DOI:
10.1039/C5RA13067K
(Review Article)
RSC Adv., 2015,
5, 85395-85409
Synthesis and use of bimetals and bimetal oxides in contaminants removal from water: a review
Received
5th July 2015
, Accepted 25th September 2015
First published on 25th September 2015
Abstract
Water pollution aggravates water scarcity by contaminating large volumes of available water. There has been increasing interest in the use of bimetallic particles and bimetallic oxides for the removal of contaminants in water. This paper reviews the recent advances in the development of bimetals and bimetal oxides, and application in the treatment of environmental contaminants. 183 published studies (1999–2015) are reviewed in this paper. The synthesis methods of bimetals including chemical methods, physical methods, and biosynthesis methods and the synthesis of bimetal oxides including hydrothermal, impregnation, sol–gel, spray pyrolysis, and precipitation methods are summarized. Then the application of bimetals and bimetal oxides to remove different environmental contaminants in water including chlorinated organic compounds, heavy metal, arsenic and selenium, nitro compounds and azo dyes, anions and oxyanions are reviewed. The review focuses on experimental conditions, removal efficiency of contaminants, and reaction mechanism in the application of bimetals and bimetal oxides. Compared with monometals, bimetals have high catalytic or removal ability for contaminants. The synthesis and application of bimetals and bimetal oxides is remarked on.
1 Introduction
Clean and plentiful water provides the foundation for prosperous communities. We rely on clean water to survive, however, right now we are heading towards a water crisis. The world's thirst for water is becoming one of the most pressing resource issues of the 21st Century. Water pollution adds enormously to the existing problems of water scarcity by contaminating large volumes of available water, which stems from high population growth and growth of cities.
The use of zero-valent metals, such as zinc,1–3 aluminum,4–6 and especially iron in contaminant removal has been intensively evaluated in the last five years.7–10 Although much research has been conducted for contaminates removal by zero-valent iron (ZVI), ZVI processes a greater number of challenges, including the need for high doses,11 and its long term reactivity being limited due to surface passivation over time.
The limitation of mono metal and metal oxide includes: slow removal efficiency for contaminants,12–15 easily deactivation,12,13,16,17 and the removal is easily affected by the pH.17,18 To improve the reactivity of mono metal and metal oxide, bimetallic particles were developed by coating small amounts of noble metals (Pd, Pt or Ag) or another metal (Ni, Mn or Cu), onto a freshly prepared metal surface. There has been great interest in the use of bimetallic particles for the removal of pollutants in recent years.19–22 Doping some other metal elements such as Ce, Mn, Ti, Co, and Zr into iron oxides to synthesize bimetal oxides has also become a growing concern for scholars. Bimetal oxides as adsorbents or catalysts to remove a range of contaminants have been studied.23–26 Bimetals and bimetal oxides play a significant role and have a bright future in contaminants remediation. There is an increasing interest in the use of bimetals and bimetal oxides for the removal of contaminants from water and this is reflected in the overall increasing number of journal articles published in recent ten years (Fig. 1a). Besides, from Fig. 1b, the articles published in the recent ten years occupy 82% of all publications on bimetals and bimetal oxides according to ISI Web of Knowledge data base.
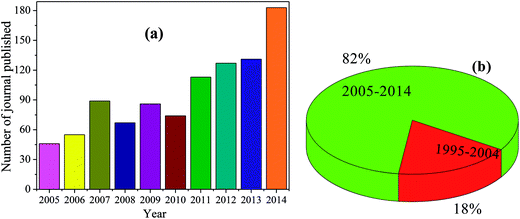 |
| Fig. 1 (a) Number of journal publications on bimetals and bimetal oxides over the past decade, and (b) the percentage of publications on bimetals and bimetal oxides in 1995–2004 and 2005–2014. | |
At least four review papers on bimetal or bimetal oxides technology have been published in the last five years. They summarized different aspects of science and technology in this field. One review is on the use of ZVI and bimetals of iron in degradation of chlorinated phenols.27 A work focused on iron oxide nanomaterials in wastewater treatment.28 O'Carroll et al. reviewed the nZVI/bimetallic nanometals for remediation of sites contamination.29 The other review was focused on the advance of bimetal Fe nanoparticles in synthesis and application in elimination of environmental pollutants.30 It can be seen that all the above published reviews were on iron based bimetals or bimetal oxides. To the best of our knowledge, there is no review on the bimetals or bimetal oxides that not only contain Fe, but also does not contain Fe such as Ni/Zn, Cu/Al and their bimetal oxides.
In this paper, the synthesis methods and the use of bimetals and bimetal oxides in the removal of environmental contaminants from water are reviewed. This review focuses on the recent development of synthesis methods of bimetals and bimetal oxides, experimental conditions, removal efficiency of contaminants, and reaction mechanism of bimetals and bimetal oxides with chlorinated organic compounds, heavy metals, arsenic and selenium, nitro compounds and azo dyes, and anions and oxyanions.
2 Synthesis of bimetals and bimetal oxides
2.1 Synthesis of bimetallic particles
Liu et al. summarized the synthesis of bimetallic Fe nanoparticles (NPs),30 so in this paper we mainly focus on the synthesis methods of bimetals composed of other metals. The synthesis methods of bimetals can be classified into three major types: chemical methods, physical methods, and biosynthesis methods.
2.1.1 Chemical methods.
2.1.1.1 Chemical reduction. In this method, metal salts dissolved in a solution are reduced by a reductant. This method is regarded as the most common method for preparing metallic NPs.31 To synthesize bimetallic particles, two different types of metals are reduced in a solution. Through co-reduction, a kind of chemical reduction, the structure of bimetals can be controlled due to the different redox potential between two metals. The metal with higher reduction potential is reduced first to form a core while the other one is reduced later and precipitates onto the surface of the core metal, which is called core–shell structure.31,32 Nevertheless, the reduction and the nucleation process are hard to control due to the different redox potential and chemical behaviors of the two metals when stronger reductants were used.32 There are two approaches to overcome the deficiency. One is to use appropriate surfactants or polymeric ligands to passivate the particle surface and control the reducing procedures. For instance, Cao et al. prepared Au/Pt bimetallic nanochains from their chloride precursors using polyvinylpyrrolidone (PVP) as stabilizer ligand and NaBH4 as the reductant.33 The other one is to select a weaker reductant. For example, Han et al. synthesized Au/M (M = Pd, Rh, Pt) bimetallic nanocrystals using chloride of Au and M as precursors and oleylamine as reductant.34 The Au–M bimetallic particles resulted in nanoscale and crystallization. Besides, other mild reductants (such as ascorbic acid and CO) were also used to prepare bimetallic particles.35,36 Some of other bimetallic materials synthesized by chemical reduction are summarized in Table 1.
Table 1 Bimetallic particles synthesized by chemical reduction
Bimetal |
Reducing agents |
Metal precursors |
Synthesis details |
Ref. |
Pt/Pd |
Ethylene glycol |
H2PtCl6, Na2PdCl4 |
Reduction of the mixture of Pt/Pd precursors by ethylene glycol in the presence of PVP and AgNO3 at 160 °C |
37 |
Cu/Ni |
H2 |
Cu(NO3)2, Ni(NO3)2 |
Precursors were added in ammonia solution, which was stirred at room temperature for 24 h, ultrasonicated for 3 h, aged for 24 h, and then reduced by H2 at 773 K for 4 h |
38 |
Ag/Cu |
NaBH4 |
AgNO3, CuSO4 |
NaBH4 was slowly dropped to the mixture of two precursors under ice cold condition and nitrogen atmosphere |
39 |
Au/Ni |
NaBH4 and H2 |
HAuCl4, NiCl2 |
A flask containing precursors and sodium dodecyl sulfate was put under ultrasonicator and NaBH4 was added into the mixture. The resulting powers were dried at 50 °C before they were annealed under a H2–Ar atmosphere (H2 vol. 5%) at 750 °C |
40 |
Co/Pd |
Oleylamine |
PdCl2, Co(NO3)2 |
Oleylamine was added into a mixture solvent of the precursors at a controlled temperature from 120 °C to 230 °C |
41 |
Pd/Ru |
H2 |
PdCl2, RuCl3 |
A mixture solvent of resulting mesoporous silica NPs, ethanol and precursors were reduced by H2 at 200 °C for 2 h |
42 |
Au/Pd |
NaBH4 |
HAuCl4, PdCl2 |
The fresh NaBH4 solution was added into the mixture solvent of precursors and poly vinylalcohol, then the solution was stirred for 30 min to form the NPs |
43 |
Pd/Ni |
H2 |
PdCl2, Ni(NO3)2 |
Precursors and γ-Al2O3 were mixed in acetic acid solution to form catalyst forerunners. After desiccation, the forerunners powers were reduced by hydrogen (0.15 L min−1) in nitrogen at 500 °C for 2 h |
44 |
2.1.1.2 Seeded growth. The method of seeded growth is a commendable approach to prepare core–shell and heterostructure bimetallic compounds.45 If the surface of seed metal is deposited by the secondary metal, the core–shell structures will form; when the secondary metal deposits on the specific site of the seed, the heterostructure will form. It is significant to adjust the thermodynamic and kinetic parameters during the synthesis of specified morphology of bimetallic particles by seeded growth method.31 Chen et al. synthesized Cu-based bimetallic nanorods by heterogeneous seeded growth.45 There are some reports that the structures of bimetallic materials are well-ordered by the seeded growth approach. For example, Adekoya et al. successfully synthesized PVP and dodecanethiol seed mediated Ag/Ru allied bimetallic NPs by seed growth or successive addition method using Ru as seed.46 The synthesized bimetallic NPs possessed well-ordered core–shell structure. From the recent research of Ko et al.,47 Au/Ag core–shell NPs with controllable shell thicknesses were generated by seeded growth approach, which can be used to detect adenosine. Han et al. reported that bimetallic Cu–Pt alloy NPs with polyhedral, stellated, or dendritic morphologies were attained by a seeded growth approach.48 Besides, Yu and He synthesized dendritic Au/Ag bimetallic NPs by the seed-assisted approach,49 in which Ag was as NPs seed.
2.1.1.3 Diffusion method. Diffusion is an achievable approach to synthesize alloy noble metal nanocrystals with spatially uniform structure (e.g. core–shell structure). Compared with wet chemical reduction methods, diffusion can achieve the aim more easily and mildly.50 From the research of Zhang et al.,50 trisoctahedral Au/Pd alloy nanocrystals were formed by diffusion of H2PdCl4 and HAuCl4 precursors. They found when the reaction temperature increased to a certain value, the crystal growth rate of an individual component depended only on the rate of diffusion of the precursors to the nuclei surfaces. However, Yu et al. reported that stable Au–AuSn, Au–Sn, AuSn–AuSn2 and AuSn2 nanocrystals were successively formed in the diffusion process at room temperature,51 which were in intermetallic core–shell structures. With increasing the temperature to 70 °C, the isolated metallic Sn was generated and cannot diffuse into the precursors of Au nanocrystals.
