DOI:
10.1039/C5RA14284A
(Paper)
RSC Adv., 2015,
5, 66704-66710
Hydrogenation in supercritical conditions catalyzed by palladium supported on modified activated carbon
Received
20th July 2015
, Accepted 29th July 2015
First published on 30th July 2015
Abstract
Biomass is expected to be a substitute for traditional fossil fuels because it is renewable and environmentally friendly. Bio-oil, as an important utilization of biomass, has a complex composition. Accordingly, it remains a great challenge for oxygenated compounds in bio-oil to be upgraded, especially for furfural. In this research, a highly active catalyst with 1 wt% palladium supported on activated carbon (Pd/AC) was prepared by impregnating Pd species on modified activated carbon, which was applied to furfural catalytic hydrogenation in super critical carbon dioxide (scCO2). The conversion rate (79%) was higher than that obtained in toluene as solvent (60%). A selectivity of furfuralcohol and tetrahydrofurfuryl alcohol of up to 100% was achieved in scCO2 conditions with no byproducts obtained. In contrast, the total selectivity was only 60% using ethanol as solvent. Meanwhile, the reaction conditions in the scCO2 including hydrogen pressure and catalyst amount have been investigated. The Pd/AC catalysts were characterized by XRD, SEM, IR and BET techniques. A green solvent, and high activity and selectivity present real advantages of this method over other methods.
Introduction
Bio-oil is a complex mixture of oxygenated compounds derived from the thermal breakdown of sustainable resources.1,2 Furfural, as one of the numerous oxygenates found in bio-oil, is particularly undesirable as fuel components.3,4 Due to its high reactivity, furfural is required to be catalytically hydrodeoxygenated to improve the bio-oil boiling point range, storage stability, and water solubility.5 Up to now, hydrogenation of furfural to furfuralcohol and other products has been investigated over different noble metal catalysts (e.g., Pt,6 Pd,7 and Ru8). Among these catalysts, the Pd catalyst exhibits higher activity for catalyzing hydrogenation and has shown an interesting behavior in terms of selectivity.7,9 Furthermore, characteristics of the support have been proposed to play an important role in determining the overall reactivity, including acid–base properties, redox properties, and the strength of the metal-support interaction.7 Activated carbon has advantages of high specific surface area and adsorption capacity, which can be considered as the support to enhance the activity of the catalyst used in hydrogenation reaction.10,11 Additionally, the activity of Pd/AC catalyst can be further improved via pore structure and surface modification.12 According to some researchers, the characteristics of the surface of the activated carbon, such as functional groups, influence the overall reaction with organic substances. Various functional groups, such as carboxyl and hydroxyl can be introduced on the surface of ACs after nitric acid treatment, which are beneficial for the dispersion of Pd nanoparticles.13,14 Consequently, in this work, modified activated carbon was used as a support for the loading of Pd by impregnation method.
On the other hand, the development of supercritical fluid technology utilized in the catalytic reaction system has been widely reported in the literature.15,16 Supercritical fluid (SCF) is an excellent solvent because of its special characteristics combined with gas-like viscosity and diffusivity, and liquid-like density and solvating properties. Herein SCFs enable to decrease mass transfer resistance and enhance transfer rate. Supercritical water (SCW), supercritical ethanol (SCE), and scCO2 are usually considered as new reaction media. In previous work, excellent results of catalytic hydrogenation of bio-oil in supercritical condition were obtained.17–19 However, conventional solvents are always non- recyclable and side reactions may occur in supercritical reaction process. Within this research, the scCO2 applied in the upgrading of bio-oil was investigated. Firstly, health and safety are the obvious benefits of scCO2 technique. Secondly, because of the instability of furfural, low temperature of scCO2 is responsible for avoiding the occurrence of adverse reactions, decreasing the formation of carbon deposition and thus preventing blockage of reactor. Another major benefit of using scCO2 in the upgrading of bio-oil refers to the superior solubility between scCO2 and hydrogen, which indicates mass transfer resistance can be lessened in the process of hydrogenation. As a result, higher conversion of furfural could be acquired. Moreover, scCO2 offers some potential advantages: high diffusivity, good mass and thermal transport properties and so on.20
In the present paper, supercritical fluid technology combined with catalysis has been used in the furfural catalytic hydrogenation. We present the results of catalytic hydrogenation on Pd/AC catalyst under supercritical conditions. Additionally, different pretreatments towards the activated carbon support have been discussed and the performance of the hydrogenation in scCO2 has been compared with that in the conventional organic solvent of ethanol and toluene.
