DOI:
10.1039/C5RA12009H
(Paper)
RSC Adv., 2015,
5, 85436-85441
Tannin as a gatekeeper of pH-responsive mesoporous silica nanoparticles for drug delivery
Received
22nd June 2015
, Accepted 28th September 2015
First published on 28th September 2015
Abstract
Tannin grafted on mesoporous silica nanoparticles (tannin-MSNs) was synthesized by the amidation reaction of carboxyl benzyl borate with amino group modified MSN. Tannin-MSNs were an MCM-41 type of mesoporous material with a uniform diameter of about 150 nm. The XRD and N2 adsorption/desorption isotherm results demonstrated that the rhodamine was effectively loaded and the pores of MSN were blocked by tannin. Rhodamine as a model cargo was used to evaluate the loading and releasing behaviors of tannin-MSNs. The drug release rate of tannin-MSNs was pH dependent. An in vitro cellular cytotoxicity test indicated that tannin-MSNs were highly biocompatible and suitable to be used as drug carriers. The cellular uptake was investigated by confocal laser scan microscopy. The results showed that tannin-MSNs are promising platforms to construct a pH-responsive controlled drug delivery system for cancer and stomach therapy.
Introduction
A controlled drug delivery system (CDDS) has drawn tremendous attention due to it possessing advantageous features of improved efficacy, hypotoxicity and low side effects, and reduced frequency of doses.1–4 Many materials, e.g., liposomes,5 block copolymers,6 dendrimers7 and inorganic nanomaterials8,9 have been used as drug carriers in CDDS. Among them, the mesoporous silica nanoparticle (MSN) is regarded as one of the most promising drug carriers because of its outstanding properties, such as large surface area, good chemical inertness, high thermal stability and excellent biocompatibility.10 Meanwhile, the MSN is proposed as a good candidate for load drug that can protect the encapsulated drugs from degradation and deterioration based on its highly ordered mesoporous structures with adjustable pore size.
Recently, extensive works relational with stimuli responsive MSN-CDDSs have been developed. Gold nanoparticles,11–13 inorganic iron oxide nanoparticles,14–21 dendrimers,22 and cyclodextrin 7-amino-coumarin derivative23 were used as the gatekeepers to cap the channels of MSN to avoid the loaded drug from release. The various gatekeeper release mechanisms of MSN-CDDSs such as the disulfide reduction mechanism,14,17 photochemistry mechanism,24,25 and pH trigger mechanism18,24 have been reported. In this paper, tannin was used as a gatekeeper of MSN for pH trigger platform. Tannin can be severed as an excellent gatekeeper because it is cheap and own to good biocompatibility, big stereo-hindrance and easy functionalization to form boronate esters24,26,27 with many catechol functional groups as a derivative of dopamine.
Tannin was grafted via the formation of the corresponding boroester bonds which anchored on the external surface of the mesoporous silica-based solid (Scheme 1). The tannin-MSNs were MCM-41 type of mesoporous materials with a unanimous diameter of about 150 nm. The rhodamine was chosen as a model cargo to evaluate the drug loading and releasing behaviors. Furthermore, doxorubicin hydrochloride (DOX) was loaded to study the in vitro cellular cytotoxicity test. Finally, the cellular uptake was investigated by confocal laser scan microscopy (CSLM).
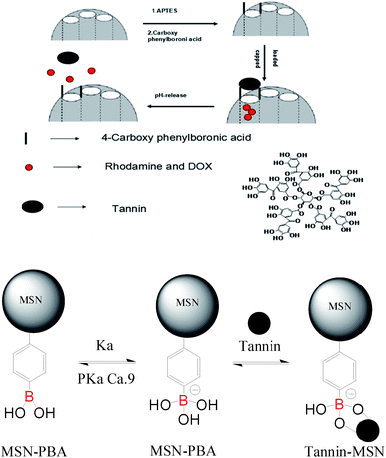 |
| Scheme 1 Preparation of drug loaded tannin-MSNs and chemical reaction mechanism of capping tannin into MSN. | |
Results and discussion
Material studio software was employed to calculate the structure of tannin. As shown in Fig. 1, the size of tannin is about 3 nm, which is suitable for a gatekeeper of MSN.