2.1.2 Physical methods.
2.1.2.1 Mechanical alloying method. Mechanical alloying method is a balling milling process in a dry, high energy environment, in which particles are kneaded with each other constantly and re-fractured because of the ball-particle collisions. The particle has the same content as the blended initial particle. The whole procedure of mechanical alloying includes repeated cold welding and fracturing. Bimetallic particles prepared by means of mechanical alloying are widely used for hydrogen generation, dechlorination and hydrogenation.52–54 Fan et al. prepared a series of Al-based materials via ball milling, which is a widely used mechanical alloying method.52 Their results showed Bi and Sn can enhance Al reactivity in Al bimetal than Zn, Ca, and Ga. In addition, Al–Bi alloy together with the compounds can accelerate the formation of its mico-galvanic cell between the anode of Al and cathode of Bi.52 Coutts et al. reported that mechanically alloyed Mg/Pd was prepared by a SPEX 8000M high-energy ball mill with 4 μm magnesium and palladium impregnated on graphite.53 Zaranski and Czujko found that the hydrogen desorption kinetics of MgH2–FeTiHx composites,54 which were synthesized by reactive mechanical milling, were affected not only by the phase composition, the milling parameters, and the amount of additive, but also by the milling time.
2.1.2.2 Sonochemical synthesis. Sonochemical synthesis is through the reduction of metal ions by ultrasound waves, which can initiate water homolysis to generate species ˙H. However, the sonochemical methodology had less been studied compared with other methodologies. Kan et al. reported a composite nano-structure of a core of Au and a shell of Ag with PVP which were prepared under ultrasound using Ag(NH3)2(OH) and HAuCl4 as precursors.55 Anandan et al. reported bimetallic Au–Ag with a core–shell structure was generated sonochemically at 20 kHz in room temperature and Ar atmosphere.56 Besides, Martínez-Casillas and Solorza-Feria synthesized Pd/Cu bimetal with regular spherical agglomerates using ultrasound, which had an average size of 8 nm.57
2.1.2.3 Radiolysis method. Radiolysis is an efficient method to synthesize bimetals.58 By this method, aqueous solution with metal precursors and stabilizer ligand is irradiated by γ-rays to generate solvated electrons. Metal ions can accept electrons to form metal atoms. Then the metal atoms tend to be aggregated.32,59 The rate and final nano-structure that the bimetallic particles formed are dependent on the γ-ray dosage. Higher dosages are more likely to form the homogeneous alloys over with core–shell morphologies.32 This methodology has been widely used to prepare bimetallic particles. For example, Doudna et al. reported that homogeneous crystalline Ag/Pd bimetallic clusters were successfully prepared with Ag2SO4 and PdSO4 under γ-radiation which was up to 2 kGy.59 Moreover, they also reported that bimetallic Ag/Pt NPs were generated with Ag/Pt ions and poly(vinyl alcohol) which was irradiated with gamma rays at dose rates below 0.5 kGy h−1.60 Remita et al. synthesized Au/Pt bimetals with HAuCl4 and K2PtCl4 by irradiation with 5 kGy.61 Mirdamadi-Esfahani et al. used KAuCl4 and H2PtCl6 to synthesize Au/Pt bimetallic NPs under high/low dose rate of γ-irradiation.62
2.1.3 Biosynthesis method. Biosynthesis method to synthesize bimetallic NPs at room temperature is paid more and more concerns. This method uses non-toxic eco-friendly reductants (e.g. plant extracts, plant powders) to reduce metal ions, which is different from chemical reduction.63 The functional groups in the reductants play a critical role in reducing and capping the bimetallic particles. This method is frequently applied to synthesize bimetallic NPs. Sheny et al. used the aqueous extract and dried powder of Anacardium occidentale leaf to prepare Au–Ag bimetallic NPs by reducing the precursors of HAuCl4 and AgNO3.64 They found lower amounts of plant material were sufficient to bring about reduction. Kumari et al. synthesized Au/Ag bimetallic NPs with reducing HAuCl4 and AgNO3 by fruit juice of pomegranate.65 Phenolic hydroxyls and proteins in the pomegranate fruit extract played an important role in reducing and stabilizing the bimetallic NPs. As shown in Fig. 2, Au/Ag particles were resulted in nanoscale and fcc crystal structure. Mondal et al. reported that the Au/Ag bimetallic NPs were synthesized using aqueous extract of dried leaves of mahogany.66 Various polyhydroxy limonoids in mahogany leaf extract contributed to reduce and stabilize the bimetallic NPs. The competitive reduction of Au and Ag ions synchronously led to the synthesis of Au–Ag bimetallic NPs, which was similarly reported in the research by Tamuly et al.67 Apart from Au/Ag bimetallic NPs, Ti/Ni68 and Au/Pd63 bimetallic NPs have also been successfully synthesized by bio-reduction method.
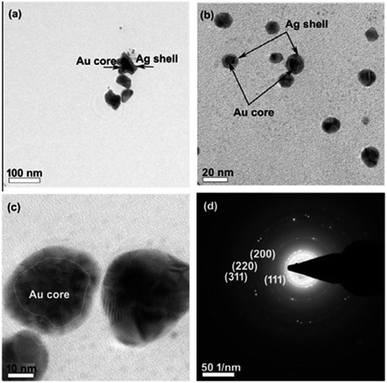 |
| Fig. 2 (a) and (b) TEM images at different magnifications, (c) HRTEM, and (d) SAED pattern of AuAg bimetallic particles with precursor ions respectively in the molar ratio and 1 : 1 (adapted with permission from literature 65. Copyright (2015) Elsevier). | |
2.2 Synthesis of bimetallic oxides
Bimetallic oxides can be synthesized using different methods. The characterization and performance of the bimetallic oxides are both strongly affected by the depositional approaches, the choice and ratio of chemical precursors, components of solvent and temperature of calcination.69–72 Some representative methods to synthesize bimetallic oxides are hydrothermal method, impregnation method, sol–gel method, spray pyrolysis method, and precipitation method.
2.2.1 Hydrothermal method. Hydrothermal method can prepare bimetallic oxides with porous structures and particular morphologies from metal salts. A facility such as Teflon-lined autoclave is needed to create a condition with spontaneous pressures and special temperature for crystallization of metal precursors.73 Various bimetallic oxides can be synthesized by controlling the reaction pH, time, temperature, and the composition of reactants in this method. From the research by Somacescu et al.,74 porous ZnO/Eu2O3 bimetallic oxides were synthesized by hydrothermal process using ZnSO4 and Eu(NO3)3 as precursors and cetyltrimethylammonium bromide as template. Straight strip morphology and hierarchical structure of the oxides were obtained as shown in Fig. 3. Without templates, Su et al. reported Ce/Zr binary oxide NPs with diverse structures, sizes and surface properties through accurate control of the ratio of Ce and Zr were prepared by hydrothermal method.75 Najafi et al. synthesized the Cu/Mo bimetallic oxides with three-dimensional morphology by hydrothermal method and nanoscale Cu/Mo bimetallic oxides formed by a sonochemical process.76 They found ultrasonication was unable to influence the crystal structure of the bimetallic oxides. Other bimetallic oxides (e.g. Fe/Mg,69 Ni/Mo,77 Co/Mo,77 Ni/W,77 Co/W,77 Fe/Mn,78 Ti/Zr,79 and Ni/Mn80) have also been synthesized by hydrothermal method.
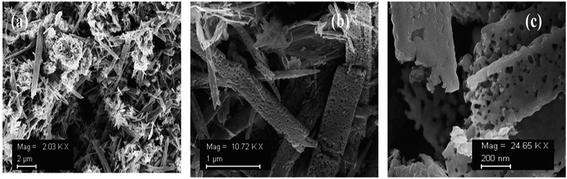 |
| Fig. 3 SEM images (a–c) for Zn/Eu2O3 (adapted with permission from literature 74. Copyright (2012) Elsevier). | |
2.2.2 Impregnation method. This technology is a synthetic process to prepare materials supported bimetallic oxides. In general, one kind of metal oxide or other solid support material prepared previously is impregnated with an aqueous solution of another metal salts or other two metal salts to synthesize bimetallic oxides, and then calcination is carried out. For example, nanosized Zn/Zr binary oxides were synthesized by impregnation method in the research by Ibrahim where the zirconium hydroxide was as a support material.81 El-Shobaky et al. reported nanostructured Cu and Mn bimetallic oxides and the corresponding monometallic oxides supported on cordierite were obtained through impregnation route.82 They found the calcination temperature affected the activity of bimetallic oxides supported on cordierite, which was opposite to the single phase supported catalyst. Several bimetallic oxides synthesized by this method are shown in Table 2.
Table 2 Bimetallic oxides prepared using impregnation method
Synthetic |
Supported materials |
Calcination conditions |
Ref. |
CuO–MnO/γ-Al2O3 |
γ-Al2O3 |
450 °C for 2 h in air |
83 |
MnO–CeO2/γ-Al2O3 |
CuO–CeO2/γ-Al2O3 |
TiO2/ZrO2 |
ZrO2 |
600 °C for 6 h in air |
70 |
MnOx/CeO2 |
CeO2 |
550 °C for 5 h in air |
84 |
Fe2O3/MgO |
Mg(OH)2 |
500 °C for 4 h in air |
69 |
Co3O4/CeO2 |
CeO2 |
600 °C for 2 h in air |
85 |
2.2.3 Sol–gel method. Sol–gel method is a technology for synthesis of bimetallic oxides with microporous and mesoporous structure. The bimetallic oxide is a result of hydrolyzing and polymerizing the metal precursors with polymerizers to obtain a sol or a gel.86 Metal alkoxide, metal halide, and metal nitrate are used as the precursors.70,86,87 Different sol–gel processes, concentrations of templates, and temperatures of calcination can lead to different structures of bimetallic oxides.71,86,88 As shown in Fig. 4, TiO2–Al2O3 binary oxides with high adsorption capacity and photocatalytic activity was prepared with different surface morphologies by adjusting the concentration of polyethylene glycol and 0.03 mol L−1 polyethylene glycol was the best concentration.86 Other researches on the synthesis of bimetallic oxides by sol–gel methods are summarized in Table 3.
