DOI:
10.1039/C5RA13723C
(Paper)
RSC Adv., 2015,
5, 65481-65486
Cost-effective synthesis of α-carboline/pyridine hybrid bipolar host materials with improved electron-transport ability for efficient blue phosphorescent OLEDs
Received
8th June 2015
, Accepted 24th July 2015
First published on 24th July 2015
Abstract
Two α-carboline/pyridine hybrid bipolar host materials, 2,6-bis(9-H-pyrido[2,3-b]indol-9-yl)pyridine (2,6-CbPy) and 9,9′,9′′-(pyridine-2,4,6-triyl)tris(9-H-pyrido[2,3-b]indole) (2,4,6-CbPy) are designed and synthesized through an efficient one-step catalyst-free C–N coupling reaction. The thermal, electrochemical and photoluminescence properties of the two host materials have been fully investigated. The introduction of the α-carboline instead of carbazole in 2,6-CbPy and 2,4,6-CbPy significantly improves the electron transporting abilities, and simultaneously maintains the good hole transporting properties with 2,6-CzPy and 2,4,6-CzPy. By using them as hosts for FIrpic-doped blue phosphorescent OLEDs, a maximum current efficiency of 44.1 cd A−1 and power efficiency of 38.5 lm W−1 has been achieved for the 2,4,6-CbPy-based device. The maximum current efficiency is higher than 42.1 cd A−1 of the carbazole-containing 2,4,6-CzPy under the same device conditions.
1. Introduction
Phosphorescent organic light-emitting diodes (PHOLEDs) continue to attract intensive attention by using heavy-metal complexes as the phosphor emitters, which can achieve 100% internal quantum efficiency by harvesting both the electro-generated singlet (25%) and triplet (75%) excitons for emission.1 To avoid competitive de-excitation pathways like triplet–triplet annihilation and/or concentration quenching, the emissive phosphors are usually dispersed into host materials as dopants at low doping concentrations, and thus the development of host materials is of equal importance to the emissive phosphors.2 Of the three primary colors (blue, green and red), the efficiency and stability of blue PHOLEDs are still unsatisfactory.3 To avoid energy feedback from the phosphorescent blue dopant, the triplet energy (ET) of the host materials should be above 2.65 eV. As a result, the design of host materials suitable for blue PHOLEDs is more challenging.
It is expected that the host materials exhibit bipolar characteristics by simultaneously containing electron-donating and electron-withdrawing moieties, with the aim to further improve the balance of the carrier transportation in blue PHOLEDs. It is interesting that small-molecule bipolar host materials based on the sky-blue phosphor emitter FIrpic [iridium(III) bis(4,6-difluorophenylpyridinato-N,C2′)picolinate] exhibiting very high external quantum efficiency (EQE) of 20–30% reported so far all possess very simple chemical structures.4 Notably, these highest efficiency bipolar host materials were obtained by multi-step synthetic procedures and inevitably involving Pd- or Cu-catalyzed C–N coupling reactions, particularly when employing most widely used carbazole as the hole-transporting moiety, which are not cost-effective for large production.7,8 In our recent work, we developed a very simple versatile approach to construct a series of carbazole/pyridine hybrid bipolar host materials through one-step catalyst free C–N coupling reaction.5 Satisfactory efficiencies (max. 42.1 cd A−1, 38.9 lm W−1) for the sky-blue PHOLEDs were achieved based on 9,9′,9′′-(pyridine-2,4,6-triyl)tris(9H-carbazole) (2,4,6-CzPy) host and FIrpic dopant.
However, only the single pyridine ring in the carbazole/pyridine hybrid bipolar host materials may not act well enough as the electron-transporting moiety,9,10 while implantation of more electron-transporting moieties leads to more complicated synthetic steps, and/or extending the π-conjugation system of the whole molecules which unavoidably decrease the triplet energy of the host materials.6 Very recently, carboline, in which one C atom of carbazole is replaced by a N atom, which can improve the electron-transporting properties of host materials have been substantially demonstrated.10–13 Moreover, it is reported by Lee et al. that among the three known isomers (α-, β-, γ-) of carboline, α-carboline exhibited the best device efficiencies.14 Therefore, in this article, we have designed and synthesized two α-carboline/pyridine hybrid bipolar host materials, namely 2,6-CbPy and 2,4,6-CbPy through our previously presented simple one-step catalyst-free aromatic nucleophilic substitution reaction, since 2,6-CzPy and 2,4,6-CzPy displayed the best device efficiencies among various bi-, tri- and tetra-carbazole substituted pyridine.5a By employing the electron-deficient α-carboline to replace electron-donating carbazole in the host materials, it is expected that the PHOLEDs device efficiency can be further improved.