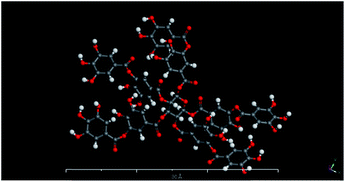 |
| Fig. 1 The structure of tannin simulated by material studio software. | |
13C CP-MAS solid-state NMR spectroscopy provides solid evidence for the formation of the tannin-MSNs. As Fig. 2 shown: a comparison of the solid state NMR spectra of tannin, MSN-PBA and tannin-MSNs was carried out. The signals at 10–42, are assigned to aliphatic carbon atoms of the APTES, and signals at 110–130 ppm are attributed to the aromatic carbon atoms of phenylboronic acid, the signal at 170 ppm is characteristic of the carbon atom from a carboxy group (C
O). The grafting of the tannin on MSN was confirmed by a substantial broadening of carbon resonances 110–150 ppm which was the result of the carbon atom signals from tannin.
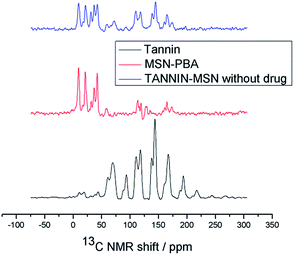 |
| Fig. 2 13C CP-MAS solid-state NMR spectra of tannin, MSN-PBA and tannin-MSNs. | |
As Fig. 3b shown that tannin encapsulated MSN displayed a thin shell, which was absent on pure MCM-41 nanoparticles (Fig. 2a). The diameters of the pure MCM-41 nanoparticles and tannin-coated MSN were about 130 nm and 150 nm, respectively. Element B was traced by EDX. In Table 1, the weight percentage and atomic percentage of B on tannin encapsulated MSN were 5.76% and 8.52%, while was 0% on pure MCM-41 nanoparticles. Both TEM and EDX results indicated that MSN was encapsulated by tannin.
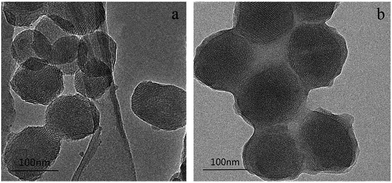 |
| Fig. 3 (a) TEM image of MSN (b) TEM image of Rh.B@tannin-MSNs. | |
Table 1 EDX data of MSN and Rh.B@tannin-MSNs
MSN |
Rh.B@tannin-MSNs |
Element |
Weight percentage |
Atomic percentage |
Element |
Weight percentage |
Atomic percentage |
|
|
|
B K |
5.76 |
8.52 |
C K |
23.53 |
34.64 |
C K |
37.34 |
49.59 |
O K |
36.19 |
40.00 |
O K |
36.44 |
36.35 |
Si K |
40.27 |
25.35 |
Si K |
20.46 |
11.62 |
Total |
100.00 |
|
Total |
100.00 |
|
As Fig. 4 shown that the intensity of the powder X-ray diffraction peaks (d100, d110 and d200 peaks) were decreased with the filling of the Rh.B and the gatekeeper of tannin.
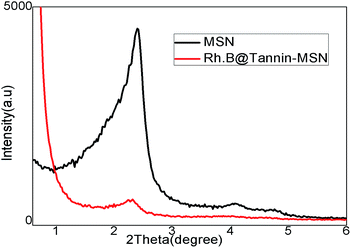 |
| Fig. 4 X-ray diffraction (XRD) patterns of MSN Rh.B@tannin-MSNs. | |
In Fig. 5, the MSN isotherms of nitrogen adsorption/desorption are type IV with H1-type hysteresis loops, while the Rh.B@tannin-MSNs isotherms are type IV with H3-type hysteresis loops. BET surface area and pore size of MSN and Rh.B@tannin-MSNs were shown in Table 2. The decrease of BET surface from 833 m2 g−1 to 302 m2 g−1 proved that MSN was capped by tannin and Rh.B loaded on MSN.