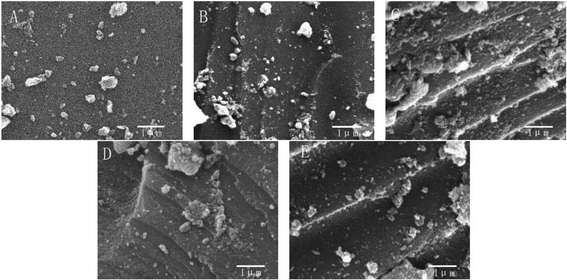 |
| Fig. 4 SEM images of mesoporous TiO2–Al2O3 binary oxides prepared using different concentration of PEG1000: (a) 0 mol L−1, (b) 0.012 mol L−1, (c) 0.030 mol L−1, (d) 0.048 mol L−1, and (e) 0.060 mol L−1 (adapted with permission from literature 86. Copyright (2012) Hindawi). | |
Table 3 Synthesis of bimetal oxides by sol–gel methodsa
Synthetic |
Precursor |
Solvent |
Gelata |
Calcination conditions |
Ref. |
NA: not available. |
Al2O3/La2O3 |
Al(O-sec-but.)3, La(acac)3 |
Ethanol |
2-Methyl-2,4-pentanediol |
650 °C for 4 h in air |
89 |
Ca2Fe2O5 |
CaCl2 from shells of African land snail, FeCl3 |
H2O |
NaOH |
1100 °C in air |
90 |
Fe/Co oxide |
Co(NO3)2, Fe(NO3)3 |
H2O |
Citric acid |
800 °C for 2 h in air |
91 |
Ca/actinide (Th and U) oxide |
Calcium nitrate, actinide nitrate |
Ethylene glycol |
Citric acid |
900 °C for 5 h in air |
92 |
CaO/Al2O3 |
AlOOH prepared from Al(OBu)3, Ca(NO3)2 |
Ethanol |
NA |
1100 °C for 3 h in air |
93 |
TiO2/ZrO2 |
Ti(OCH(CH3)2)4, Zr(OCH2CH2CH3)4 |
Ethanol and water |
NA |
550 °C for 2 h in air |
94 |
2.2.4 Spray pyrolysis method. In spray pyrolysis method, bimetal oxides thin films and nano-powders can be effectively and easily prepared in an equipment. For preparation of bimetallic thin films, bimetallic precursors were sprayed and deposited on substrates in an apparatus with regular parameters (e.g. temperature, solution flow rate, nozzle to substrate distance and carrier gas pressure), which can affect the structure and grain size of the film.95,96 For preparation of nano-powders, the viscous gel of bimetallic precursors are firstly obtained, then the gel undergoes a smouldering burning in a device such as a furnace with a relatively higher temperature where plenty of ashes can generate. The product ashes are crushed to obtain powders.97,98 However, the characteristics of the powders varied with the temperature of calcination following the ashes crushing. Zhang et al. reported a mild, ultrafast and eco-friendly spray pyrolysis strategy to prepare nano-phase ZnMn2O4.99 In their research, precursory solution of manganous and zinc acetate were sprayed into a tubular furnace with a high speed of 1 mL s−1 while precursory solution of these two acetate with absolute ethanol and ethylene glycol relatively were treated under the same conditions. Interestingly, these three kinds of resultants revealed the same phase and structure of ZnMn2O4 NPs and ethylene glycol can increase the specific surface area and pore volume of the particles.
2.2.5 Precipitation method. Precipitation including co-precipitation and asynchronous precipitation is a simple and popular wet chemical method for synthesis amorphous nano-structure bimetallic oxides.100,101 Precursors of metal salts are precipitated by adjusting the pH value to alkalescence or alkalinity with precipitants such as sodium hydroxide and ammonia. Moreover, calcination with appropriate temperature followed by the separation of the sediments is needed to generate particles with small size and high specific surface area, whereas improper temperature can lead to the decrease of specific surface area.102,103 However, the disadvantage of agglomeration can appear during the process of preparation. The addition of dispersing agents and stabilizers can be an effective method.72,104,105 Other studies on synthesis of bimetallic oxides by precipitation method are summarized in Table 4.
Table 4 Synthesis of bimetallic oxides by precipitation methoda
Bimetal oxides |
Precursor |
BET surface area (m2 g−1) |
Precipitant |
Temperature (°C) |
Calcination conditions |
Ref. |
NA: not available. |
Fe/Mn |
KMnO4, FeSO4 |
231 |
NaOH |
25 |
NA |
106 |
Fe/Zr |
FeCl2, ZrOCl2 |
106.2 |
NaOH |
50 |
500 °C in air for 4 h |
107 |
Fe/Mn |
FeSO4, MnCl2 |
Above 200 |
Oxalic acid |
0 |
300 °C in air for 1 h with heating rate of 1 °C/min |
108 |
Zr/Mn |
Zr(NO3)4, Mn(NO3)2 |
127.4–144.5 |
Ammonia |
Room temperature |
500 °C for 5 h in air |
109 |
Fe/Zr |
FeCl3, ZrOCl2 |
74.61 |
NaOH |
60 |
NA |
110 |
Zr/Mn |
Mn(VII) salt, Mn(II) salt, zirconium salt |
213 |
NA |
Room temperature |
NA |
111 |
Fe/Mn |
FeCl3, FeSO4, MnSO4 |
NA |
NaOH |
Room temperature |
NA |
112 |
Ce/Mn |
Ce(NO3)3, Mn(NO3)2 |
106 |
Na2CO3 |
Room temperature |
550 °C for 6 h in air |
73 |
Ti/Al |
TiCl4, AlCl3 |
186–218 |
Ammonia gas |
Room temperature |
500 °C for 6 h in air |
113 |
Sn/Mn |
SnCl4, Mn(NO3)2 |
47.9–54.0 |
Aqueous ammonia |
Room temperature |
500 °C for 5 h in air |
114 |
Cr/Zr |
Cr(NO3)3, ZrOCl2 |
59.9–220.8 |
Aqueous ammonium |
60 |
500 °C for 3 h in air |
115 |
Mg/Al |
Mg(NO3)2, Al(NO3)3 |
52.1–83.4 |
NaOH |
Room temperature |
At 600, 800 and 1000 °C, respectively, for 3 h in air |
116 |
3 Application of bimetals and bimetal oxides for the removal of contaminants
3.1 Removal of chlorinated organic compounds (COCs)
3.1.1 COCs removed by bimetals. COCs are ubiquitous contaminants in environment, which pose a great threat to human health, since they are recalcitrant, lipophilic, and can be bioaccumulated through food chain.117 In recent years, bimetal-catalyzed dechlorination has become one of the hotspots in the chemical degradation of chlorinated toxic organic compounds (Table 5). Just take the most typical Fe based bimetallic system for example, in bimetallic system, a second metal M (such as Pd, Ni, Pt, and Cu) deposited on metal substrate serves as a promising alternative hydrodechlorination reagent and the active hydrogen species produced from the corrosion of metal substrate is further stored in M lattice. Besides the catalytic function, the galvanic effect between the Fe anode and the stable M cathode can also improve the reactivity due to the increase of corrosion of metal substrate.
Table 5 Selective of catalytic removal of COCs by different bimetallic particlesa
COCs |
Bimetal |
Optimum pH |
Dosage and ratio of bimetal |
Removal efficiency |
Ref. |
NA: not available. |
2-Chlorobiphenyl |
Pd/Al |
3.0 |
5.0 g L−1, 1.43 wt% Pd |
100% |
118 |
Hexachlorobenzene |
Cu/Fe NPs |
≤4.0 |
NA, 5.0 wt% Cu |
98% |
119 |
2,4-Dichlorophenol |
Pd/Fe NPs |
5.7 |
6.0 g L−1, 0.20 wt% Pd |
99.4% |
120 |
Dichlorodiphenyltrichloroethane |
Ni/Fe NPs |
4–10 |
0.05 g L−1, Ni/Fe molar ratio is 1 : 3.5 |
>90% |
121 |
1-(2-Chlorophenyl)ethanol |
Pd/Fe |
5.59 |
20.0 g L−1, 0.10 wt% Pd |
100% |
122 |
2,4,6-Trichlorophenol |
Pd/Zn, Ni/Zn, Cu/Zn, Pt/Zn |
4.9 |
200.0 g L−1, Pd, Ni, Cu and Pt content is 636, 1269, 1272, and 887 ppm (mg of catalytic metal per kg of base metal) |
100% was only observed in Pd/Zn |
123 |
Hexachlorobenzene |
Pb/Fe |
7.0 |
84.2 g L−1, 1.4 wt% Pb |
99% |
124 |
3-Chlorophenol |
Pd/Al NPs |
3.0–4.0 |
2.0 g L−1, 1.16 wt% Pd |
99.7% |
125 |
Pentachlorophenol |
Ni/Fe NPs |
5.3 |
12.0 g L−1, 0.5 wt% Ni |
100% |
126 |
γ-Hexachlorocyclohexane |
Pd/Fe NPs |
6.5 |
0.5 g L−1, total iron content 59.2% |
100% |
127 |
Dichloromethane |
Cu/Al |
10.0 |
60.0 g L−1, 20 wt% Cu |
98% |
12 |
Tetrachlorobisphenol A |
Pd/Fe |
6.0 |
6.0 g L−1, 0.044 wt% Pd |
100% |
13 |
1,2,3,4-TCDD |
Ag/Fe |
6.85 |
20.0 g L−1, 0.006 mol% Ag |
>90% |
14 |
Carbon tetrachloride |
Fe/Al |
6.7 |
10.0 g L−1, Fe/Al molar ratio is about 2 : 3 |
100% |
16 |
The use of Fe based bimetals in removing COCs achieved great success, but its reactivity may be hard to remain in an extended run due to the spontaneous and uncontrollable ZVI corrosion. The corrosion causes the loss of catalyst base, iron oxide precipitation, and excessive H2 formation, which further leads to the deactivation of M/Fe.128 As to the bimetallic NPs, the reactivity is greatly improved because the decrease in particle size can lead to a higher intrinsic reactivity and less mass transfer restrictions. However, due to the very high reactivity, the deactivation of bimetallic NPs is quite easy and rapid through the formation of metal oxide encapsulation or agglomeration. It is reported that the loading NPs onto carbon microspheres or polymeric granules and adsorption of the polymer or surfactant on the surface of bimetallic NPs can hinder the agglomeration.129 Nevertheless, these modification methods of bimetallic NPs will obviously increase the cost-efficiency in their further application. Furthermore, the removal mechanism of the hydrodechlorination by bimetals varied at different pH values. The electron transfer is through the formation of the galvanic cells at higher pH, whereas at low pH mainly via direct reduction of H+.130
New attempts were made with the introduction of other active metal to replace Fe as the substrate in bimetallic systems, such as Sn,17 Zn,123 Mg,131 and Al.132 Agarwal et al. prepared Pd/Mg bimetals through a simple wet-chemistry procedure,133 in which Pd0 was reductively deposited onto Mg by intimately mixing it with a palladium nanoparticle precursor in ethanol. The synthesized Pd/Mg bimetals were used to dechlorinate 2-chlorobiphenyl, and the performance for 2-chlorobiphenyl dechlorination in the presence of naturally abundant anions was evaluated. Inspired by the study of Agarwal et al., Yang et al. selected Mg, Al, Mn, Zn, Fe, Sn, and Cu as the substrates of the Pd-loaded bimetallic particles.118 They investigated the catalytic hydrodechlorination of 2-chlorobiphenyl in aqueous solution with these Pd-loaded bimetallic particles and found that Pd/Al particles had the highest stability and relatively high reactivity in acid aqueous solution (Fig. 5). Pd/Mg and Pd/Al particles exhibited to be effective for hydrodechlorination mainly because Mg and Al possess significantly lower standard electrode potential and unique corrosion properties, resulting in a greater force to drive the hydrodechlorination reaction.
