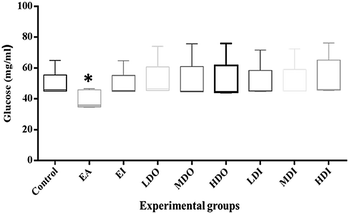 |
| Fig. 2 Mean changes in the hemolymph glucose titer during the experiment. The glucose was quantified at different time intervals as a function of stress caused during experiments. The asterisk represents the significantly lower hemolymph glucose level during the course of the experiment. Mean ± SEM (P < 0.001). The groups are represented by: EA (eyestalk ablated), EI (ecdysteroid injected), LDI (low dose injected), MDI (medium dose injected), HDI (high dose injected), LDO (low dose oral), MDO (medium dose oral), HDO (high dose oral). | |
3.5. Biochemical analysis of muscle and hepatopancreas
In muscle, the total protein concentration was higher in the medium (21.787 mg/100 g) and high dose group (21.878 g/100 g) of lobsters as compared to control lobsters (19.669 g/100 g) (Fig. S6†). A similar pattern was observed in ecdysteroid injected and eyestalk ablated lobsters where total protein concentration was higher than the control but slightly lower than CGE-1 administered lobsters. There was no significant difference in the total carbohydrate observed in all experimental groups. The total carbohydrate quantity was recorded as 1.5, 1.6 and 1.6 g/100 g in control, bilateral eyestalk ablated and high dose experimental group lobsters respectively. The improved growth performance in CGE-1 treated animals can probably be attributed to the interference of MIH binding to the y-organs resulting in higher synthesis and secretion of 20E. This 20E upsurge in the hemolymph may in turn explain the better growth and feed efficiency observed in the experimental animals. The variation in the biochemical parameters could be due to the different route of CGE-1 administration. It is also very interesting to observe that the increment in the molting-frequency due to CGE-1 treatment does not cause any adverse effect on the rates of protein synthesis.
For the hepatopancreas there were no significant changes observed in total protein (Fig. S7†) and also for carbohydrate where the medium (1.571 g/100 g) and high dose (1.60 g/100 g) groups are comparable with the control (1.521 g/100 g). But, a higher level of HDL was observed in treated lobsters as compared to controls probably due to the increased food intake and the result was correlated with the significantly increased weight gain (Fig. S8†).
3.6. Analysis of hepatosomatic and molt mineralization index
The different stages of molt cycle are key factors influencing the crustacean condition,37 the hepatosomatic index of each lobster was analyzed when all the lobsters reached the same molting stage. A significantly higher hepatosomatic index was observed in medium and high dose experimental group lobsters (3.7) as compared to control (3.1), eyestalk ablated (3.3) and ecdysteroid injected (3.34) lobsters (Fig. 3). A higher index suggests that the administration or diet supplemented with CGE-1 improve the physiology by stimulating metabolism of biomolecules and energy storage in the hepatopancreas.38 Furthermore, a significantly higher hepatosomatic index specifies the higher ability of treated animals to up-regulate the production of enzyme which enables absorption of lipids and other biomolecules and efficacious utilization of their storage reserves during the intermolt stage. Moreover, the hepatosomatic index reflects the size of the hepatopancreas as compared to their body weight. It is very significant because in crustaceans the hepatopancreas plays a vital role not only in vitellogenin synthesis, but it also helps in detoxification of the body. Hence, these results showed that CGE-1 does not cause toxicity to the hepatopancreas.
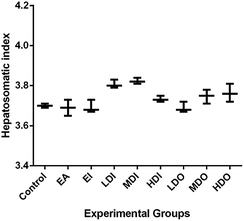 |
| Fig. 3 Molt related variations of the hepatosomatic index over three consecutive molting cycles for specimens of P. homarus. The error bars represent the standard error of the mean for n = 10 samples. No significant differences in the HSI for experimental groups were observed in relation to the treatment (P < 0.0001). The groups are represented by: EA (eyestalk ablated), EI (ecdysteroid injected), LDI (low dose injected), MDI (medium dose injected), HDI (high dose injected), LDO (low dose oral), MDO (medium dose oral), HDO (high dose oral). | |
3.7. Effect of CGE-1 on cuticle and molt-mineralization index
The predominant mineral in the lobster cuticle is amorphous calcium carbonate embedded in the chitin matrix. It was observed that the molt mineralization index was higher in CGE-1 administered lobsters as compared to saline controls (Fig. 4). An increase in the molt mineralization index correlated with the size increment of the lobster as this will help to form a new cuticle during post molt.