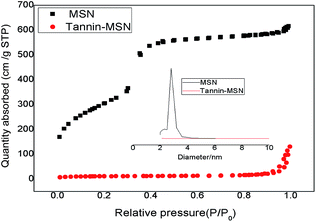 |
| Fig. 5 Nitrogen adsorption–desorption isotherms and pore size distribution of MSNs and Rh.B@tannin-MSNs. | |
Table 2 Summary of the porous properties of the tannin-MSN
Sample |
SBET/(m2 g−1) |
Pore size/nm |
Vt/(cm3 g−1) |
MSN |
833.56 |
3.86 |
0.82 |
Rh.B@tannin-MSNs |
302.72 |
|
0.49 |
The TGA curves of tannin-MSNs nanoparticles were shown in Fig. 6. The carboxyl benzyl borate modified MSN nanoparticles losed about 6.2% weight from ca. 25 to 280 °C, which was attributed to the desorption of physically adsorbed water, weight loss losed about 2.0% occurred from 280 to 400 °C, which was due to the further condensation of the silane coupling agent. The decline in the temperature range from ca. 400 to 800 °C should be ascribed to the removal of carboxyl benzyl borate grafted onto the surface when heated in the nitrogen atmosphere. And tannin capped MSN had similar curve with carboxyl benzyl borate modified MSN and more weight loss due to the introduction of rhodamine and tannin, while MSN presented lower weight loss. All of above results indicated that tannin was grafted on MSN.
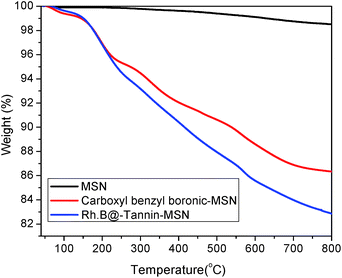 |
| Fig. 6 TGA curves of MSN, PBA-MSN and Rh.B@tannin-MSNs. | |
Release behaviors were measured at different pH to understand the pH-trigger gating behavior of Rh.B@tannin-MSNs nanoparticles. The released dye amount was shown in Fig. 7 at the different pH. It was noticed that little amount of dye was released out into the solution under shaken for 120 h in PBS solution (pH 7.4), signifying the efficient confinement of dye in the pores of MSN by virtue of capped with tannin. As the pH decreased, tannin was cleaved more quickly and the release of model drugs was effectively accelerated. While the Rh.B@MSNs shown a higher release rate than Rh.B@tannin-MSNs. The above results suggested that the tannin as gatekeepers could retain the fluorescein molecules in the pores and still prevent undesired leaching from the mesopores in the pH 7.4 solution. But the boronate esters can be cleaved under lower pH.
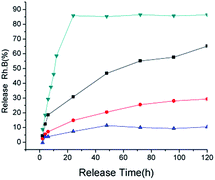 |
| Fig. 7 Release profiles of Rh.B@tannin-MSNs and Rh.B@MSNs at different pH: (black) Rh.B@tannin-MSNs at pH 1.7; (red) Rh.B@tannin-MSNs at pH 5.0; (blue) Rh.B@tannin-MSNs at PBS pH 7.4; (blue-grey) Rh.B@MSNs at PBS pH 7.4. | |
In vitro cell assay, the in vitro cell cytotoxicity of MSN and tannin-MSNs to Marc-145 cells were investigated by MTT. In Fig. 8a both MSN and tannin-MSNs showen no obvious cytotoxic effect on the Marc-145 cells at 0.5 μg ml−1 after incubation for 24 h. Even as the concentration of tannin-MSNs was as high as 50 μg ml−1, the cell viability was about 88% after incubation for 24 h. These results demonstrated that both MSN and tannin-MSNs are nontoxic at low concentrations and slightly toxic at high concentrations. Therefore, tannin-MSNs are highly biocompatible and suitable to be used as the drug delivery in CDDS.