Experimental
1. Preparation of catalyst
The activated carbon support was prepared by the following procedure. The base AC (coconut shell charcoal, purchased from Aladdin Industrial Co.) was pretreated with 200 mL of 3 mol L−1 nitric acid solution at 80 °C for 5 h with continuous magnetic stirring. After carboxyl and hydroxyl groups formed, the AC was washed thoroughly with deionized water until the neutral pH was reached, followed by drying at 110 °C for 24 h. The support was denoted as H-AC after oxidation process of nitric acid. And then the pretreated AC was further calcined at 300 °C for 5 h in air and then denoted as M-AC.
The modified AC was impregnated with H2PdCl4 solutions and stirred for 30 min at 50 °C by ultrasonic oscillation. After that, the sample was dried for 10 h at 110 °C. Then the sample was precipitated with NaOH solution added until the pH value reached to 9. At this time, calculated amount of hydrazine hydrate solution was dropwise added, and the solution was stirred for 2 h at 60 °C. Ultimately, it was dried at 110 °C for 8 h. The obtained catalysts were denoted as 1% Pd/H-AC and 1% Pd/M-AC.
2. Catalyzing hydrogenation reactions in supercritical conditions
The experiment of furfural hydrogenation was carried out in a 50 mL stainless steel autoclave equipped with an electrical heating jacket and a magnetic stirrer (Fig. 1). 0.1 g 1% Pd/AC and 0.5 mL furfural (0.006 mol) were introduced into the autoclave. The reactor was purged with H2 five times to remove air from the setup, and then filled with hydrogen at 3.0 MPa. Gaseous CO2 could be transformed into liquid CO2 by cryostat circulator. Afterwards, liquid CO2 was compressed to 8.0 MPa using a piston metering pump. The reactor was then heated to a specific temperature of 130 °C within set time for hydrogenation. After reaction for 5 h, the liquid products were analyzed by GC-MS and GC (equipped with HP-5 capillary column and flame ionization detector (FID)). The quantitative analysis was used known standards and an internal normalization method.
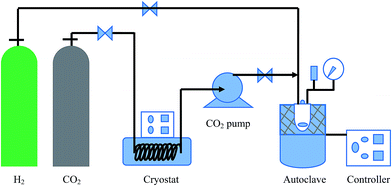 |
| Fig. 1 Photograph of stainless steel autoclave used in furfural hydrogenation reaction. | |
For comparison, the influence of different solvents such as ethanol and toluene for the catalytic hydrogenation was investigated with the amount of 0 mL, 1 mL, 2 mL, 3 mL, 4 mL, respectively, during the condition of hydrogen at 3.0 MPa and temperature of 130 °C for 5 h. The conversion and selectivity of furfural on the measurements was calculated as follows:
|
FAL conversion X [%] = [m(FAL,in) − m(FAL,out)]/m(FAL,in) × 100%
| (1) |
|
FAL selectivity S [%] = m(FAL)/m(FAL,in)/X(FAL) × 100%
| (2) |
3. Catalysts characterization
Structural characterizations of the samples were performed using X-ray diffraction (XRD). The XRD patterns were recorded on a Rigaku D/max-IIIB instrument using CuKα radiation (40 kV and 40 mA). The phase analysis was determined from XRD patterns using the MDI-JADE-5 program. The Fourier infrared (IR) spectrum was observed on a model of VECTOR-22 measurements. Nitrogen sorption isotherms were obtained on a OMNIISORP 100CX instrument at liquid-nitrogen temperature (77 K). All samples were degassed at 110 °C prior to measurements. Specific surface area was acquired using the Brunauer–Emmett–Teller (BET) method based on adsorption data. Pore size distribution (PSD) was evaluated by the Barrett–Joyner–Halenda (BJH) method from the desorption branch of the isotherm. The micro pore volume (Vmicro) was obtained from the t-plot method. The morphologies of the supports were detected by scanning electron microscopy (SEM) on a JEM-T20 instrument.