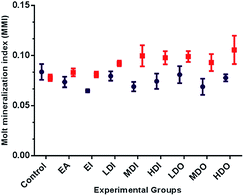 |
| Fig. 4 Progression of gastrolith growth (expressed as MMI values) in three consecutive molt cycles. The values were synchronized and are presented as mean ± SEM. Circles and squares represent initial and final molt mineralization index, respectively (p < 0.0001). The groups are represented by: EA (eyestalk ablated), EI (ecdysteroid injected), LDI (low dose injected), MDI (medium dose injected), HDI (high dose injected), LDO (low dose oral), MDO (medium dose oral), HDO (high dose oral). | |
Also, scanning electron micrographs of the cuticle revealed that no significant changes in the deposition of calcium carbonate and chitin matrix were observed in all the experimental groups (Fig. 5). The elemental analysis of the exoskeleton confirmed the presence of calcium and phosphorus in all the experimental groups. The probable changes in the FTIR spectra of the cuticle after the completion of three molt cycles in all experimental groups were recorded. The spectrum of amorphous calcium carbonate (868.12, 1072.58, 1420–1474 cm−1) and chitin absorption at 1650 cm−1 was similar in all the experimental groups (Fig. 6). In vivo experiments have shown that CGE-1 does not interfere with the shell hardening process.
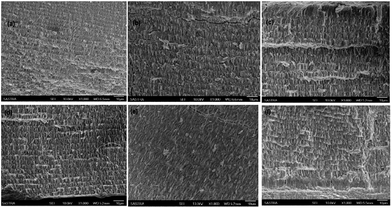 |
| Fig. 5 Scanning Electron Microscope (SEM) images of the cross-sectional fracture surface of P. homarus chitin matrix after three successive moltings. No significant difference was observed between control (a), eye-stalk ablated (b), ecdysteroid injected (c) and CGE-1 administered low (d), medium (e) and high dose groups (f). Similar outcomes were seen among CGE-1 oral and injected groups. | |
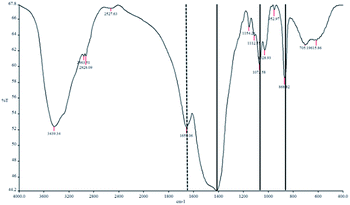 |
| Fig. 6 Fourier transform infrared spectroscopy (FT-IR) spectra of the cuticle during the intermolt in P. homarus. Solid vertical lines represent the main peaks in the vibrational spectrum of amorphous calcium carbonate (868.12, 1072.58, 1420–1474 cm−1). The dashed vertical line represents the main chitin peak (1655.06 cm−1). Similar patterns of the spectrum for calcium carbonate and chitin was detected in all experimental groups. | |
3.8. Effect of CGE-1 hepatopancreas, muscle, ovary and gills
The photomicrographs of all experimental lobsters were evaluated for cellular integrity and lesion. No significant changes were observed in the organs from treated group lobsters as compared to the saline control. The results are in accordance with our various biochemical analyses where no abnormal variations were observed. The level of various proteins, lipids and glucose were shown to be morphological stage dependent changes and these changes were relevant to the controls. Similarly, the biochemical analysis of these organs reflected a better growth and development and these factors were highly correlated with the histopathological changes in the various dose groups with different molting stages. The results also demonstrated that there are no concerns over the effect of CGE-1 on gills due to their repeated contact through water or due to the CGE-1 supplemented diet. As we have already discussed about the role of MIH and 20E on the vitellogenesis and hence, reproduction, the cellular integration has clearly indicated that the CGE-1 did not exhibit an inhibitory effect on the various stages of ovary development.
3.9. CGE-1 does not cause acute oral toxicity and cytotoxicity
No sign of toxicity was observed during gross necropsy after 14th day for all drug groups. The food intake of drug animals was comparably similar to those of control animals. At a dose of 2000 mg kg−1 of body weight no death was recorded during 14 days. These data indicate that the N,N′-di-(3-bromobenzoyl)diamineethane (CGE-1) has no toxicity and can be used to accelerate growth in crustaceans. The effect of CGE-1 on the cytotoxicity to HepG-2 cells was evaluated. The results indicate that the CGE-1 will not cause any toxicity to the humans as the hepatic cells are able to metabolize the compound and ensure excretion.