2. Experimental
2.1 General information
All the reagents and materials were received from Energy Chemical and used without further purification, unless otherwise noted. 1H NMR and 13C NMR spectra were measured on a MECUYR-VX300 spectrometer. Elemental analysis of carbon, hydrogen and nitrogen was performed on a Vario EL III microanalyzer. Mass spectra were recorded on a Thermo ISQ mass spectrometer using an electron impact (EI) ionization technique. UV-vis absorption spectra were recorded on a Shimadzu UV-2500 recording spectrophotometer. PL spectra were recorded on a Hitachi F-4500 fluorescence spectrophotometer. Differential scanning calorimetry (DSC) was performed on a NETZSCH DSC 200 PC unit at a heating rate of 10 °C min−1 from −40 to 300 °C under argon. The glass transition temperature (Tg) was determined from the second heating scan. Thermogravimetric analysis (TGA) was undertaken with a NETZSCH STA 449C instrument. The thermal stability of the samples under a nitrogen atmosphere was determined by measuring their weight loss while heating at a rate of 20 °C min−1 from 25 to 600 °C. Cyclic voltammetry (CV) was measured in nitrogen-purged dichloromethane for oxidation scan by using a CHI voltammetric analyzer. Tetrabutylammonium hexafluorophosphate (TBAPF6) (0.1 M) was used as the supporting electrolyte. The conventional three-electrode configuration consists of a platinum working electrode, a platinum wire auxiliary electrode, and an Ag/AgCl wire pseudo-reference electrode with ferrocenium–ferrocene (Fc+/Fc) as the internal standard. Cyclic voltammograms were obtained at scan rate of 100 mV s−1. Formal potentials are calculated as the average of cyclic voltammetric anodic and cathodic peaks. The onset potential was determined from the intersection of two tangents drawn at the rising and background current of the cyclic voltammogram.
2.2 Device fabrication and measurements
The hole-injecting material MoO3, hole-transporting material NPB (1,4-bis(1-naphthylphenylamino)-biphenyl), exciton-blocking material TCTA (tris(4-(9-H-carbazol-9-yl)phenyl)amine) and electron-transporting material TmPyPb (1,3,5-tri(m-pyrid-3-ylphenyl)benzene) were purchased from Luminescence Technology Corp. Commercial indium tin oxide (ITO) coated glass with sheet resistance of 10 Ω per square was used as the starting substrates. Before device fabrication, the ITO glass substrates were pre-cleaned carefully and treated by UV/O3 for 2 min. Then the sample was transferred to the deposition system. 5 nm of MoO3 was firstly deposited to ITO substrate, followed by 60 nm NPB, 5 nm TCTA, 10 nm emitting layer and 35 nm TmPyPB. Finally, a cathode composed of 2 nm CsCO3 and 100 nm aluminum was sequentially deposited onto the substrates in the vacuum of 10−6 Torr to construct the device. The current density–voltage–luminance (J–V–L) curves of EL devices were measured with a Keithley 2400 Source meter and a Keithley 2000 Source multimeter equipped with a calibrated silicon photodiode. The EL spectra were measured by JY SPEX CCD3000 spectrometer. All measurements were carried out at room temperature under ambient conditions.
2.3 Synthesis
2,6-Bis(9-H-pyrido[2,3-b]indol-9-yl)pyridine (2,6-CbPy). A mixture of pyridoindole (0.64 g, 3.8 mmol), 2,6-difluoropyridine (0.20 g, 1.7 mmol) and K2CO3 (0.94 g, 6.8 mmol) in dimethyl sulfoxide (DMSO) (10 mL) was stirred at 150 °C for 12 h under an argon atmosphere. After cooling to room temperature, the mixture was poured into water, filtered and then purified by column chromatography over silica gel using 1
:
3 CH2Cl2/petroleum ether (V/V) as the eluent to afford a white solid (0.56 g, 81%). Mp 250 °C; 1H NMR (400 MHz, CDCl3, δ): 8.59 (d, J = 3.7 Hz, 2H), 8.46–8.41 (m, 4H), 8.32 (d, J = 7.7 Hz, 2H), 8.24–8.20 (m, 1H), 8.10 (d, 2H), 7.43–7.32 (m, 6H); 13C NMR (100 MHz, CDCl3, δ): 148.41, 142.61, 130.88, 130.12, 124.38, 122.92, 119.68, 119.25, 116.91; MS EI (m/z): 411.10 [M+]; anal. calcd for C27H17N5: C 78.81, H 4.16, N 17.02; found: C 78.43, H 4.09, N 16.93.