Results and discussion
1. Impact of CO2 solvent for furfural hydrogenation
The catalytic performances of palladium supported on different supports in scCO2 condition are listed in Table 1. Pd supported on M-AC samples exhibits higher activities for furfural hydrogenation than that supported on H-AC samples or base ACs. The conversion of furfural reaches 79% for 1% Pd/M-AC catalyst. For 1% Pd/H-AC catalyst, the conversion of furfural is only about 47%, which is even lower than that of 1% Pd/AC catalyst (conversion of 66%). It is interesting to be found that the selectivity of furfural and tetrahydrofurfuryl alcohol is distinct over different catalysts. The total selectivity for furfuralcohol and tetrahydrofurfuryl alcohol with 1% Pd/M-AC as catalyst is up to 100%.
Table 1 Furfural hydrogenation in scCO2 condition over different catalystsa
Catalyst |
Conversion (%) |
Selectivity (%) |
Furfuralcohol |
Tetrahydrofurfuryl alcohol |
Reaction conditions: catalyst = 0.1 g; P CO2 = 8 MPa; P H2 = 3 MPa; furfural = 0.5 mL; temperature = 130 °C; time = 5 h. |
1% Pd/AC |
66 |
79 |
21 |
1% Pd/H-AC |
47 |
64 |
34 |
1% Pd/M-AC |
79 |
71 |
29 |
The performances of furfural hydrogenation within or without scCO2 are shown in Table 2. In the absence of catalysts, during solventless condition (P CO2 = 0 MPa), the conversion of furfural is only 10%. However, it increases to 34% by using scCO2 as the reaction medium. Both results show that the furfural hydrogenation can happen even without the help of catalyst. We subsequently tested the activity of 1% Pd/M-AC catalyst under the same conditions and found that the conversion of furfural is raised up to 79% in scCO2 condition, while it is only 48% in solventless condition. It is clearly that the conversion of furfural over 1% Pd/M-AC catalyst can be improved with the introduction of scCO2. Reduction of mass-transfer limitations and a higher solubility of hydrogen in scCO2 are responsible for the high conversion of furfural in SCF.21,22 Additionally, in scCO2 condition, the liquid phase (furfural) disappears and the reaction system contains two phases of CO2-riched fluid phase (CO2, H2, dissolved furfural) and solid phase (catalyst).20
Table 2 Furfural hydrogenation in scCO2 condition and solventless conditiona
Reaction conditions |
Catalyst amount (g) |
Conversion (%) |
Selectivity (%) |
Furfuralcohol |
Tetrahydrofurfuryl alcohol |
Reaction conditions: scCO2 condition (P CO2 = 8 MPa); solventless condition (P CO2 = 0 MPa); P H2 = 3 MPa; furfural = 0.5 mL; temperature = 130 °C; time = 5 h. |
scCO2 condition |
0.1 |
79 |
71 |
29 |
Solventless condition |
0.1 |
48 |
87 |
12 |
scCO2 condition |
0.0 |
34 |
81 |
19 |
Furthermore, in comparison of conventional organic solvents, CO2 is non-toxic, inexpensive, non-flammable, and recyclable.23 The combination of scCO2 and catalysts would be a green chemistry reaction system. No organic solvents are used and separation of the CO2 solvent after utilization is very easy.24
2. Impact of reaction conditions for furfural hydrogenation
2.1 Impact of ethanol and toluene solvent for furfural hydrogenation. Effect of ethanol as solvent for the reaction of furfural hydrogenation is summarized in Fig. 2. The conversion of furfural is 48% in the absence of ethanol over 1% Pd/M-AC with selectivity of 87% for furfuralcohol and of 12% for tetrahydrofurfuryl alcohol. The conversion of furfural increases with the amount of added ethanol and reaches 84% after the addition of 4 mL. However, the selectivity of the target products decreases reaching a low selectivity of 43% for furfuralcohol and a low selectivity of 17% for tetrahydrofurfuryl alcohol. This is due to the fact that ethanol does react with furfural producing 2-furaldehyde diethyl acetal and 2-(methoxymethyl) tetrahydrofuran (detected by GC-MS).
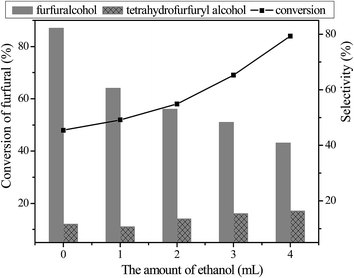 |
| Fig. 2 Effect of ethanol on the furfural hydrogenation. Conditions: furfural = 0.5 mL; temperature = 130 °C; P H2 = 3 MPa; catalyst = 0.1 g; time = 5 h. | |
The conversion of furfural also increases with the amount of added toluene and reaches 60% after the addition of 4 mL (not shown in Fig. 2). As for organic solvent of toluene in the reaction of furfural hydrogenation, only furfuralcohol and tetrahydrofurfuryl alcohol are detected after the reaction. However, it is difficult to separate the toluene and target product, and also toluene is environmentally harmful.