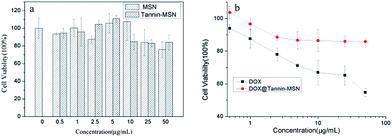 |
| Fig. 8 (a) Cell viabilities of Marc-145 cells incubated with different concentrations of MSN and tannin capped MSN without drug for 24 h (b) cell viabilities of Hela cells incubated with different concentrations of DOX and DOX@tannin-MSNs for 24 h (data are presented as the average ± standard deviation (n = 3)). | |
As the Fig. 8b shown that the in vitro cellular cytotoxicity of DOX and DOX@tannin-MSNs to Hela cells at different concentrations. The cytotoxicity of DOX and DOX@tannin-MSNs increased with the increase of their concentrations, and DOX@tannin-MSNs has lower cytotoxicity than DOX. After 24 h incubations with free DOX and DOX@tannin-MSNs, the cell viabilities were 95% and 105% at the concentration of 0.5 μg ml−1, 55% and 90% at the concentration of 50 μg ml−1, respectively.
The cellular uptake of DOX@tannin-MSNs by Hela cells were further investigated by CLSM. Hela cells were cultured at 37 °C for 24 h. DOX@tannin-MSNs were put into the culture medium with a DOX concentration of 10 μg ml−1. Then the nucleus was stained with DAPI and the pretreated cells were observed under CLSM. As shown in Fig. 9, both DOX@tannin-MSNs and control group presented DAPI fluorescence. The DOX fluorescence was mainly observed in the cytoplasm of the cells when the cells were cultured in the DOX@tannin-MSNs for 24 h. While the blank group presented no DOX fluorescence. CLSM results shown that DOX@tannin-MSNs may be taken up by the cells through an endocytosis mechanism and the DOX was released in endocytic compartments.
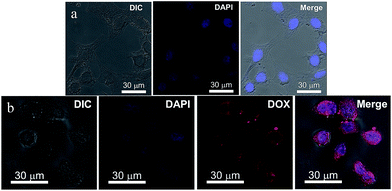 |
| Fig. 9 CLSM images of Hela cells (a) control group (b) Hela cell were cultured with DOX@tannin-MSNs for 24 h. Cell nuclei were stained with DAPI. | |
Experimental
Materials
All reagents were available from commercial sources. N-Cetyltrimethylammonium bromide (CTABr), 4-carboxy phenylboronic acid, DOX, rhodamine, (3-amincpropyl)triethoxysilane, N-hydroxysuccinimide (NHS), tannin, tris(hydroxymethyl)aminomethane, tetraethoxysilane (TEOS) and 1-ethyl-3-(3-dimethylaminopropyl)carbodiimide hydrochloride (EDC-HCl) were purchased from Aldrich and used directly without further purification. Anhydrous ethanol, dichloromethane, hydrochloric acid, sodium dihydrogen phosphate and disodium hydrogen phosphate sodium citrate were purchased from Sinopharm Chemical Reagent Co. RPMI-1640, fetal bovine serum, penicillin and streptomycin were purchased from Gibco.