9,9′,9′′-(Pyridine-2,4,6-triyl)tris(9-H-pyrido[2,3-b]indole) (2,4,6-CbPy). The synthetic procedure was the same as 2,6-CbPy except for using 2,4,6-trifluoropyridine (0.14 g, 1.03 mmol) to replace 2,6-difluoropyridine. Purified by column chromatography over silica gel using 1
:
1 CH2Cl2/petroleum ether (V/V), the product is a white solid (0.42 g, 70%). Mp 307 °C; 1H NMR (CDCl3, 400 MHz, δ): 8.95 (s, 2H), 8.65 (d, J = 8.4 Hz, 2H), 8.59–8.57 (m, 3H), 8.46–8.40 (m, 4H), 8.18–8.11 (m, 3H), 7.64 (t, J = 7.2 Hz, 1H), 7.49–7.31 (m, 8H); 13C NMR (100 MHz, CDCl3, δ): 149.05, 148.63, 130.93, 130.70, 130.13, 130.08, 124.46, 123.59, 122.93, 119.78, 117.34, 115.76, 114.66; MS EI (m/z): 577.18 [M+]; anal. calcd for C38H23N7: C 79.01, H 4.01, N 16.97; found: C 78.90, H 4.04, N 16.80.
3. Results and discussion
3.1 Synthesis and characterization
Scheme 1 illustrates the synthetic routes for the two new host materials of 2,6-CbPy and 2,4,6-CbPy. Both compounds can be synthesized by a one-step catalyst free C–N coupling reaction, by using pyridoindole and cheap 2,6-difluoropyridine or 2,4,6-trifluoropyridine as the starting materials. Satisfactory yields of 81 and 70% were achieved for 2,6-CbPy and 2,4,6-CbPy, respectively. The slightly lower yields than the carbazole containing 2,6-CzPy and 2,4,6-CzPy was attributed to the use of electron-withdrawing α-carboline as the nitrogen nucleophiles instead of our previously reported electron-donating carbazole that reduced the H atom activity of at the 9-N position.2b,5 Nevertheless, the synthetic methodology in this work is still superior to general procedures for metal-catalyzed C–N coupling reaction of other carboline derivatives.10–13 Both compounds were fully characterized by 1H NMR, 13C NMR, mass spectrometry and elemental analysis. The thermal properties of 2,6-CbPy and 2,4,6-CbPy were investigated by thermogravimetric analysis (TGA) and differential scanning calorimetry (DSC). As shown in Fig. 1, 2,6-CbPy and 2,4,6-CbPy decomposed at 338 and 441 °C respectively (Td, corresponding to 5% weight loss in the TGA). And their related glass transition temperatures (Tg) appeared at 75 and 117 °C respectively (inset of Fig. 1). The Tg values of 2,6-CbPy and 2,4,6-CbPy are similar to their carbazole analogues (75 and 124 °C, Table 1), which indicated that small chemical modification from C atom in carbazole to N atom in α-carboline can not significantly alter the thermal properties of the compounds.