2.2 Impact of hydrogen pressure for furfural hydrogenation. The effect of hydrogen pressure on the furfural hydrogenation is illustrated in Fig. 3. As can be seen from Fig. 3, the conversion of furfural increases from 33% to 81% with the hydrogen pressure increased from 1 MPa to 4 MPa. However, the selectivity of furfuralcohol has been dropped significantly from 76% to 58%. This can be ascribed to the fact that furfuralcohol has the tendency to be converted into tetrahydrofurfuryl alcohol in high hydrogen pressure.25
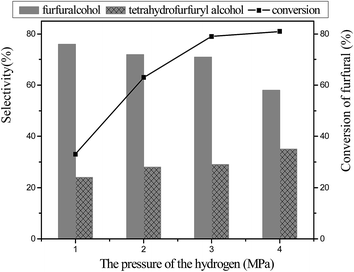 |
| Fig. 3 Effect of hydrogen pressure on the furfural hydrogenation. Conditions: furfural = 0.5 mL; temperature = 130 °C; catalyst = 0.1 g; P CO2 = 8 MPa; time = 5 h. | |
2.3 Impact of catalyst amount for furfural hydrogenation. The influence of the catalyst amount has been observed on the conversion of the furfural hydrogenation under the similar reaction condition in Fig. 4. The conversion of furfural enhances from 34% to 79% in the scCO2 condition, as the amount of catalyst increases from 0 g to 0.1 g. However, the conversion has showed no significant increase when the amount of catalyst continuously increases from 0.1 g to 0.2 g. Furthermore, as the amount of catalyst increases to 0.2 g, the selectivity of furfuralcohol has been decreased from 81% to 46% and that of tetrahydrofurfuryl alcohol could be increased from 19% to 54%. This result suggests that tetrahydrofurfuryl alcohol can be obtained by using excessive amounts of catalyst.
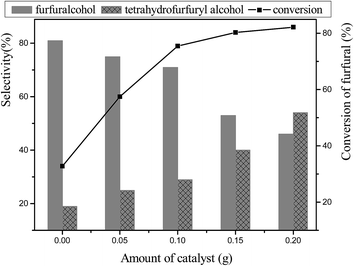 |
| Fig. 4 Effect of catalyst amount on the furfural hydrogenation. Conditions: furfural = 0.5 mL; temperature = 130 °C; P H2 = 3 MPa; P CO2 = 8 MPa; time = 5 h. | |
3. Characterization of the active carbon samples
Fig. 5 demonstrates the shape and behavior of the N2 adsorption isotherms for ACs with different treatment methods. For both H-AC and M-AC samples, N2 adsorption increased at low relative pressure (P/P0). However, the knee of the isotherms is wide, no clear plateau is attained and a certain hysteresis slope can be observed at intermediate and high relative pressures, all these facts indicating the presence of large micropores and mesopores (type IV).26 This is shown by the average diameter values, which are 2.56 nm and 2.59 nm for H-AC and M-AC samples (Table 3).
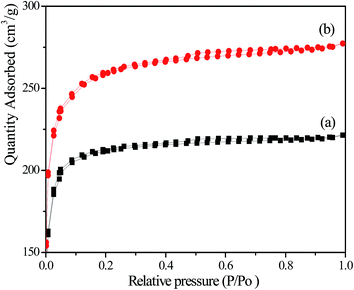 |
| Fig. 5 Nitrogen adsorption/desorption isotherms of samples of support with different treatment methods (a) H-AC and (b) M-AC. | |
Table 3 The BET surface area, pore volume and average pore diameter of AC samples before and after treatment
Sample |
Surface area (m2 g−1) |
Micropore volume (cm3 g−1) |
Average pore diameter (nm) |
AC |
779 |
0.33 |
2.69 |
H-AC |
650 |
0.28 |
2.56 |
M-AC |
804 |
0.31 |
2.59 |
The specific data of surface area, micropore volume and average pore diameter about ACs are given in Table 3. In contrast to AC samples, surface area of H-AC samples decreases from 779 m2 g−1 to 650 m2 g−1 and micropore volume decreases from 0.33 cm3 g−1 to 0.28 cm3 g−1. It is consistent with the literature that parts of activated carbon pores may be collapsed during the modified process of reduction between nitric acid and carbon surface groups.27 However, the surface area of M-AC samples increases significantly to 804 m2 g−1 and micropore volume reaches 0.31 cm3 g−1 compared with H-AC samples.