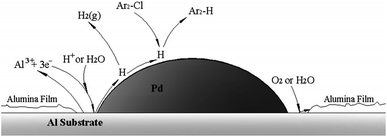 |
| Fig. 5 Proposed mechanism for the hydrodechlorination of 2-chlorobiphenyl on Pd/Al bimetallic particle in acidic solution (adapted with permission from literature 118. Copyright (2011) Elsevier). | |
3.1.2 COCs removed by bimetal oxides. Compared with mono-metallic oxides, bimetal oxides have two advantages. First, the change of physical properties like surface area, surface charge, porosity, and crystallinity might enhance the catalytic activity and adsorption capacity;15 Second, bimetal oxides combined the advantages of different oxides.134 Using bimetallic composite oxides as the catalysts for hydrodechlorination had achieved great success just as the bimetals. Zhou et al. studied the catalytic Al/Mg composite oxide on the degradation and dechlorination of PVC-containing mixed plastics.135 The comparative experiments in Al/Mg composite oxide, MgO, and γ-Al2O3 revealed that Al/Mg composite oxide had a good combination of the advantages of MgO and γ-Al2O3. Therefore, the Al/Mg composite oxide catalyst showed both cracking and dechlorination ability can be effectively used for catalytic degradation and dechlorination of PVC-containing mixed plastics. Ma et al. investigated the dechlorination of hexachlorobenzene with a mixture of commercial CaO and α-Fe2O3 (CaO/α-Fe2O3) in closed systems at temperatures of 300 °C and 350 °C.136 They found that the dechlorination efficiency was dramatically enhanced due to the remarkable synergic effect of CaO/α-Fe2O3 compared with CaO or α-Fe2O3 alone. The proposed mechanism showed that unsaturated iron ions at the surface serve as an initial adsorption sites for hexachlorobenzene leading to the breakage of C–Cl bond, and calcium servers as a sink for chlorine ion regenerating iron oxide.
3.2 Removal of heavy metals
3.2.1 Heavy metal removed by bimetals. Owing to the toxicity effects of heavy metal on human and wildlife, non-biodegradable, and bioaccumulation even at relatively low concentration, heavy metal contamination has gained great concerns.137 The toxicity of heavy metals in the environment depends on its transformation, solubility, and mobility, which are governed by redox reactions, precipitation/dissolution reactions, and adsorption/desorption phenomena.29 Consequently, these mechanisms are often involved in water treatment strategies for removing heavy-metal pollutants.138Recent advances have shown that bimetals with great redox activity and adsorption capacity can effectively remove heavy metals ions. The most typical bimetals proposed in the remediation of heavy metal contamination is bimetallic Fe or bimetallic Fe NPs. With the introduction of a second metal like Pd, Ag, Ni, Pt, and Zr with ZVI or ZVI NPs, the formed bimetallic system can provide more surface active sites and increase heavy metal removal efficiency. Their galvanic cells effect can also accelerate the heavy metal removal efficiency. The use of bimetallic Fe or bimetallic Fe NPs for reductive removal of heavy metal such as Cr(IV), Cd(II), and Cu(II) achieved great success.139–141 Moreover, stabilizing bimetallic Fe NPs in a matrix which has certain properties of adsorption of inorganic contaminants can ameliorate its capability of eliminating heavy metal contaminants. For example, Kadu et al. investigated the remediation of Cr(VI) by Fe–Ni bimetallic NPs and their nanocomposites prepared with montmorillonite (MMT) clay (Fig. 6),142 which included in situ formed and loaded Fe–Ni NPs on MMT. The incorporation of Fe/Ni NPs into MMT clay matrix guaranteed the proper dispersion of NPs and the removal capacity was enhanced mainly due to the adsorption tendency of MMT as well as reduction capacity of NPs. The recycle experiments demonstrated that only the in suit formed Fe/Ni NPs on MMT kept 100% removal in the first 3 cycles.
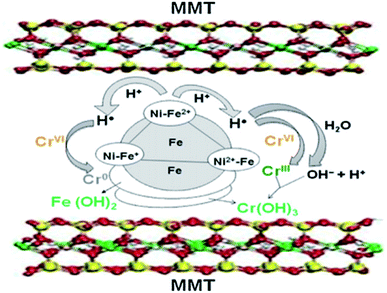 |
| Fig. 6 Schematic diagram of mechanism pathway for Cr(VI) reduction by Fe/Ni NPs (adapted with permission from literature 142. Copyright (2011) Elsevier). | |
3.2.2 Heavy metal removed by bimetal oxides. Just like bimetals, bimetal oxides also demonstrated a remarkable capacity in elimination of heavy metal contamination. The bimetal oxides were employed to remove heavy metal ions such as Sb(III),106 Cr(VI),143 and Co(II).144 Different from the bimetals, bimetal oxides normally used as adsorbents in the removal of the heavy metal ions. Therefore, the removal mechanism of heavy metals by bimetals and bimetal oxides are not the same (Table 6).
Table 6 Mechanisms of the heavy metal removed by bimetals and bimetal oxides
Heavy metal |
Bimetals or bimetal oxides |
Optimum pH |
Removal capacity (mg g−1) |
Mechanism |
Ref. |
Cr(VI) |
Ag/Fe NPs |
2.0 |
55.18 |
Reduction |
145 |
Cd(II) |
Au doped nZVI particles |
9.0 |
188 |
Reduction and adsorption |
146 |
Pb(II) |
Fe/Mg (hydr)oxides |
5.0–10.0 |
95.88 |
Adsorption |
147 |
Cu(II) |
Co/Fe2O3 NPs |
6.0 |
50.9 |
Adsorption |
148 |
Sb(V) |
Fe/Zr oxide |
≤5.5 |
51 |
Adsorption |
134 |
Hu et al. reported an ingenious strategy for the recycling of Cr(VI)-enriched sorbents,149 which are Zn/Al-based nanocomposites with a Zn to Al ratio of 4
:
1. These Zn/Al-based nanocomposites not only behave as a sorbent, but a photocatalytic function can be evolved by calcination. The Zn/Al composite sorbents showed good removal capacities for Cr(VI) even after repeated absorption cycles. Liu et al. investigated the simultaneous removal of Cd(II) and Sb(V) by Fe/Mn binary oxides.150 They found that the simultaneous adsorption of Sb(V) and Cd(II) onto Fe/Mn binary oxides can be achieved over a wide initial pH range from 2 to 9, and the Cd2+ showed higher affinity towards Fe/Mn binary oxides than Ca2+ and Mn2+.
3.3 Removal of arsenic and selenium
Arsenic (As) and selenium (Se) are not heavy metal elements, but they show similar toxicity of heavy metals, consequently they are classified as semi-metallic elements. The most typical method for the removal of As and Se is adsorption. Bimetal oxides are reported to be excellent adsorbents for the removal of As and Se.26,105,151–155
Basu et al. summarized that among the adsorbent materials investigated for arsenic removal, the performances of the mixed metal oxides were found to be superior to that of the individual oxides.156 Zhang et al. prepared a novel Fe–Mn binary oxide by low cost materials using a simultaneous oxidation and coprecipitation method.157 The Fe–Mn binary oxide can not only completely oxidize arsenite (As(III)) to arsenate As(V), but also can effectively remove both As(V) and As(III), particularly the As(III), attributing to the combination of the oxidation property of manganese dioxide and the high adsorption features to As(V) by iron oxides. In their further study, they employed X-ray absorption spectroscopy to investigate the change of the oxidation state of arsenic and manganese during As(III) sorption (using X-ray absorption near-edge structure) and to determine the mechanisms of arsenic sorption in single and binary systems of Fe and Mn oxides (using near-edge X-ray absorption fine structure).158 The results showed that the MnOx content is mainly responsible for oxidation and the FeOOH content is dominant for adsorption, and the As surface complex is an inner-sphere bidentate binuclear corner-sharing complex (Fig. 7). Zhang et al. synthesized bimetal oxide magnetic nanomaterials (MnFe2O4 and CoFe2O4) and employed them to remove As(III) and As(V).159 The maximum adsorption capacities of As(III) and As(V) on MnFe2O4 were 94 and 90 mg g−1, and on CoFe2O4 were 100 and 74 mg g−1, respectively. MnFe2O4 and CoFe2O4 showed higher As(III) and As(V) adsorption capacities than the referenced Fe3O4, which might be caused by the increase of the surface hydroxyl (M–OH) species.
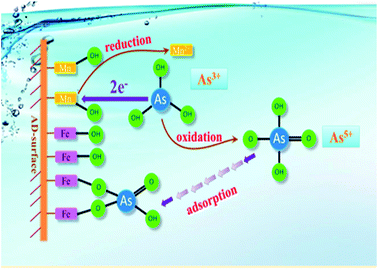 |
| Fig. 7 Schematic diagram of mechanism pathway for As(III) removal by Fe–Mn binary oxide (adapted with permission from literature 158. Copyright (2014) American Chemical Society). | |
Sun et al. investigated the removal of Se(IV) and Se(VI) by three types of MFe2O4 (M = Mn, Cu, Co) spinel ferrite NPs,160 which were prepared through a hydrothermal method. Due to their simple synthesis process, high saturation magnetization, and excellent performance, CuFe2O4 and CoFe2O4 can be promising adsorbents for Se(IV) and Se(VI) removal compared with MnFe2O4.
3.4 Removal of nitro compounds and azo dyes
3.4.1 Nitro compounds and azo dyes removed by bimetals. Nitro compounds are usually classified into nitro aliphatic and nitro aromatic compounds. Compared with nitro aliphatic compounds, nitro aromatic compounds have a broader application and harder to be degraded, leading a more widely detection in the environment. Azo compounds are another widespread nitrogenous compounds in the environment, especially azo dyes, which contain one or more azo (N
N) bonds, are the most important and largest class of synthetic dyes used in commercial applications.161 Due to the existence of N–O and N
N bonds, nitro or azo compounds are refractory pollutants in the environment.The use of bimetals or bimetallic NPs in the elimination of nitro compounds are well reported, especially in the degradation of some persistent nitro aromatic compounds (Table 7). Xiong et al. executed a comparative study on the reactivity of Fe/Cu bimetallic particles and ZVI in the degradation of p-nitrophenol under different aeration conditions.167 Results showed that dissolved oxygen can improve the mineralization of p-nitrophenol, and Cu can enhance the reactivity of ZVI. p-Nitrophenol was degraded into nontoxic and biodegradable intermediate products, and subsequently mineralized into CO2 and H2O. Kim et al. investigated the reduction of nitro compounds and olefins by Pd/Pt bimetallic NPs on functionalized multi-wall-carbon nanotubes.168 They found Pd/Pt bimetallic NPs on functionalized multi-wall-carbon nanotubes have remarkable activity for efficient chemoselective reduction of nitro compounds without any loss of activity in the 10 times recycles.