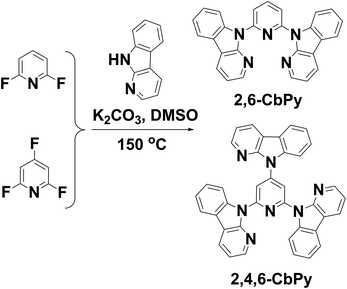 |
| Scheme 1 Synthetic procedure for 2,6-CbPy and 2,4,6-CbPy. | |
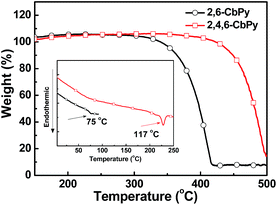 |
| Fig. 1 TGA and DSC (inset) curves of 2,6-CbPy and 2,4,6-CbPy. | |
Table 1 Thermal and optical properties of 2,6-CbPy and 2,4,6-CbPy
Compounds |
Tg/Tm/Td [°C] |
λabsa [nm] |
λPL,maxa [nm] |
λPL,maxb [nm] |
Egc [eV] |
HOMO/LUMOd [eV] |
ETe [eV] |
Measured in CHCl3 solution at room temperature. Measured at film state. Energy gaps (Eg) calculated from the onsets of UV-vis spectra. HOMO deduced from CV and LUMO was calculated from Eg and HOMO (calculated results in parentheses). Triplet energy calculated from phosphorescence spectra. Data from ref. 5a. |
2,6-CbPy |
75/250/338 |
289, 298, 314 |
377 |
386 |
3.54 |
−5.70 (−5.43)/−2.16 (−1.07) |
2.88 |
2,4,6-CbPy |
117/307/421 |
286, 293, 323 |
374 |
390 |
3.53 |
−5.81 (−5.53)/−2.31 (−1.28) |
2.87 |
2,6-CzPyf |
75/222/329 |
289, 337 |
377 |
379 |
3.54 |
−5.63 (−5.43)/−2.08 (−1.07) |
3.00 |
2,4,6-CzPyf |
124/312/412 |
288, 334 |
387 |
376 |
3.46 |
−5.67 (−5.51)/−2.21 (−1.27) |
2.99 |
3.2 Photophysical properties
The absorption and photoluminescence (PL) spectra of the two compounds in CHCl3 solution were shown in Fig. 2. All the key data were summarized in Table 1. The strong absorption peak at ∼290 nm might originate from the n–π* transitions of the pyridoindole moiety, which was similar to their carbazole analogues. And the weak absorption bands over 310 nm could be assigned to π–π* transitions of the backbone due to the twisted structure. From the onsets of the absorption spectra, the energy gaps (Eg) were deduced to be 3.54 and 3.53 eV for 2,6-CbPy and 2,4,6-CbPy, respectively. The carboline based two new compounds displayed little difference on the photoluminescence behavior compared to carbazole containing 2,6-CzPy and 2,4,6-CzPy. 2,6-CbPy and 2,4,6-CbPy exhibited ultraviolet emission with peaks at 377 and 374 nm in CHCl3 solution and 386 and 390 nm at film state, respectively. The triplet energies (ET) calculated from the highest-energy vibronic sub-bands of the low temperature (77 K) phosphorescence spectra were determined to be ca. 2.88 eV for 2,6-CbPy and 2.87 eV for 2,4,6-CbPy, which was slightly lower than ∼3.0 eV of their carbazole counterparts. However, the high triplet energies of two compounds qualify for their application as hosts of blue phosphorescent FIrpic (ET = 2.65 eV).3
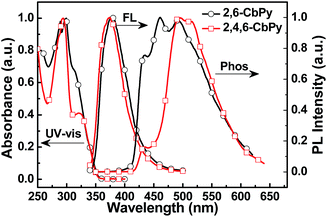 |
| Fig. 2 UV-vis absorption and fluorescence (FL) spectra of 2,6-CbPy and 2,4,6-CbPy in CHCl3 at room temperature, and their phosphorescence (Phos) spectra in neat film at 77 K. | |
3.3 Electrochemical properties and DFT calculations
The electrochemical behavior of the two compounds was examined by cyclic voltammetry (CV) in CH2Cl2 solution (Fig. 3). They exhibited quasi-reversible/irreversible oxidation behaviors whereas non-detectable reduction processes. Calculated from the onsets of the oxidation potentials, their HOMO energy levels were −5.70 eV for 2,6-CbPy and −5.81 eV for 2,4,6-CbPy (relative to vacuum energy level). The introduction of electron-withdrawing α-carboline lowers the HOMO levels compared to carbazole containing 2,6-CzPy and 2,4,6-CzPy (Table 1). Deduced from the HOMO energy levels and energy gaps (Eg), their LUMO energy levels were −2.16 and −2.31 eV for 2,6-CbPy and 2,4,6-CbPy, respectively.