Methods
Powder XRD patterns were recorded on a X-ray diffractometer (D/MAX-2200/PC, Rigaku) equipped with Cu Kα radiation (40 kV, 20 mA) at a rate of 1.0° min−1 over the range of 6(2θ). TEM-EDX images were taken with a microscope (JEM-2010, JEOL) operating at 200 kV. The solid-state13 CNMR spectra were performed on a Bruker Avance 400 NMR spectrometer at 400 MHz with 4 mm sample rotors in a tripleresonance probe head. The nitrogen adsorption/desorption isotherms were measured at 196 °C with a Micromeritics ASAP 2010M + C porosimeter. The surface area was calculated by the Brunauer–Emmett–Teller (BET) method, and the pore size was obtained from the maximum of the pore size distribution curve calculated by the Barrett–Joyner–Halenda (BJH) method using the adsorption branch of the isotherm. TGA were investigated by a Perkin Elmer 7 Series thermal analysis system from 40 to 800 °C at a heating rate of 20 °C min−1 under N2 flow. The UV-vis spectrum was recorded on a Perkin Elmer (Lambda 750S) UV-vis spectrometer. All measurements were performed in quartz cuvettes. PBS 7.4 was used as a blank. The confocal laser scan microscopy images were performed by LSM510 META, ZEISS.
Synthetic procedures
Synthesis of silica mesoporous nanoparticles (MCM-41). In a typical reaction, the MCM-41 mesoporous nanoparticles were prepared by the following procedure: CTABr (1.0 g, 2.74 mmol) was dissolved in 480 ml deionized water. Then 3.4 ml NaOH (2.00 M in deionized water) was added to the CTABr solution, followed by rising the solution temperature to 80 °C. TEOS (5.0 ml, 25.7 mmol) was added dropwise to the solution. The white precipitate was emerged after the mixture was stirred for 2 h. Finally, the solid product was filtered, washed with deionized water and ethanol several times, and dried at 60 °C. The final porous material (MCM-41) was calcined at 550 °C in an oxidant atmosphere for 6 h in order to remove the template phase.
Synthesis of MSN-NH2 nanoparticles (S1). 0.25 g MCM-41 solid was suspended in 20 ml of anhydrous ethanol. Then 0.5 g APTES (2.26 mmol) was added and the mixture was stirred for 12 h. The product (S1) was separated by centrifugation (10k rpm, 10 min) after washed with ethanol several times, and dried at 80 °C for 12 h.
Synthesis of carboxy phenylboronic acid grafted onto MSN-NH2 nanoparticles (S2). 0.5 g 4-carboxy phenylboronic acid (2.55 mmol) was resolved in 20 ml dichloromethane. Then 0.6 g EDS (2 mmol) and 0.23 g NHS (2 mmol) was added. The mixture was stirred at 0 °C for 30 min. After that, S1 was added into the solution and stirred at room temperature for 12 h under nitrogen atmosphere. Then, the particles were centrifuged (10k rpm, 10 min) and washed with dichloromethane several times, and dried at 80 °C for 12 h.
Synthesis of Rh.B/DOX@tannin-MSN (S3). 0.20 g S2 was soaked and stirred in 20 ml (pH 9.0) with Rh.B/DOX (4 mg) at 37 °C for 24 h, followed by the addition of 0.2 g tannin (0.12 mmol). The solution was stirred at 37 °C for 6 h, and then centrifuged 10
000 rpm for 10 minutes and resuspended in DI water to remove excess tannin and Rh.B/DOX. To calculate the Rh.B/DOX loading efficiency, the supernatant fluid was collected, and the residual Rh.B/DOX content was determined using the calibration curve of Rh.B standard solutions by the UV-vis measurement at 495 nm. The loading efficiency of Rh.B/DOX is about 77%/79% respectively in MSN, which was calculated as follows:
In vitro drug release of tannin-MSNs in PBS with different pH. To measure in vitro drug release, 50 mg S3 was put into a dialysis bag and sealed, and then put into the brown bottle with 100 ml PBS with different pH. The brown bottle was placed in a constant temperature shock chamber at 37 °C and shook at the speed of 100 rpm. At certain time intervals, 3 ml of the release medium was fetched to test the released drug concentration and then returned to the brown bottle. The released Rh.B concentration was measured by recording the absorbance of the release medium at 559 nm on a UV-vis absorption spectrophotometer (Lambda 35, Perkin-Elmer).