Table 7 Nitro compounds and azo dyes removal using bimetals and bimetallic NPs
Nitro compounds or azo dye |
Bimetals or bimetal NPs |
Optimum conditions |
Removal efficiency |
Ref. |
Hexahydro-1,3,5-trinitro-1,3,5-triazine |
Bi/Fe NPs |
1.0 g L−1 Bi/Fe, 4%-Bi/Fe0 (atomic ratio) at pH 3.0 |
100% |
162 |
Hexahydro-1,3,5-trinitro-1,3,5-triazine, high melt explosive, trinitrotoluene, nitrotriazolone, nitroguanidine, 2,4-dinitroanisole |
Fe/Cu |
3% solid/liquid (S/L) Fe/Cu loading 9.7 wt% Cu at pH 3.0 |
100% |
163 |
para-Nitrochlorobenzene |
Pd/Fe |
40 g L−1 Pd/Fe, 0.03 wt% Pd at pH 6.5 |
100% |
164 |
2,4-Nitrophenols |
Fe@Au NPs |
1.4 g L−1 Fe@Au, 0.06 M NaBH4 at pH 7.2 |
100% |
165 |
Acid black 1 |
Cu/Fe |
0.5 g L−1 Cu/Fe, Cu/Fe ratio (wt) 1 : 1 at pH 9.0 |
94% TOC removal |
166 |
Methylene blue |
Cu/Fe |
100.0 g L−1 Cu/Fe, Cu/Fe ratio (wt) 1 : 4 at pH 6.0–9.0 |
98% color removal |
18 |
The performances of bimetals or bimetallic NPs in the catalytic reduction of azo dyes have been investigated thoroughly in recent years, since they are considered as effective catalysts to break the N
N bonds in the azo dyes. Bokare et al. reported the reductive degradation of azo dye Orange G in aqueous solution using the Fe–Ni bimetallic NPs.169 They found that the reductive cleavage of the azo linkage leads to the formation of aniline and naphthol amine derivatives with the latter remaining adsorbed on the particle surface.
The leaching of toxic heavy metal from bimetals will lead to great threats to our life and environment. Therefore, various versatile catalyst supports were utilized to suppress metal leaching, which may bring excellent catalyst performance and practicability. Cai et al. prepared two supported bimetallic catalysts of Fe–Co/GAC and Fe–Co/SBA-15 and employed them in the ultrasound enhanced heterogeneous activation of peroxydisulfate and peroxymonosulfate process to degrade Acid Orange 7 and Orange II, respectively.170,171 The sulfate radicals (SO4−˙) were assumed to be the dominating reactive species for the Orange II decolorization in the US/Fe–Co/SBA-15/PMS system (Fig. 8). Both Fe–Co/GAC and Fe–Co/SBA-15 exhibited good activity and stability in the decolorization of Acid Orange 7 and Orange II.
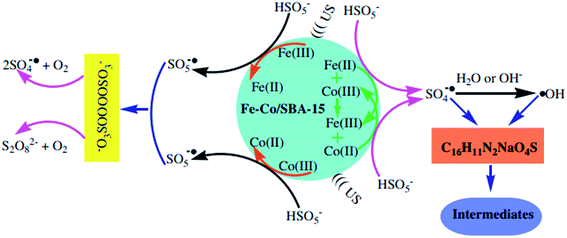 |
| Fig. 8 Proposed mechanism of US/Fe–Co/SBA-15/PMS system (adapted with permission from literature 171. Copyright (2015) Elsevier). | |
3.4.2 Nitro compounds and azo dye removed by bimetal oxides. Bimetal oxides are also well reported in the removal of nitro compounds and azo dyes. Hua et al. investigated the degradation process of two dyes through catalytic wet air oxidation with the catalyst CuO/γ-Al2O3 prepared by consecutive impregnation.172 Results indicated that the degradation of dyes began with the cleavage the azo bond, proceeding with the oxidation of the lower molecular weight intermediate, and resulting in the complete mineralization of dye to CO2 and H2O. The CuO/γ-Al2O3 catalysts exhibited a remarkable reactivity with CuO acting as O2 transfer carrier and the high surface area, low cost, and high strength γ-Al2O3 acting as carrier.Besides γ-Al2O3, some efforts have been made on the use of iron oxide NPs as support to develop the magnetically recoverable nanocatalysts for the removal of nitro compounds and azo dyes. Chiou et al. have successfully synthesized Ag/Fe oxide composite NPs via a facile one-pot green process.173 The use of Ag/Fe oxide composite NPs for 4-nitrophenol removal revealed its high activity and stability, giving this magnetically recoverable nanocatalysts a promising future in wastewater treatment. Safavi et al. investigated the degradation of the selected azo dyes using a novel palladium/hydroxyapatite/Fe3O4 nanocatalyst.174 The high catalytic activity, magnetic separatability and good stability of palladium/hydroxyapatite/Fe3O4 nanocatalyst make it a good alternative for decontamination.
3.5 Removal of anions and oxyanions
3.5.1 Anions and oxyanions removed by bimetals. The anions and oxyanions in aqueous system coming from the natural and anthropogenic processes pose a great threat to human and other living creature. For example, some anions and oxyanions of the halogen elements are of particular concern due to their toxicity, mutagenicity, carcinogenicity, and radioactivity.175 Besides halogen anions and oxyanions, nitrate, sulfate and phosphate are all widespread anion constituents in surface water, groundwater, and wastewater. Membrane techniques,176 adsorption,177 and selective reduction are all alternative strategies for the elimination of these anions and oxyanions.178It is well recognized that the ideal way to remove nitrate is via the selective reduction of NO3− to N2 without further reduction to NH4+ or NH3. Bimetals have been proposed to use in the selective reduction of nitrate and achieved success. Liou et al. investigated the selective reduction of NO3− to N2 using bimetallic particles of Pd/Zn, Pt/Zn, and Cu/Zn at neutral pH.179 They found that compared with the naked iron and zinc, the selectivity of N2 production is highly promoted after the bimetals deposition.
Different from the reduction of NO3− and NO2−, the final products are often halogen anions (Cl− and Br−) in the reduction of halogen oxyanions, because of the high reactivity of the intermediate products (such as ClO− and Cl2). Bimetals are good candidates for the catalytic reduction of halogen oxyanions. Choe et al. has proposed that a Re/Pd bimetal catalyst may be useful for the treatment of perchlorate-contaminated water, especially as part of a hybrid ion exchange/catalytic reduction process.180 They investigated the influence of rhenium speciation on the stability and activity of Re/Pd bimetals for the catalytic reduction of perchlorate.180 Re sorption under different redox conditions result in the different Re speciation on the catalyst outer surface. As for practical applications, it is recommended that Re/Pd with lower Re contents should be used and longer-term exposure to oxic waters are avoided to ensure longer catalyst lifetimes. Based on this conclusion, Liu et al. further studied the application of a Re–Pd bimetallic catalyst for treatment of perchlorate in waste ion-exchange regenerant brine.181 Results showed that the co-contamination of the ion-exchange waste brine by excess NO3− was the major cause of the deactivation of the Re–Pd catalyst. As a pre-treatment of NO3−, a separate bimetallic catalyst (In–Pd/Al2O3) was used to improve the selectivity for N2 over NH4+ and enabled facile ClO4− reduction by the Re–Pd catalyst, with which making this sequential catalytic treatment a promising strategy for enabling reuse of waste ion-exchange brine containing NO3− and ClO4− (Fig. 9).
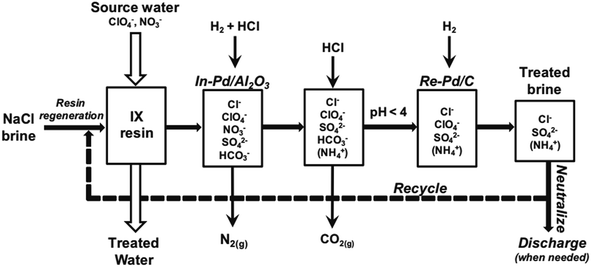 |
| Fig. 9 Sequential catalytic treatment strategy for recycling waste ion-exchange regenerant brines contaminated by ClO4− and NO3− (adapted with permission from literature 181. Copyright (2013) Elsevier). | |
3.5.2 Anions and oxyanions removed by bimetal oxides. Compared with the bimetal, bimetal oxides usually serve as a sorbents in the removal of anions and oxyanions. Moriyama et al. used the Mg–Al bimetal oxides as a sorbents for the removal of F− in aqueous solutions.116 They found that a higher calcinations temperature will result in the formation of the additional MgAl2O4 phase, which did not contribute to the immobilization of F−. Thakre et al. investigated the performance of the chitosan based mesoporous Ti/Al binary metal oxide supported beads for fluoride removal from drinking water.182 The excellent defluoridation capacity with negligible release of aluminium and titanium ions and the highly reusability of the adsorbent shows its promising application in practice.As phosphate is a necessary nutrient for the growth of most organisms, the removal of phosphate from water is of great importance to control eutrophication. Lu et al. examined the performance of a nano-structured Fe/Ti bimetal oxide sorbent in the removal of phosphate from aqueous solution.183 They found that the Fe/Ti bimetal oxide prepared with a Fe/Ti molar ratio of 20
:
1 was the optimal sorbent and its Langmuir sorption capacity for phosphate was 35.4 mg g−1 at pH 6.8, which excel most of reported Fe oxide-based sorbents. The main mechanism of phosphate absorption was the replacement of surface hydroxyl groups by phosphate via formation of innersphere surface complex.
4 Remarks and conclusions
There has been great interest in the use of bimetals and bimetal oxides for the removal of environmental contaminants and encouraging treatment efficiencies have been documented. This paper gives an overview of the recent advances of the synthesis methods and application of bimetals and bimetal oxides.
Catalytic properties of bimetals and bimetal oxides depend on their structure, size, shape, composition, and surface property, all of which are affected by different preparation procedures. Among the synthesis methods of bimetals, chemical methods are more widely used, however, they often occur in presence of toxic solvents and reducing agents. Physical methods are green and environmentally friendly, however, they often require complicated equipments. Biosynthesis method using non-toxic and eco-friendly reductants is frequently applied to synthesize bimetallic NPs. Among all the methods to synthesize bimetal oxides, precipitation method is a popular and widely used method. The addition of dispersing agents and stabilizers can overcome the disadvantages of agglomeration during the preparation. Hydrothermal method can prepare bimetallic oxides with porous structures and particular morphologies from metal salts. Sol–gel method is a low cost and simple method to prepare bimetal oxides with excellent compositional control, high homogeneity at the molecular level, and low crystallisation temperature.88 However, sol–gel method needs calcination, which is similar to impregnation method. Spray pyrolysis method can prepare bimetal oxides effectively and easily, however, it needs a high reaction temperature and an equipment to spray precursors. Precipitation method is beneficial to prepare amorphous bimetallic oxides and the specific surface area of the oxides is affected by the calcination temperature.
To sum up, current preparation methods for bimetals and bimetal oxides are often complex and high cost. So the future research may decrease the cost by finding new low-cost raw materials, and develop more simple and practicable methods.