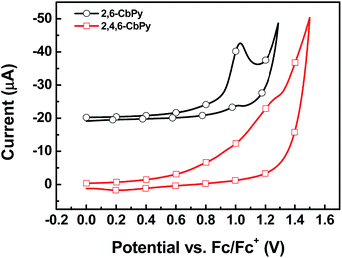 |
| Fig. 3 Cyclic voltammograms in CH2Cl2 for oxidation scan. | |
The geometrical and electronic properties of the two compounds were studied by density functional theory (DFT) calculations using B3LYP hybrid functional at the basis set of 6-31G(d). As shown in Fig. 4, the LUMO of 2,6-CbPy and 2,4,6-CbPy were mainly located in pyridine, with partially distribution on all the corresponding electron-withdrawing carboline. And the HOMO were distributed nearly on the same parts of peripheral two carboline and the central pyridine ring for both compounds. The excess of electron-withdrawing α-carboline moiety at the 4-position of 2,4,6-CbPy lowers both the HOMO and LUMO levels compared with 2,6-CbPy, the theoretical trend was in good agreement with experimental results for both compounds. Furthermore, the HOMO energy of the two new electron-deficient α-carboline based compounds were lower than their carbazole counterparts respectively.
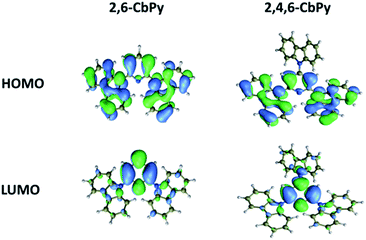 |
| Fig. 4 Molecular orbital distributions of 2,6-CbPy and 2,4,6-CbPy. | |
3.4 Electroluminescent device
Before blue PHOLEDs were fabricated, the charge transport properties of the new carboline/pyridine hybrid compounds were initially investigated. 2,4,6-CbPy was selected as the representatives to fabricate the single-carrier devices with the following configuration of ITO/MoO3 (8 nm)/2,4,6-CbPy (80 nm)/MoO3 (8 nm)/Al (120 nm) for hole-only device; and Al (120 nm)/Cs2CO3 (2 nm)/2,4,6-CbPy (80 nm)/Cs2CO3 (2 nm)/Al (120 nm) for electron-only device. Besides, the relevant control devices were also constructed based on the carbazole analogue 2,4,6-CzPy under the same condition. In these single-carrier devices, MoO3 and Cs2CO3 served as the hole- and electron-injecting materials as well as preventing electron and hole injection from the cathode and anode, respectively. The current density versus voltage curves of these single-carrier devices were depicted in Fig. 5. As shown, the electron current of 2,4,6-CbPy device was obviously higher than the control 2,4,6-CzPy device under the same condition in the range of the PHOLED device operating voltages (solid line, below 12 V); while the hole current curves of the two devices displayed similar trend and almost coincided with each other. It is noteworthy that besides maintaining the good hole-transport properties, the replacement of carbazole by α-carboline in the bipolar host materials significantly improved the electron transporting abilities.
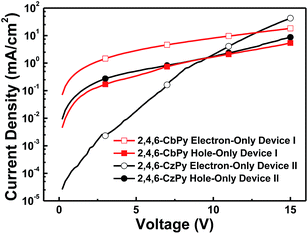 |
| Fig. 5 Current density–voltage curves of the hole- and electron-only devices for 2,6-CbPy and 2,4,6-CzPy. | |
Subsequently, sky-blue PHOLEDs with the following configuration of ITO/MoO3 (5 nm)/NPB (60 nm)/TCTA (5 nm)/2,6-CbPy (device A) or 2,4,6-CbPy (device B):FIrpic (10 nm)/TmPyPB (35 nm)/Cs2CO3 (2 nm)/Al were fabricated to evaluate the EL performance of the high triplet new compounds, where NPB was used as the hole transporting material; TCTA was used to function as hole-transport and to confine triplet excitons within the emitting layer; TmPyPB was acted as the electron transporting as well as hole-blocking material; FIrpic was used as the blue-emitting material with optimized doping concentration of 13 wt%; MoO3 and Cs2CO3 served as hole- and electron injecting materials, respectively. The energy levels of the materials involved in the devices were depicted in Fig. 6.15 The luminance–current density–voltage characteristics, current efficiency (CE)/power efficiency (PE) versus luminance and electroluminescent (EL) spectra of the two devices were shown in Fig. 7, and all the key device data were summarized in Table 2.