In vitro cell assay. Hela cells were cultured at 4 × 105 cells per dish in RPMI-1640 supplemented with penicillin (100 U ml−1), streptomycin (100 mg ml−1), and 10% heat-inactivated fetal bovine serum. Before particles were added, Hela cells were allowed to adhere to the Petri dish at 37 °C, 5% CO2 atmosphere incubated for 24 h. Each Petri dish was added 100 μl tannin-MSNs nanoparticles with architecture (0.2 mg, loading of DOX 36 mg g−1). Nanoparticles were incubated with cells for 24 h. After incubation, cells were washed with PBS three times and examined under the confocal laser scan microscopy (LSM-510, Zeiss).
Methylthiazol tetrazolium (MTT) cell viability assay. Firstly, Hela/Marc-145 cells were cultured in a 96-well plate at the concentration of 5 × 105 cells per ml in 100 μl culture medium of RPMI-1640 supplemented with 10% fetal bovine serum, 100 U ml−1 penicillin, and 100 mg ml−1 streptomycin, and incubated at 37 °C, 5% CO2 atmosphere for 24 h. Total volume was brought up to 200 μl by adding 100 μl of culture medium. DOX@tannin-MSNs/tannin-MSNs were added with different concentrations in triplicate wells for each sample. The cells were cultured in 37 °C, 5% CO2 atmosphere for 24 h. After the incubation, cells were washed and replenished with fresh culture medium, which were further incubated for 2 h. Then, the cells were incubated with MTT, which was at a final concentration of 0.5 mg ml−1 for each well, cultured at 37 °C, 5% CO2 atmosphere for 4 h. Finally, to dissolve the resulting formazan crystals, 100 μl of DMF solubilization solution was added to each well. Absorbance was measured at a wavelength of 570 nm.
Conclusions
Tannin-MSNs was successfully prepared by the amidation of carboxyl benzyl borate acid and amino group functionalized MSN. The releasing behaviors of tannin-MSNs is controllable by adjusting pH of solution. The in vitro cellular cytotoxicity test demonstrated that the tannin-MSNs was highly biocompatible, nontoxic and suitable to utilize as drug carriers in CDDS. Furthermore, CLSM results shown that DOX@tannin-MSNs may be taken up by the cells through an endocytosis mechanism and the DOX are released in endocytic compartments. It is possible that tannin-MSNs are promising platforms to construct pH-responsive CDDS for cancer therapy and stomach therapy.
Acknowledgements
The authors are grateful for project support from The Dow Chemical Company, the National Science Fund of China (21274085) and the Shanghai Leading Academic Discipline Project (No. B202).
References
- Y. Zhu, Y. Fang and S. Kaskel, J. Phys. Chem. C, 2010, 114, 16382–16388 CAS.
- X. Guo and F. C. Szoka, Acc. Chem. Res., 2003, 36, 335–341 CrossRef CAS PubMed.
- S. Kapoor and A. J. Bhattacharyya, J. Phys. Chem. C, 2009, 113, 7155–7163 CAS.
- D. Peer, J. M. Karp, S. Hong, O. C. Farokhzad, R. Margalit and R. Langer, Nat. Nanotechnol., 2007, 2, 751–760 CrossRef CAS PubMed.
- L. Hosta-Rigau, R. Chandrawati, E. Saveriades, P. D. Odermatt, A. Postma, F. Ercole, K. Breheney, K. L. Wark, B. Stadler and F. Caruso, Biomacromolecules, 2010, 11, 3548–3555 CrossRef CAS PubMed.
- X. Yang, J. Grailer, I. Rowland, A. Javadi, S. Hurley, V. Matson, D. Steeber and S. Gong, CrossRef, PubMed, CAS, Web of Science® Times Cited, 1.
- Y. Shen, Z. Zhou, M. Sui, J. Tang, P. Xu, E. A. V. Kirk, W. J. Murdoch, M. Fan and M. Radosz, Nanomedicine, 2010, 5, 1205–1217 CrossRef CAS PubMed.