The application of bimetal or bimetal oxides in the removal of contaminants have achieved great success, however, the roles of bimetals or bimetal oxides played in the removal of these contaminants are not the same. In bimetals, a substrate metal combines with a second metal M, which serves as a promising alternative hydrodechlorination and hydrogenation reagent and a storage of the active hydrogen species. Its remarkable catalytic function turns bimetals into good catalysts for the hydrodechlorination of COCs, nitroaromatic compounds, and azo dyes. Considering the high reducibility of bimetals, they are also employed as reductants for the removal of Cr(VI) and some oxyanions. In the bimetal oxides, the metal oxide with low reactivity and low cost are used as host metal oxides, while the other metal oxide with high reactivity acts as functional metal oxides. Bimetal oxides with the combination of advantages of two oxides not only can be good sorbents, but also can be good reductants/oxidants or catalysts in the removal of contaminants.
The leaching of metal ions from bimetals or bimetal oxides raises concern on the decreased catalyst longevity, high costs, and potential secondary metal contamination to the treated wastewater. So explore methods to decrease the metal leaching is another future research.
Acknowledgements
This research was supported by the National Natural Science Foundation of China (No. 51008084), Development Program for Outstanding Young Teachers in Guangdong Province (No. Yq2013055), and Guangzhou Pearl River Nova Program (No. 2012J2200097).
References
- Y. Han, Z. Chen, L. Tong, L. Yang, J. Shen, B. Wang, Y. Liu, Y. Liu and Q. Chen, Water Res., 2013, 47, 216–224 CrossRef CAS PubMed.
- V. Bokare, J. Jung, Y. Y. Chang and Y. S. Chang, J. Hazard. Mater., 2013, 250–251, 397–402 CrossRef CAS PubMed.
- L. Cong, J. Guo, J. Liu, H. Shi and M. Wang, J. Environ. Manage., 2015, 150, 451–455 CrossRef CAS PubMed.
- W. Liu, H. Zhang, B. Cao, K. Lin and J. Gan, Water Res., 2011, 45, 1872–1878 CrossRef CAS PubMed.
- C. C. Wu, L. C. Hus, P. N. Chiang, J. C. Liu, W. H. Kuan, C. C. Chen, Y. M. Tzou, M. K. Wang and C. E. Hwang, Water Res., 2013, 47, 2583–2591 CrossRef CAS PubMed.
- Z. Cheng, F. Fu, Y. Pang, B. Tang and J. Lu, Chem. Eng. J., 2015, 260, 284–290 CrossRef CAS PubMed.
- Y. Li, W. Cheng, G. Sheng, J. Li, H. Dong, Y. Chen and L. Zhu, Appl. Catal., B, 2015, 174–175, 329–335 CrossRef CAS PubMed.
- Y. Liu, Y. Zhang and B. J. Ni, Water Res., 2015, 75, 292–300 CrossRef CAS PubMed.
- L. Ling, B. Pan and W. Zhang, Water Res., 2015, 71, 274–281 CrossRef CAS PubMed.
- L. Ling and W. Zhang, Environ. Sci. Technol. Lett., 2014, 1, 305–309 CrossRef CAS.
- S. B. Mortazavi, B. Ramavandi and G. Moussavi, Environ. Technol., 2011, 32, 251–260 CrossRef CAS PubMed.
- C. C. Huang, S. L. Lo and H. L. Lien, Chem. Eng. J., 2015, 273, 413–420 CrossRef CAS PubMed.
- Q. Huang, W. Liu, P. Peng and W. Huang, J. Hazard. Mater., 2013, 262, 634–641 CrossRef CAS PubMed.
- Z. Wang, W. Huang, P. Peng and D. E. Fennell, Chem. Eng. J., 2015, 273, 465–471 CrossRef CAS PubMed.
- H. J. Hong, W. Farooq, J. S. Yang and J. W. Yang, Sep. Sci. Technol., 2010, 45, 1975–1981 CrossRef CAS PubMed.
- L. H. Chen, C. C. Huang and H. L. Lien, Chemosphere, 2008, 73, 692–697 CrossRef CAS PubMed.
- C. J. Lin, Y. H. Liou and S. L. Lo, Chemosphere, 2009, 74, 314–319 CrossRef PubMed.
- L. Ma, Z. Ding, T. Gao, R. Zhou, W. Xu and J. Liu, Chemosphere, 2004, 55, 1207–1212 CrossRef CAS PubMed.
- W. Fang, C. Xu, J. Zheng, G. Chen and K. Jiang, RSC Adv., 2015, 5, 39612–39619 CAS.
- Y. Wang, X. Zhao, W. Gao, L. Chen, S. Chen, M. Wei, M. Gao, C. Wang, Y. Zhang and J. Yang, RSC Adv., 2015, 5, 7454–7460 RSC.
- J. Zhao, J. Zeng, X. Cheng, L. Wang, H. Yang and B. Shen, RSC Adv., 2015, 5, 16727–16734 RSC.
- B. Yang, J. Zhang, Y. Zhang, S. Deng, G. Yu, J. Wu, H. Zhang and J. Liu, Chem. Eng. J., 2014, 250, 222–229 CrossRef CAS PubMed.
- Z. Wen, Y. Zhang, C. Dai and Z. Sun, J. Hazard. Mater., 2015, 287, 225–233 CrossRef CAS PubMed.
- Z. Wen, C. Dai, Y. Zhu and Y. Zhang, RSC Adv., 2015, 5, 4058–4068 RSC.
- J. P. Lee, D. Chen, X. Li, S. Yoo, L. A. Bottomley, M. A. El-Sayed, S. Park and M. Liu, Nanoscale, 2013, 5, 11620–11624 RSC.
- Z. Wen, Y. Zhang, C. Dai, B. Chen, S. Guo, H. Yu and D. Wu, Microporous Mesoporous Mater., 2014, 200, 235–244 CrossRef CAS PubMed.
- B. Gunawardana, N. Singhal and P. Swedlund, Environ. Eng. Res., 2011, 16, 187–203 CrossRef.
- P. Xu, G. Zeng, D. Huang, C. Feng, S. Hu, M. Zhao, C. Lai, Z. Wei, C. Huang, G. Xie and Z. Liu, Sci. Total Environ., 2012, 424, 1–10 CrossRef CAS PubMed.
- D. O'Carroll, B. Sleep, M. Krol, H. Boparai and C. Kocur, Adv. Water Resour., 2013, 51, 104–122 CrossRef PubMed.
- W. Liu, T. Qian and H. Jiang, Chem. Eng. J., 2014, 236, 448–463 CrossRef CAS PubMed.
- D. Wang and Y. Li, Adv. Mater., 2011, 23, 1044–1060 CrossRef CAS PubMed.
- M. Sankar, N. Dimitratos, P. J. Miedziak, P. P. Wells, C. J. Kiely and G. J. Hutchings, Chem. Soc. Rev., 2012, 41, 8099–8139 RSC.
- X. Cao, N. Wang, S. Jia, L. Guo and K. Li, Biosens. Bioelectron., 2013, 39, 226–230 CrossRef CAS PubMed.
- T. Han, Y. Zhang, J. Xu, J. Dong and C. C. Liu, Sens. Actuators, B, 2015, 207, 404–412 CrossRef CAS PubMed.
- Y. Pan, X. Guo, M. Li, Y. Liang, Y. Wu, Y. Wen and H. Yang, Electrochim. Acta, 2015, 159, 40–45 CrossRef CAS PubMed.
- M. Khan, A. B. Yousaf, M. Chen, C. Wei, X. Wu, N. Huang, Z. Qi and L. Li, J. Power Sources, 2015, 282, 520–528 CrossRef CAS PubMed.
- N. V. Long, T. D. Hien, T. Asaka, M. Ohtaki and M. Nogami, Int. J. Hydrogen Energy, 2011, 36, 8478–8491 CrossRef PubMed.
- H. Chen, S. Wang, M. Xiao, D. Han, Y. Lu and Y. Meng, Chin. J. Chem. Eng., 2012, 20, 906–913 CrossRef CAS.
- W. Bhaathsingh and A. S. Nesaraj, Trans. Nonferrous Met. Soc. China, 2013, 23, 128–133 CrossRef.
- T. Yang, C. M. R. de Almeida, D. Ramasamy and F. J. A. Loureiro, J. Power Sources, 2014, 269, 46–53 CrossRef CAS PubMed.
- F. Yang, J. Wang, Y. Cao, L. Zhang and X. Zhang, Sens. Actuators, B, 2014, 205, 20–25 CrossRef CAS PubMed.
- C. Huang, X. Yang, H. Yang, P. Huang, H. Song and S. Liao, Appl. Surf. Sci., 2014, 315, 138–143 CrossRef CAS PubMed.
- C. Han, L. Wu, L. Ge, Y. Li and Z. Zhao, Carbon, 2015, 92, 31–40 CrossRef CAS PubMed.
- Y. Feng, J. Hao, W. Liu, Y. Yao, Y. Cheng and H. Xu, Chin. Chem. Lett., 2015, 26, 709–713 CrossRef CAS PubMed.
- S. Chen, S. V. Jenkins, J. Tao, Y. Zhu and J. Chen, J. Phys. Chem. C, 2013, 117, 8924–8932 CAS.
- J. A. Adekoya, E. O. Dare, M. A. Mesubi and N. Revaprasadu, Phys. E, 2015, 71, 70–78 CrossRef CAS PubMed.
- F. H. Ko, M. R. Tai, F. K. Liu and Y. C. Chang, Sens. Actuators, B, 2015, 211, 283–289 CrossRef CAS PubMed.
- L. Han, P. Cui, H. He, H. Liu, Z. Peng and J. Yang, J. Power Sources, 2015, 286, 488–494 CrossRef CAS PubMed.
- H. Yu and Y. He, Sens. Actuators, B, 2015, 209, 877–882 CrossRef CAS PubMed.
- J. Zhang, L. Zhang, Y. Jia, G. Chen, X. Wang, Q. Kuang, Z. Xie and L. Zheng, Nano Res., 2012, 5, 618–629 CrossRef CAS.
- K. Yu, T. Yao, Z. Pan, S. Wei and Y. Xie, Dalton Trans., 2009, 46, 10353–10358 RSC.
- M. Fan, F. Xu and L. Sun, Int. J. Hydrogen Energy, 2007, 32, 2809–2815 CrossRef CAS PubMed.
- J. L. Coutts, R. W. Devor, B. Aitken, M. D. Hampton, J. W. Quinn, C. A. Clausen and C. L. Geiger, J. Hazard. Mater., 2011, 192, 1380–1387 CrossRef CAS PubMed.
- Z. Zaranski and T. Czujko, J. Alloys Compd., 2011, 509S, S608–S611 CrossRef PubMed.
- C. Kan, J. Zhu and C. Wang, J. Cryst. Growth, 2009, 311, 1565–1570 CrossRef CAS PubMed.
- S. Anandan, F. Grieser and M. Ashokkumar, J. Phys. Chem. C, 2008, 112, 15102–15105 CAS.
- D. C. Martínez-Casillas and O. Solorza-Feria, ECS Trans., 2011, 36, 565–571 Search PubMed.