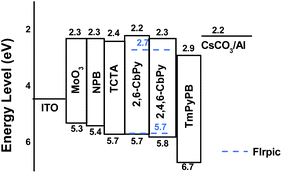 |
| Fig. 6 The energy levels of the materials used in the device. | |
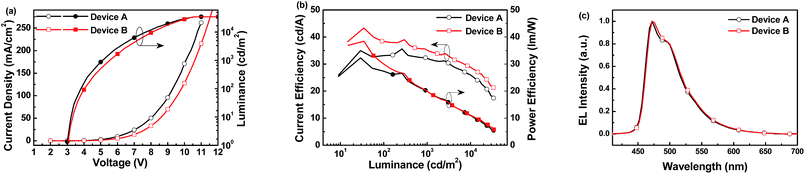 |
| Fig. 7 (a) Current density–voltage–luminance of the two devices. (b) Current efficiency/power efficiency versus luminance of the two devices. (c) EL spectra recorded at 8 V of devices. | |
Table 2 Electroluminescent properties of the blue phosphorescent devices A and B
Device |
Host material |
Vona [V] |
ηcb [cd A−1] |
ηpc [lm W−1] |
CIE (x, y) at 8 V |
Turn-on voltage. Maximum current efficiency and at 1000 cd m−2. Maximum power efficiency and at 1000 cd m−2. Data from ref. 5a. |
A |
2,6-CbPy |
3.0 |
35.9/31.9 |
33.0/19.3 |
(0.16, 0.33) |
B |
2,4,6-CbPy |
3.0 |
44.1/34.6 |
38.5/19.4 |
(0.16, 0.35) |
Cd |
2,4,6-CzPy |
2.9 |
42.1/37.5 |
38.9/23.3 |
(0.16, 0.35) |
The two devices exhibited typical sky-blue emission from FIrpic with the Commission Internationale de l'Eclairage (CIE) coordinates of (0.16, 0.33) for device A and (0.16, 0.35) for device B (Fig. 7c), indicating that the triplet excitons were well controlled on the FIrpic emitter. Both devices displayed turn-on voltages of 3 V and maximum luminance of 34
775 cd m−2 and 34
676 cd m−2, respectively. A maximum current efficiency of 35.9 cd A−1 and power efficiency of 33.0 lm W−1 was achieved in device A based on 2,6-CbPy host. At high brightness of 1000 cd m−2 (3.7 mA cm−2 at 5.2 V), device A showed CE of 31.9 cd A−1 and PE of 19.3 lm W−1. On the other hand, device B based on 2,4,6-CbPy exhibited better performance than device A, with the maximum current efficiency of 44.1 cd A−1 and power efficiency of 38.5 lm W−1 at 0.2 mA cm−2. At high brightness of 100 and 1000 cd m−2, the current efficiency was still as high as 38.2 and 34.6 cd A−1, respectively. The higher efficiencies of device B might be attributed to more α-carboline moieties of 2,4,6-CbPy. The device efficiency of the newly developed 2,4,6-CbPy host is higher than 32.7 cd A−1 (at 100 cd m−2)16 or 19.5 lm W−1 (maximum value)17 for 1,3-di(9H-carbazol-9-yl)benzene (mCP) host with similar device structure.
It is noticeable that the device efficiencies based on carboline containing 2,4,6-CbPy was slightly higher than the carbazole analogue of 2,4,6-CzPy (maximum efficiency of 42.1 cd A−1) under the identical device configuration, which was also the optimized device configuration for 2,4,6-CzPy.5a As discussed in the hole- and electron-only device, the hole-transport properties of the 2,4,6-CbPy and 2,4,6-CzPy was almost the same, while the electron-mobility of carboline containing 2,4,6-CbPy was significantly higher than the carbazole based 2,4,6-CzPy with the device operating voltages. Therefore, we believe the device performance of 2,4,6-CbPy host can be further improved by optimizing the device structure to obtain new balance for hole and electron current in the devices, such as increasing the electron-transport TmPyPB thickness to reduce electron-current, or reducing the hole-transport NPB layer thickness or employing higher hole mobility of TAPC (1,1-bis[(di-4-tolylamino)phenyl]cyclohexane) (∼10−2 cm2 V−1 s−1) as the hole-transporting material to increase hole-current.2a
4. Conclusion
In conclusion, two α-carboline/pyridine hybrid small-molecule host materials have been designed and synthesized through a low-cost catalyst-free C–N coupling reaction. The replacement of carbazole by α-carboline in the new bipolar host materials have resulted in comparable hole-transport property whereas significantly improved electron-transporting ability, simultaneously without sacrificing the triplet energies. The carboline containing 2,4,6-CbPy host exhibited slightly higher maximum current efficiency of 44.1 cd A−1 than 42.1 cd A−1 of the carbazole based 2,4,6-CzPy at the same device condition. It is expected that device performance of 2,4,6-CbPy hosted device can be further improved under the more optimized device configuration.