The SEM images of the Pd/AC catalysts before and after treatment are presented in Fig. 6. Small cavities and a more disordered surface structure can be observed for the 1% Pd/M-AC catalyst. This porous structure offers more contact points with active compounds, which improves the catalytic performance. It can be observed that the treatment with nitric acid and calcination favors the development of the porosity, which is also in good correlation with the results of BET.
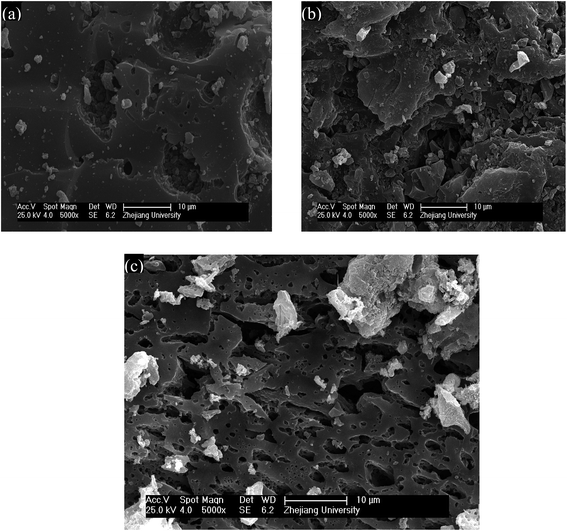 |
| Fig. 6 SEM micrographs of catalysts (a) 1% Pd/AC, (b) 1% Pd/H-AC and (c) 1% Pd/M-AC. | |
4. Functional groups of the modified active carbon
The modified active carbon is favorable to the dispersion of palladium species. Note that the oxygenated functional groups on the surface of active carbon mainly play a key role for the palladium dispersed. The palladium dispersion increases with the increasing amount of surface oxygen groups.28 The FT-IR spectra of modified activated carbon with different treatment methods are shown in Fig. 7. Three main peaks at about 1760 cm−1, 1640 cm−1 and 1380 cm−1 appear in the oxidized samples. The peak at 1760 cm−1 indicates the existence of carbonyl/carboxyl groups. The peak occurring at 1640 cm−1 may be attributable to quinone structure. The band at 1380 cm−1 may be due to the C–O stretching vibrations in alcohols or ether or ester groups. These data are consistent with the literature reported.29,30 It is proposed that oxygenates functional groups, such as carboxyl and hydroxyl groups, have been increased significantly on the surface of activated carbon after the design of nitric acid and high temperature treatment which are helpful for palladium loaded. Accordingly, the activity of Pd/AC catalyst can be further improved via surface modified.
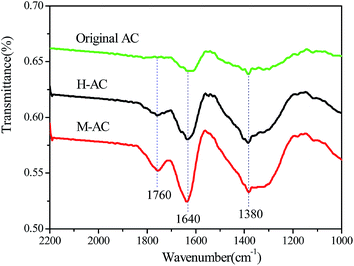 |
| Fig. 7 IR of samples of support with different treatment methods. | |
5. The morphology of the 1% Pd/H-AC and 1% Pd/M-AC catalysts
XRD was used for direct observations of the catalyst with different treatment methods to the AC support. Two patterns, representing of 1% Pd/H-AC and 1% Pd/M-AC catalyst are reported in Fig. 8. Three diffraction peaks for palladium appear at 2θ = 40.0°, 46.6°, 68.0° for the catalyst with different AC supports, corresponding to Pd(111), Pd(200), Pd(220), respectively.31 Moreover, two wide diffraction peaks have been detected at 2θ = 22.6° and 2θ = 26.5° which can be ascribed to the amorphous structure of activated carbon. Characteristic peak of palladium metal has been detected on both catalysts, because palladium species are mainly in the form of a metallic state after the catalysts are reduced. The average particle size calculated by the Scherrer's equation32 is 19.6 nm and 17.0 nm for 1% Pd/H-AC and 1% Pd/M-AC catalysts, respectively, which indicates the palladium species are in good dispersion on the 1% Pd/M-AC catalyst.