- K. Torigoe, H. Remita, P. Beaunier and J. Belloni, Radiat. Phys. Chem., 2002, 64, 215–222 CrossRef CAS.
- C. M. Doudna, M. F. Bertino and A. T. Tokuhiro, Langmuir, 2002, 18, 2434–2435 CrossRef CAS.
- C. M. Doudna, M. F. Bertino, F. D. Blum, A. T. Tokuhiro, D. Lahiri-Dey, S. Chattopadhyay and J. Terry, J. Phys. Chem. B, 2003, 107, 2966–2970 CrossRef CAS.
- S. Remita, G. Picq, J. Khatouri and M. Mostafavi, Radiat. Phys. Chem., 1999, 54, 463–473 CrossRef CAS.
- M. Mirdamadi-Esfahani, M. Mostafavi, B. Keita, L. Nadjo, P. Kooyman and H. Remita, Gold Bull., 2010, 43, 49–56 CrossRef CAS.
- G. Zhan, J. Huang, M. Du, I. Abdul-Rauf, Y. Ma and Q. Li, Mater. Lett., 2011, 65, 2989–2991 CrossRef CAS PubMed.
- D. S. Sheny, J. Mathew and D. Philip, Spectrochim. Acta, Part A, 2011, 79, 254–262 CrossRef CAS PubMed.
- M. M. Kumari, J. Jacob and D. Philip, Spectrochim. Acta, Part A, 2015, 137, 185–192 CrossRef PubMed.
- S. Mondal, N. Roy, R. A. Laskar, I. Sk, S. Basu, D. Mandal and N. A. Begum, Colloids Surf., B, 2011, 82, 497–504 CrossRef CAS PubMed.
- C. Tamuly, M. Hazarika, S. C. Borah, M. R. Das and M. P. Boruah, Colloids Surf., B, 2013, 102, 627–634 CrossRef CAS PubMed.
- P. S. Schabes-Retchkiman, G. Canizal, R. Herrera-Becerra, C. Zorrilla, H. B. Liu and J. A. Ascencio, Opt. Mater., 2006, 29, 95–99 CrossRef CAS PubMed.
- H. R. Mahmoud, S. A. El-Molla and M. Saif, Powder Technol., 2013, 249, 225–233 CrossRef CAS PubMed.
- A. Kambur, G. S. Pozan and I. Boz, Appl. Catal., B, 2012, 115–116, 149–158 CrossRef CAS PubMed.
- M. Polat, A. M. Soylu, D. A. Erdogan, H. Erguven, E. I. Vovk and E. Ozensoy, Catal. Today, 2015, 241, 25–32 CrossRef CAS PubMed.
- B. An and D. Zhao, J. Hazard. Mater., 2012, 211–212, 332–341 CrossRef CAS PubMed.
- W. Zhan, X. Zhang, Y. Guo, L. Wang, Y. Guo and G. Lu, J. Rare Earths, 2014, 32, 146–152 CrossRef CAS.
- S. Somacescu, A. Dinescu, A. Stanoiu, C. E. Simion and J. M. C. Moreno, Mater. Lett., 2012, 89, 219–222 CrossRef CAS PubMed.
- Y. Su, W. Yang, W. Sun, Q. Li and J. K. Shang, Chem. Eng. J., 2015, 268, 270–279 CrossRef CAS PubMed.
- M. Najafi, A. Abbasi, M. Masteri-Farahani and J. Janczak, Polyhedron, 2015, 93, 76–83 CrossRef CAS PubMed.
- C. Liu, H. Liu, C. Yin, X. Zhao, B. Liu, X. Li, Y. Li and Y. Liu, Fuel, 2015, 154, 88–94 CrossRef CAS PubMed.
- H. Cui, J. Cai, H. Zhao, B. Yuan, C. Ai and M. Fu, J. Hazard. Mater., 2014, 279, 26–31 CrossRef CAS PubMed.
- H. Uchiyama, K. Matsumoto and H. Kozuka, J. Cryst. Growth, 2012, 338, 201–207 CrossRef CAS PubMed.
- C. H. Wu, J. S. Ma and C. H. Lu, Curr. Appl. Phys., 2012, 12, 1190–1194 CrossRef PubMed.
- M. M. Ibrahim, Spectrochim. Acta, Part A, 2015, 145, 487–492 CrossRef CAS PubMed.
- G. A. El-Shobaky, H. G. El-Shobaky, A. A. A. Badawy and Y. M. Fahmy, Appl. Catal., A, 2011, 409–410, 234–238 CrossRef CAS PubMed.
- S. M. Saqer, D. I. Kondarides and X. E. Verykios, Appl. Catal., B, 2011, 103, 275–286 CrossRef CAS PubMed.
- M. Cui, Y. Li, X. Wang, J. Wang and M. Shen, J. Rare Earths, 2013, 31, 572–576 CrossRef CAS.
- M. Konsolakis, M. Sgourakis and S. A. C. Carabineiro, Appl. Surf. Sci., 2015, 341, 48–54 CrossRef CAS PubMed.
- W. Zhang, R. Li and H. He, Int. J. Photoenergy, 2012, 2012, 108175 Search PubMed.
- A. Darmawan, J. Motuzas, S. Smart, A. Julbe and J. C. D. da Costa, J. Membr. Sci., 2015, 474, 32–38 CrossRef CAS PubMed.
- M. R. Mohammadi and D. J. Fray, Sens. Actuators, B, 2011, 155, 568–576 CrossRef CAS PubMed.
- A. Barrera, S. Fuentes, G. Díaz, A. Gómez-Cortés, F. Tzompantzi and J. C. Molina, Fuel, 2012, 93, 136–141 CrossRef CAS PubMed.
- N. A. Oladoja and A. L. Ahmad, Sep. Purif. Technol., 2013, 116, 230–239 CrossRef CAS PubMed.
- T. Block, N. Knoblauch and M. Schmücker, Thermochim. Acta, 2014, 577, 25–32 CrossRef CAS PubMed.
- J. B. Branco, A. C. Ferreira and J. P. Leal, J. Mol. Catal. A: Chem., 2014, 390, 45–51 CrossRef CAS PubMed.
- M. Zahedi, N. Roohpour and A. K. Ray, J. Alloys Compd., 2014, 582, 277–282 CrossRef CAS PubMed.
- S. Khan, J. Kim, A. Sotto and B. van der Bruggen, J. Ind. Eng. Chem., 2015, 21, 779–786 CrossRef CAS PubMed.
- M. M. Bagheri-Mohagheghi and M. Shokooh-Saremi, Thin Solid Films, 2003, 441, 238–242 CrossRef CAS.
- L. M. Berger, F. L. Toma, S. Scheitz, R. Trache and T. Börner, Materialwiss. Werkstofftech., 2014, 45, 465–475 CrossRef CAS PubMed.
- S. Salem, S. H. Jazayeri, F. Bondioli, A. Allahverdi and M. Shirvani, Thermochim. Acta, 2011, 521, 191–196 CrossRef CAS PubMed.
- S. Salem, J. Ind. Eng. Chem., 2014, 20, 818–823 CrossRef CAS PubMed.
- L. Zhang, S. Zhu, H. Cao, G. Pang, J. Lin, L. Hou and C. Yuan, RSC Adv., 2015, 5, 13667–13673 RSC.
- Z. Ren, G. Zhang and J. P. Chen, J. Colloid Interface Sci., 2011, 358, 230–237 CrossRef CAS PubMed.
- L. Chai, Y. Wang, N. Zhao, W. Yang and X. You, Water Res., 2013, 47, 4040–4049 CrossRef CAS PubMed.
- Z. Quan, E. Ni, S. Hayashi and N. Sonoyama, J. Mater. Chem. A, 2013, 1, 8848–8856 CAS.
- L. Yang, Y. Zhang, X. Liu, X. Jiang, Z. Zhang, T. Zhang and L. Zhang, Chem. Eng. J., 2014, 246, 88–96 CrossRef CAS PubMed.
- Q. Guo, B. Mei, S. Zhou, Z. Shi, Y. Feng, J. Wu, G. Yan and L. Li, J. Non-Cryst. Solids, 2009, 355, 922–925 CrossRef CAS PubMed.
- W. Xie, Q. Liang, T. Qian and D. Zhao, Water Res., 2015, 70, 485–494 CrossRef CAS PubMed.
- W. Xu, H. Wang, R. Liu, X. Zhao and J. Qu, J. Colloid Interface Sci., 2011, 363, 320–326 CrossRef CAS PubMed.
- F. Long, J. Gong, G. Zeng, L. Chen, X. Wang, J. Deng, Q. Niu, H. Zhang and X. Zhang, Chem. Eng. J., 2011, 171, 448–455 CrossRef CAS PubMed.
- Z. Shu, Y. Chen, W. Huang, X. Cui, L. Zhang, H. Chen, G. Zhang, X. Fan, Y. Wang, G. Tao, D. He and J. Shi, Appl. Catal., B, 2013, 140–141, 42–50 CrossRef CAS PubMed.
- J. Xie, Z. Qu, N. Yan, S. Yang, W. Chen, L. Hu, W. Huang and P. Liu, J. Hazard. Mater., 2013, 261, 206–213 CrossRef CAS PubMed.
- S. K. Swain, T. Patnaik, P. C. Patnaik, U. Jha and R. K. Dey, Chem. Eng. J., 2013, 215–216, 763–771 CrossRef PubMed.
- G. Zhang, A. Khorshed and J. P. Chen, J. Colloid Interface Sci., 2013, 397, 137–143 CrossRef CAS PubMed.
- C. Shan and M. Tong, Water Res., 2013, 47, 3411–3421 CrossRef CAS PubMed.
- A. E. Awadallah, M. S. Mostafa, A. A. Aboul-Enein and S. A. Hanafi, Fuel, 2014, 129, 68–77 CrossRef CAS PubMed.
- J. Xie, H. Xu, Z. Qu, W. Huang, W. Chen, Y. Ma, S. Zhao, P. Liu and N. Yan, J. Colloid Interface Sci., 2014, 428, 121–127 CrossRef CAS PubMed.
- H. R. Mahmoud, J. Mol. Catal. A: Chem., 2014, 392, 216–222 CrossRef CAS PubMed.
- S. Moriyama, K. Sasaki and T. Hirajima, Chemosphere, 2014, 95, 597–603 CrossRef CAS PubMed.
- J. Luo, J. Hu, X. Wei, L. Fu and L. Li, Chemosphere, 2015, 131, 17–33 CrossRef CAS PubMed.
- B. Yang, S. Deng, G. Yu, H. Zhang, J. Wu and Q. Zhuo, J. Hazard. Mater., 2011, 189, 76–83 CrossRef CAS PubMed.
- N. Zhu, H. Luan, S. Yuan, J. Chen, X. Wu and L. Wang, J. Hazard. Mater., 2010, 176, 1101–1105 CrossRef CAS PubMed.
- Z. Zhang, Q. Shen, N. Cissoko, J. Wo and X. Xu, J. Hazard. Mater., 2010, 182, 252–258 CrossRef CAS PubMed.