Acknowledgements
The authors thank the financial support from the NSFC (61474062) and NSF of Jiangshu Province (BK20130919). We also thank Prof. Chuluo Yang and Prof. Jingui Qin for the measurement of the physical properties of the new materials.
References
-
(a) S. R. Forrest, M. A. Baldo, D. F. O. Brien, Y. You, A. Shoustikov, S. Sibley and M. E. Thompson, Nature, 1998, 395, 151 CrossRef;
(b) M. A. Baldo, M. E. Thompson and S. R. Forrest, Nature, 2000, 403, 750 CrossRef CAS PubMed;
(c) C. Han, Z. Zhang, H. Xu, J. Li, G. Xie, R. Chen, Y. Zhao and W. Huang, Angew. Chem., Int. Ed., 2012, 51, 10104 CrossRef CAS PubMed;
(d) C. Fan and C. Yang, Chem. Soc. Rev., 2014, 43, 6439 RSC;
(e) D. Yu, F. Zhao, C. Han, H. Xu, J. Li, Z. Zhang, Z. Deng, D. Ma and P. Yan, Adv. Mater., 2012, 24, 509 CrossRef CAS PubMed.
-
(a) Y. Tao, C. Yang and J. Qin, Chem. Soc. Rev., 2011, 40, 2943 RSC;
(b) Y. Tao, Q. Wang, C. Yang, Q. Wang, Z. Zhang, T. Zou, J. Qin and D. Ma, Angew. Chem., Int. Ed., 2008, 47, 8104 CrossRef CAS PubMed;
(c) Y. Tao, Q. Wang, C. Yang, C. Zhong, J. Qin and D. Ma, Adv. Funct. Mater., 2010, 20, 2923 CrossRef CAS PubMed;
(d) C. Fan, L. Zhu, T. Liu, B. Jiang, D. Ma, J. Qin and C. Yang, Angew. Chem., Int. Ed., 2014, 53, 2147 CrossRef CAS PubMed;
(e) B. X. Mi, Z. Q. Gao, Z. J. Liao, W. Huang and C. H. Chen, Sci. China: Chem., 2010, 53, 1679 CrossRef CAS.
-
(a) Y. Seino, H. Sasabe, Y. J. Pu and J. Kido, Adv. Mater., 2014, 26, 1612 CrossRef CAS PubMed;
(b) H. Sasabe and J. Kido, Chem. Mater., 2011, 23, 621 CrossRef CAS;
(c) Z. An, R. Chen, J. Yin, G. Xie, H. Shi, T. Tsuboi and W. Huang, Chem.–Eur. J., 2011, 17, 10871 CrossRef CAS PubMed;
(d) R. Chen, G. Xie, Y. Zhao, S. Zhang, J. Yin, S. Liu and W. Huang, Org. Electron., 2011, 12, 1619 CrossRef CAS PubMed;
(e) H. Sasabe, Y. Pu, K. Nakayama and J. Kido, Chem. Commun., 2009, 6655 RSC;
(f) F. Wang, Y. Tao and W. Huang, Chin. J. Chem., 2015, 73, 9 CAS.
-
(a) J. Jin, W. Zhang, B. Wang, G. Mu, P. Xu, L. Wang, H. Huang, J. Chen and D. Ma, Chem. Mater., 2014, 26, 2388 CrossRef CAS;
(b) W. L. Chil and Y. L. Jun, Adv. Mater., 2013, 25, 5450 CrossRef PubMed;
(c) H. H. Chou and C. H. Cheng, Adv. Mater., 2010, 22, 2468 CrossRef CAS PubMed;
(d) B. Pan, B. Wang, Y. Wang, P. Xu, L. Wang, J. Chen and D. Ma, J. Mater. Chem. C, 2014, 2, 2466 RSC;
(e) Y. Seino, H. Sasabe, Y.-J. Pu and J. Kido, Adv. Mater., 2014, 26, 1612 CrossRef CAS PubMed;
(f) C. W. Lee, J.-K. Kim, S. H. Joo and J. Y. Lee, ACS Appl. Mater. Interfaces, 2013, 5, 2169 CrossRef CAS PubMed.