- T. Panczyk, T. P. Warzocha and P. J. Camp, J. Phys. Chem. C, 2010, 114, 21299–21308 CAS.
- M. C. Urbina, S. Zinoveva, T. Miller, C. M. Sabliov, W. T. Monroe and C. S. Kumar, J. Phys. Chem. C, 2008, 112, 11102–11108 CAS.
- Y.-S. Lin and C. L. Haynes, J. Am. Chem. Soc., 2010, 132, 4834–4842 CrossRef CAS PubMed.
- F. Torney, B. G. Trewyn, V. S.-Y. Lin and K. Wang, Nat. Nanotechnol., 2007, 2, 295–300 CrossRef CAS PubMed.
- R. Liu, P. Liao, Z. Zhang, R. J. Hooley and P. Feng, Chem. Mater., 2010, 22, 5797–5799 CrossRef CAS.
- C.-L. Zhu, C.-H. Lu, X.-Y. Song, H.-H. Yang and X.-R. Wang, J. Am. Chem. Soc., 2011, 133, 1278–1281 CrossRef CAS PubMed.
- S. Giri, B. G. Trewyn, M. P. Stellmaker and V. S. Y. Lin, Angew. Chem., Int. Ed., 2005, 44, 5038–5044 CrossRef CAS PubMed.
- W. Xu, Y. Liao and D. L. Akins, J. Phys. Chem. B, 2002, 106, 11127–11131 CrossRef CAS.
- H. Zheng, Y. Wang and S. Che, J. Phys. Chem. C, 2011, 115, 16803–16813 CAS.
- C.-Y. Lai, B. G. Trewyn, D. M. Jeftinija, K. Jeftinija, S. Xu, S. Jeftinija and V. S.-Y. Lin, J. Am. Chem. Soc., 2003, 125, 4451–4459 CrossRef CAS PubMed.
- F. Muhammad, M. Guo, W. Qi, F. Sun, A. Wang, Y. Guo and G. Zhu, J. Am. Chem. Soc., 2011, 133, 8778–8781 CrossRef CAS PubMed.
- M. Mahmoudi, S. Sant, B. Wang, S. Laurent and T. Sen, Adv. Drug Delivery Rev., 2011, 63, 24–46 CrossRef CAS PubMed.
- T. Sen, S. J. Sheppard, T. Mercer, M. Eizadi-Sharifabad, M. Mahmoudi and A. Elhissi, RSC Adv., 2012, 2, 5221–5228 RSC.
- M. E. Sharifabad, T. Mercer and T. Sen, J. Appl. Phys., 2015, 117, 17D139 CrossRef PubMed.
- D. R. Radu, C.-Y. Lai, K. Jeftinija, E. W. Rowe, S. Jeftinija and V. S.-Y. Lin, J. Am. Chem. Soc., 2004, 126, 13216–13217 CrossRef CAS PubMed.
- H. Meng, M. Xue, T. Xia, Y.-L. Zhao, F. Tamanoi, J. F. Stoddart, J. I. Zink and A. E. Nel, J. Am. Chem. Soc., 2010, 132, 12690–12697 CrossRef CAS PubMed.
- E. Aznar, M. D. Marcos, R. N. Martínez-Máñez, F. Sancenón, J. Soto, P. Amorós and C. Guillem, J. Am. Chem. Soc., 2009, 131, 6833–6843 CrossRef CAS PubMed.
- N. K. Mal, M. Fujiwara and Y. Tanaka, Nature, 2003, 421, 350–353 CrossRef CAS PubMed.
- Y. Li, W. Xiao, K. Xiao, L. Berti, J. Luo, H. P. Tseng, G. Fung and K. S. Lam, Angew. Chem., 2012, 124, 2918–2923 CrossRef PubMed.
- M. DenizáYilmaz and J. FraseráStoddart, Nanoscale, 2015, 7, 7178–7183 RSC.
|
This journal is © The Royal Society of Chemistry 2015 |
Click here to see how this site uses Cookies. View our privacy policy here.