- H. Tian, J. Li, Z. Mu, L. Li and Z. Hao, Sep. Purif. Technol., 2009, 66, 84–89 CrossRef CAS PubMed.
- H. Zhou, S. Wang and G. D. Sheng, J. Zhejiang Univ., Sci., A, 2010, 11, 356–362 CrossRef CAS.
- J. H. Choi and Y. H. Kim, J. Hazard. Mater., 2009, 166, 984–991 CrossRef CAS PubMed.
- X. Nie, J. Liu, D. Yue, X. Zeng and Y. Nie, Chemosphere, 2013, 90, 2403–2407 CrossRef CAS PubMed.
- B. Yang, S. Deng, G. Yu, Y. Lu, H. Zhang, J. Xiao, G. Chen, X. Cheng and L. Shi, Chem. Eng. J., 2013, 219, 492–498 CrossRef CAS PubMed.
- C. Lin, Y. Shih and J. MacFarlane, Chem. Eng. J., 2015, 262, 59–67 CrossRef CAS PubMed.
- V. Nagpal, A. D. Bokare, R. C. Chikate, C. V. Rode and K. M. Paknikar, J. Hazard. Mater., 2010, 175, 680–687 CrossRef CAS PubMed.
- G. N. Jovanovic, J. E. Atwater, P. Ž. Plazl and I. Plazl, Chem. Eng. J., 2015, 274, 50–60 CrossRef CAS PubMed.
- S. Bleyl, F. D. Kopinke and K. Mackenzie, Chem. Eng. J., 2012, 191, 588–595 CrossRef CAS PubMed.
- X. Wang, C. Chen, Y. Chang and H. Liu, J. Hazard. Mater., 2009, 161, 815–823 CrossRef CAS PubMed.
- R. DeVor, K. C. Knighton, B. Aitken, P. Maloney, E. Holland, L. Talalaj, R. Fidler, S. Elsheimer, C. A. Clausen and C. L. Geiger, Chemosphere, 2008, 73, 896–900 CrossRef CAS PubMed.
- B. Yang, Y. Zhang, S. Deng, G. Yu, Y. Lu, J. Wu, J. Xiao, G. Chen, X. Cheng and L. Shi, Chem. Eng. J., 2013, 234, 346–353 CrossRef CAS PubMed.
- S. Agarwal, S. R. Al-Abed and D. D. Dionysiou, Appl. Catal., B, 2009, 92, 17–22 CrossRef CAS PubMed.
- X. Li, X. Dou and J. Li, J. Environ. Sci., 2012, 24, 1197–1203 CrossRef CAS.
- Q. Zhou, C. Tang, Y. Wang and L. Zheng, Fuel, 2004, 83, 1727–1732 CrossRef CAS PubMed.
- X. Ma, M. Zheng, W. Liu, Y. Qian, X. Zhao and B. Zhang, Chemosphere, 2005, 60, 796–801 CrossRef CAS PubMed.
- M. Matouq, N. Jildeh, M. Qtaishat, M. Hindiyeh and M. Q. Al Syouf, J. Environ. Chem. Eng., 2015, 3, 775–784 CrossRef CAS PubMed.
- F. Fu and Q. Wang, J. Environ. Manage., 2011, 92, 407–418 CrossRef CAS PubMed.
- M. R. Huguet and W. D. Marshall, J. Hazard. Mater., 2009, 169, 1081–1087 CrossRef PubMed.
- X. Weng, S. Lin, Y. Zhong and Z. Chen, Chem. Eng. J., 2013, 229, 27–34 CrossRef CAS PubMed.
- Y. Kuang, J. Du, R. Zhou, Z. Chen, M. Megharaj and R. Naidu, J. Colloid Interface Sci., 2015, 447, 85–91 CrossRef CAS PubMed.
- B. S. Kadu, Y. D. Sathe, A. B. Ingle, R. C. Chikate, K. R. Patil and C. V. Rode, Appl. Catal., B, 2011, 104, 407–414 CrossRef CAS PubMed.
- Z. Zhang, M. Liao, H. Zeng, S. Xu, X. Liu, J. Du, P. Zhu and Q. Huang, Appl. Clay Sci., 2014, 102, 246–253 CrossRef CAS PubMed.
- L. Jiang, S. Xiao and J. Chen, Colloids Surf., A, 2015, 479, 1–10 CrossRef CAS PubMed.
- K. P. Singh, A. K. Singh, S. Gupta and S. Sinha, Desalination, 2011, 270, 275–284 CrossRef CAS PubMed.
- Y. Su, A. S. Adeleye, Y. Huang, X. Sun, C. Dai, X. Zhou, Y. Zhang and A. A. Keller, Water Res., 2014, 63, 102–111 CrossRef CAS PubMed.
- M. Ranđelović, M. Purenović, A. Zarubica, J. Purenović, B. Matović and M. Momčilović, J. Hazard. Mater., 2012, 199–200, 367–374 CrossRef PubMed.
- E. T. Wong, K. H. Chan and A. Idris, Chem. Eng. J., 2015, 268, 311–324 CrossRef CAS PubMed.
- B. Hu, W. Liu, W. Gao, J. Han, H. Liu and L. A. Lucia, Chem. Eng. J., 2015, 277, 150–158 CrossRef CAS PubMed.
- R. Liu, F. Liu, C. Hu, Z. He, H. Liu and J. Qu, J. Hazard. Mater., 2015, 300, 847–854 CrossRef CAS PubMed.
- K. Wu, T. Liu, W. Xue and X. Wang, Chem. Eng. J., 2012, 192, 343–349 CrossRef CAS PubMed.
- E. B. Simsek, E. Özdemir and U. Beker, Chem. Eng. J., 2013, 220, 402–411 CrossRef CAS PubMed.
- T. Basu and U. C. Ghosh, Appl. Surf. Sci., 2013, 283, 471–481 CrossRef CAS PubMed.
- Y. Zhang, M. Yang, X. Dou, H. He and D. Wang, Environ. Sci. Technol., 2005, 39, 7246–7253 CrossRef CAS.
- Y. T. Chan, W. H. Kuan, T. Y. Chen and M. K. Wang, Water Res., 2009, 43, 4412–4420 CrossRef CAS PubMed.
- T. Basu, K. Gupta and U. C. Ghosh, Chem. Eng. J., 2012, 183, 303–314 CrossRef CAS PubMed.
- G. Zhang, J. Qu, H. Liu, R. Liu and R. Wu, Water Res., 2007, 41, 1921–1928 CrossRef CAS PubMed.
- G. Zhang, F. Liu, H. Liu, J. Qu and R. Liu, Environ. Sci. Technol., 2014, 48, 10316–10322 CrossRef CAS PubMed.
- S. Zhang, H. Niu, Y. Cai, X. Zhao and Y. Shi, Chem. Eng. J., 2010, 158, 599–607 CrossRef CAS PubMed.
- W. Sun, W. Pan, F. Wang and N. Xu, Chem. Eng. J., 2015, 273, 353–362 CrossRef CAS PubMed.
- R. L. Singh, P. K. Singh and R. P. Singh, Int. Biodeterior. Biodegrad., 2015, 104, 21–31 CrossRef CAS PubMed.
- J. Gong, C. S. Lee, Y. Y. Chang and Y. S. Chang, J. Hazard. Mater., 2015, 286, 107–117 CrossRef CAS PubMed.
- A. Koutsospyros, J. Pavlov, J. Fawcett, D. Strickland, B. Smolinski and W. Braida, J. Hazard. Mater., 2012, 219–220, 75–81 CrossRef CAS PubMed.
- X. Xu, H. Zhou and M. Zhou, Chemosphere, 2006, 62, 847–852 CrossRef CAS PubMed.
- V. K. Gupta, N. Atar, M. L. Yola, Z. Üstündağ and L. Uzun, Water Res., 2014, 48, 210–217 CrossRef CAS PubMed.
- A. C. K. Yip, F. L. Y. Lam and X. Hu, Chem. Eng. Sci., 2007, 62, 5150–5153 CrossRef CAS PubMed.
- Z. Xiong, B. Lai, P. Yang, Y. Zhou, J. Wang and S. Fang, J. Hazard. Mater., 2015, 297, 261–268 CrossRef CAS PubMed.
- E. Kim, H. S. Jeong and B. M. Kim, Catal. Commun., 2014, 45, 25–29 CrossRef CAS PubMed.
- A. D. Bokare, R. C. Chikate, C. V. Rode and K. M. Paknikar, Appl. Catal., B, 2008, 79, 270–278 CrossRef CAS PubMed.
- C. Cai, L. Wang, H. Gao, L. Hou and H. Zhang, J. Environ. Sci., 2014, 26, 1267–1273 CrossRef CAS.
- C. Cai, H. Zhang, X. Zhong and L. Hou, J. Hazard. Mater., 2015, 283, 70–79 CrossRef CAS PubMed.
- L. Hua, H. Ma and L. Zhang, Chemosphere, 2013, 90, 143–149 CrossRef CAS PubMed.
- J. R. Chiou, B. H. Lai, K. C. Hsu and D. H. Chen, J. Hazard. Mater., 2013, 248–249, 394–400 CrossRef CAS PubMed.
- A. Safavi and S. Momeni, J. Hazard. Mater., 2012, 201–202, 125–131 CrossRef CAS PubMed.
- F. L. Theiss, S. J. Couperthwaite, G. A. Ayoko and R. L. Frost, J. Colloid Interface Sci., 2014, 417, 356–368 CrossRef CAS PubMed.
- R. Epsztein, O. Nir, O. Lahav and M. Green, Chem. Eng. J., 2015, 279, 372–378 CrossRef CAS PubMed.
- K. H. Goh and T. T. Lim, J. Hazard. Mater., 2010, 180, 401–408 CrossRef CAS PubMed.
- H. Ren, S. Jia, J. Zou, S. Wu and X. Han, Appl. Catal., B, 2015, 176–177, 53–61 CrossRef CAS PubMed.
- Y. H. Liou, C. J. Lin, I. C. Hung, S. Y. Chen and S. L. Lo, Chem. Eng. J., 2015, 181–182, 236–242 CrossRef PubMed.
- J. K. Choe, J. R. Shapley, T. J. Strathmann and C. J. Werth, Environ. Sci. Technol., 2010, 44, 4716–4721 CrossRef CAS PubMed.
- J. Liu, J. K. Choe, Z. Sasnow, C. J. Werth and T. J. Strathmann, Water Res., 2013, 47, 91–101 CrossRef CAS PubMed.
- D. Thakre, S. Jagtap, N. Sakhare, N. Labhsetwar, S. Meshram and S. Rayalu, Chem. Eng. J., 2010, 158, 315–324 CrossRef CAS PubMed.
- J. Lu, D. Liu, J. Hao, G. Zhang and B. Lu, Chem. Eng. Res. Des., 2015, 93, 652–661 CrossRef CAS PubMed.
|
This journal is © The Royal Society of Chemistry 2015 |
Click here to see how this site uses Cookies. View our privacy policy here.