-
(a) C. Tang, R. Bi, Y. Tao, F. Wang, X. Cao, S. Wang, T. Jiang, C. Zhong, H. Zhang and W. Huang, Chem. Commun., 2015, 51, 1650 RSC;
(b) C. Tang, T. Yang, X. Cao, Y. Tao, F. Wang, C. Zhong, Y. Qian, X. Zhang and W. Huang, Adv. Opt. Mater., 2015, 3, 786 CrossRef CAS PubMed.
-
(a) S.-J. Su, H. Sasabe, T. i. Takeda and J. Kido, Chem. Mater., 2008, 20, 1691 CrossRef CAS;
(b) C. W. Lee and J. Y. Lee, Adv. Mater., 2013, 25, 5450 CrossRef CAS PubMed.
- Y. J. Cho, K. S. Yook and J. Y. Lee, Adv. Mater., 2014, 26, 4050 CrossRef CAS PubMed.
- S. L. Gong, Y.-L. Chang, K. Wu, R. White, Z.-H. Lu, D. Song and C. Yang, Chem. Mater., 2014, 26, 1463 CrossRef CAS.
- Y. H. Son, Y. J. Kim, M. J. Park, H.-Y. Oh, J. S. Park, J. H. Yang, M. C. Suh and J. H. Kwon, J. Mater. Chem. C, 2013, 1, 5008 RSC.
- Y. Tao, J. Xiao, C. Zheng, Z. Zhang, M. Yan, R. Chen, X. Zhou, H. Li, Z. An, Z. Wang, H. Xu and W. Huang, Angew. Chem., Int. Ed., 2013, 52, 10491 CrossRef CAS PubMed.
- J. K. Sun, J. K. Young and H. S. Yong, Chem. Commun., 2013, 49, 6788 RSC.
- C. W. Lee, Y. Im, J.-A. Seo and J. Y. Lee, Chem. Commun., 2013, 49, 9860 RSC.
-
(a) T. Motoyama, H. Sasabe, Y. Seino, J. Takamatsu and J. Kido, Chem. Lett., 2011, 40, 306 CrossRef CAS;
(b) H. Fukagawa, N. Yokoyama, S. Irisa and S. Tokito, Adv. Mater., 2010, 22, 4775 CrossRef CAS PubMed;
(c) C. W. Lee and J. Y. Lee, Chem. Mater., 2014, 26, 1616 CrossRef CAS;
(d) J. W. Yang and J. Y. Lee, Org. Electron., 2015, 22, 74 CrossRef CAS PubMed;
(e) S. Y. Byeon, S. K. Jeon, S.-H. Hwang and J. Y. Lee, Dyes Pigm., 2015, 120, 258 CrossRef CAS PubMed.
- Y. Im and J. Y. Lee, Chem. Commun., 2013, 49, 5948 RSC.
-
(a) Y. Shirota and H. Kageyama, Chem. Rev., 2007, 107, 953 CrossRef CAS PubMed;
(b) D.-H. Lee, Y.-P. Liu, K.-H. Lee, H. Chae and S. M. Cho, Org. Electron., 2010, 11, 427 CrossRef CAS PubMed;
(c) K. Goushi, R. Kwong, J. J. Brown, H. Sasabe and C. Adachi, J. Appl. Phys., 2004, 95, 7798 CrossRef CAS PubMed;
(d) S.-J. Su, E. Gonmori, H. Sasabe and J. Kido, Adv. Mater., 2008, 20, 4189 CAS;
(e) S.-J. Su, Y. Takahashi, T. Chiba, T. Takeda and J. Kido, Adv. Funct. Mater., 2009, 19, 1260 CrossRef CAS PubMed.
- J.-K. Bin, N.-S. Cho and J.-I. Hong, Adv. Mater., 2012, 24, 2911 CrossRef CAS PubMed.
- X. Yang, S. Zhuang, X. Qiao, G. Mu, L. Wang, J. Chen and D. Ma, Opt. Express, 2012, 20, 24412 Search PubMed.
Footnote |
† The two authors contributed equally to this work. |
|
This journal is © The Royal Society of Chemistry 2015 |
Click here to see how this site uses Cookies. View our privacy policy here.