4. Conclusion
The criteria used to assess the effect of CGE-1 involves primarily mortality, growth and reproduction. Other factors such as exoskeleton abnormalities, stress sensitivity and a comprehensive biochemical analysis of various organs were also evaluated. Using these factors, the best growth enhancer, CGE-1 was validated with the intention of developing an evidence-based tool for a harmless and more effective approach to stimulate growth in decapod crustaceans. Although there are various practices adopted, including the bilateral eyestalk ablation or the use of antibiotics that influence molting, none of them has been identified for their ability to enhance molting without causing any harm to the animal or the environment. Current evidence from structural analysis of MIH, although only confirmed on spiny lobsters, suggests that CGE-1 can not only be used on lobsters, but also may have similar effects on different decapod crustacean species and facilitate rapid growth. Among those treated with CGE-1, the high dose group (both oral and injected) exhibited a considerably better molting frequency in a specified period of time than other experimental groups. The high dose group results in a 93% shortening of intermolt period as compared to the saline control after three consecutive moltings. Assessment of contemporary methods by comparison with the designed growth enhancer clearly indicated that there was a significant difference in hemolymph ecdysteroid titer and intermolt period. Since, the eyestalk secretes several neurohormones, most of these methods have produced various biological effects other than regulating the ecdysteroid production. It is also very evident from the sequence analysis and homology modeling MIH from various decapod crustaceans that they share a high degree of similarity in the binding site, hence it is highly likely that the CGE-1 can also have a similar effect on other decapod crustaceans. In that case, CGE-1 can be used as a universal growth enhancer to promote growth in several other economically important decapod crustaceans.
Acknowledgements
We are grateful to the Department of Science and Technology, Ministry of Science and Technology, Government of India for providing fellowship to Mr Sajal Shrivastava under the INSPIRE fellowship program. We also thank the management of SASTRA University for providing infrastructure for research.
References
- M. K. Chattopadhyay, Use of antibiotics as feed additives: a burning question, Front. Microbiol., 2014, 5, 1–3 CrossRef PubMed.
- S. Q. A. Shah, F. C. Cabello, T. M. L’Abée-Lund, A. Tomova, H. P. Godfrey, A. H. Buschmann and H. Sørum, Environ. Microbiol., 2014, 16, 1310–1320 CrossRef CAS PubMed.
- F. C. Cabello, H. P. Godfrey, A. Tomova, L. Ivanova, H. Dölz, A. Millanao and A. H. Buschmann, Environ. Microbiol., 2013, 15, 1917–1942 CrossRef PubMed.
- D. Soyez, Ann. N. Y. Acad. Sci., 1997, 814, 319–323 CrossRef CAS PubMed.
- F. Van Herp, in SEMINAR SERIES-SOCIETY FOR EXPERIMENTAL BIOLOGY, Cambridge University Press, 1998, vol. 65, pp. 53–70 Search PubMed.
- R. Keller, Experientia, 1992, 48, 439–448 CrossRef CAS.
- D. P. V De Kleijn and F. Van Herp, Comp. Biochem. Physiol., Part B: Biochem. Mol. Biol., 1995, 112, 573–579 CrossRef.
- F. Lachaise, A. Le Roux, M. Hubert and R. Lafont, J. Crustacean Biol., 1993, 13, 198–234 CrossRef.
- S. G. Webster, Proc. R. Soc. London, Ser. B, 1993, 251, 53–59 CrossRef CAS.
- M. A. Hesni, N. Shabanipour, A. Atabati and A. Bitaraf, Influence of eyestalk ablation and temperature on molting and mortality of Narrow-clawed Crayfish (Astacus leptodactylus), Turk. J. Fish Aquat. Sci., 2008, 8(2), 219–223 Search PubMed.
- N. Zmora, J. Trant, Y. Zohar and J. S. Chung, Saline Syst., 2009, 5, 7 CrossRef PubMed.
- G. Wainwright, S. G. Webster, M. C. Wilkinson, J. S. Chung and H. H. Rees, J. Biol. Chem., 1996, 271, 12749–12754 CrossRef CAS PubMed.
- T. Nakatsuji and H. Sonobe, Gen. Comp. Endocrinol., 2004, 135, 358–364 CrossRef CAS PubMed.
- H. Katayama, K. Nagata, T. Ohira, F. Yumoto, M. Tanokura and H. Nagasawa, J. Biol. Chem., 2003, 278, 9620–9623 CrossRef CAS PubMed.
- H. Katayama, T. Ohira, S. Nagata and H. Nagasawa, Biochemistry, 2004, 43, 9629–9635 CrossRef CAS PubMed.
- H.-W. Kim, L. A. Batista, J. L. Hoppes, K. J. Lee and D. L. Mykles, J. Exp. Biol., 2004, 207, 2845–2857 CrossRef CAS PubMed.
- E. Spaziani, T. C. Jegla, W. L. Wang, J. A. Booth, S. M. Connolly, C. C. Conrad, M. J. Dewall, C. M. Sarno, D. K. Stone and R. Montgomery, Am. Zool., 2001, 41, 418–429 CrossRef CAS.
- E. Spaziani, M. P. Mattson, W. L. Wang and H. E. McDougall, Am. Zool., 1999, 39, 496–512 CAS.
- D. Barnum, J. Greene, A. Smellie and P. Sprague, J. Chem. Inf. Comput. Sci., 1996, 36, 563–571 CrossRef CAS.
- O. Guner, O. Clement and Y. Kurogi, Curr. Med. Chem., 2004, 11, 2991–3005 CrossRef.
- S. Shrivastava and A. Princy, Rev. Aquaculture, 2013, 5, 111–120 CrossRef PubMed.
- Z. J. Witczak, T. Poplawski, A. Czubatka, J. Sarnik, P. Tokarz, A. L. VanWert and R. Bielski, Bioorg. Med. Chem. Lett., 2014, 24, 1752–1757 CrossRef CAS PubMed.
- M. J. O’Halloran and R. K. O’Dor, J. Crustacean Biol., 1988, 8, 164–176 CrossRef.
- G. S. Rao, R. M. George, M. K. Anil, K. N. Saleela, S. Jasmine, H. J. Kingsly and G. Rao, Indian J. Fish., 2010, 57, 23–29 Search PubMed.
- H. U. Bergmeyer, Principles of enzymatic analysis., Verlag Chemie., 1978 Search PubMed.
- N. Rifai, P. S. Bachorik and J. J. Albers, Lipids, lipoprotein and apolipoprotein, Tietz textbook of clinical chemistry, ed. C. A. Burtis and E. R. Ashwood, W.B. Saunders company, Philadelphia, 3rd edn, 1999, pp. 806–861 Search PubMed.
- D. A. Wiebe, Lab. Meas. Lipids, Lipoproteins Apolipoproteins, 1994, 91–105 CAS.
- P. Porcheron, M. Moriniere, J. Grassi and P. Pradelles, Insect Biochem., 1989, 19, 117–122 CrossRef CAS.
- Y. Queneau, A. P. Rauter and T. Lindhorst, Carbohydrate Chemistry: Chemical and Biological Approaches, Royal Society of Chemistry, 2014, vol. 40 Search PubMed.
- H. Do Huu and C. M. Jones, Aquaculture, 2014, 432, 258–264 CrossRef CAS PubMed.
- A. Shechter, A. Berman, A. Singer, A. Freiman, M. Grinstein, J. Erez, E. D. Aflalo and A. Sagi, Biol. Bull., 2008, 214, 122–134 CrossRef CAS.
- C. M. Santos, G. V Lima, A. A. Nascimento, A. Sales and L. M. Y. Oshiro, Braz. J. Biol., 2009, 69, 161–169 CrossRef CAS PubMed.
- J. D. Shields, K. N. Wheeler and J. A. Moss, J. Shellfish Res., 2012, 31, 439–447 CrossRef.
- G. Vázquez-Islas, I. S. Racotta, A. Robles-Romo and R. Campos-Ramos, Aquaculture, 2013, 414, 1–8 CrossRef PubMed.
- E. V. Radhakrishnan and M. Vijayakumaran, Indian J. Fish., 1984, 31, 130–147 Search PubMed.
- J. K. Leung-Trujillo and A. L. Lawrence, J. World Maric. Soc., 1985, 16, 258–266 CrossRef PubMed.
- R. K. K. Fotedar, B. Knott and L. H. Evans, Freshwater Crayfish Pap. Int. Symp., 5th, 1999, 12, 478–493 Search PubMed.
- J. Jussila, Physiological responses of Astacid and Parastacid crayfishes (Crustacea: Decapoda) to conditions of intensive culture, University of Kuopio Perth, Western Australia, 1997 Search PubMed.
Footnote |
† Electronic supplementary information (ESI) available. See DOI: 10.1039/c5ra10002j |
|
This journal is © The Royal Society of Chemistry 2015 |
Click here to see how this site uses Cookies. View our privacy policy here.