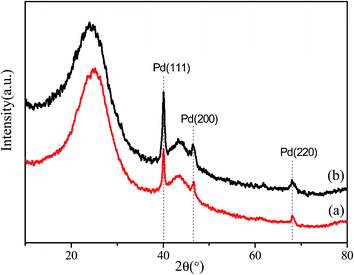 |
| Fig. 8 XRD patterns of (a) 1% Pd/M-AC catalyst and (b) 1% Pd/H-AC catalyst. | |
Conclusions
The results of this work indicate that supercritical CO2 is an excellent solvent for the catalytic hydrogenation of furfural because it is environment friendly, cost effective, and sustainable. Furthermore, it has a low viscosity, which is an advantage in a flow reactor, and the solubility of hydrogen in it is higher than in most solvents. This shows its potential to become a very good alternative to traditional organic solvents for such reactions. On the other hand, 1% Pd/M-AC has been demonstrated to be an efficient catalyst for the catalytic hydrogenation of furfural in scCO2. This work should prove useful for the development of processes for upgrading bio-oils which has a wide range of applications.
Acknowledgements
The support of 973 Program (2013CB228104) of China is greatly appreciated.
References
- D. C. Elliott and T. R. Hart, Catalytic hydroprocessing of chemical models for bio-oil, Energy Fuels, 2009, 23, 631–637 CrossRef CAS.
- S. De, B. Saha and R. Luque, Hydrodeoxygenation processes: advances on catalytic transformations of biomass-derived platform chemicals into hydrocarbon fuels, Bioresour. Technol., 2015, 178, 108–118 CrossRef CAS PubMed.
- S. Sitthisa and D. E. Resasco, Hydrodeoxygenation of furfural over supported metal catalysts: a comparative study of Cu, Pd and Ni, Catal. Lett., 2011, 141, 784–791 CrossRef CAS.
- S. Sitthisa, T. Pham, T. Prasomsri, T. Sooknoi, R. G. Mallinson and D. E. Resasco, Conversion of furfural and 2-methylpentanal on Pd/SiO2 and Pd–Cu/SiO2 catalysts, J. Catal., 2011, 280, 17–27 CrossRef CAS PubMed.
- D. E. Resasco and S. Crossley, Molecular engineering approach in the selection of catalytic strategies for upgrading of biofuels, AIChE J., 2009, 55, 1082–1089 CrossRef CAS PubMed.
- J. Kijenski, P. Winiarek, T. Paryjczak, A. Lewicki and A. Mikolajska, Platinum deposited on monolayer supports in selective hydrogenation of furfural to furfuryl alcohol, Appl. Catal., A, 2002, 233, 171–182 CrossRef CAS.
- X. Xu, Y. Li, Y. T. Gong, P. F. Zhang, H. R. Li and Y. Wang, Synthesis of Palladium nanoparticles supported on mesoporous N-doped carbon and their catalytic ability for biofuel upgrade, J. Am. Chem. Soc., 2012, 134, 16987–16990 CrossRef CAS PubMed.
- Z. Strassberger, M. Mooijman, E. Ruijter, A. H. Alberts, C. de Graaff, R. V. A. Orru and G. Rothenberg, A facile route to ruthenium–carbene complexes and their application in furfural hydrogenation, Appl. Organomet. Chem., 2010, 24, 142–146 CAS.
- E. Diaz, A. F. Mohedano, J. A. Casas, L. Calvo, M. A. Gilarranz and J. J. Rodriguez, Comparison of activated carbon-supported Pd and Rh catalysts for aqueous-phase hydrodechlorination, Appl. Catal., B, 2011, 106, 469–475 CrossRef CAS PubMed.
- J. X. Guo, J. Liang, Y. H. Chu, M. C. Sun, H. Q. Yin and J. J. Li, Desulfurization activity of nickel supported on acid-treated activated carbons, Appl. Catal., A, 2012, 421–422, 142–147 CrossRef CAS PubMed.
- H. Jin, X. M. Wang, Z. R. Gu and J. Polin, Carbon materials from high ash biochar for supercapacitor and improvement of capacitance with HNO3 surface oxidation, J. Power Sources, 2013, 236, 285–292 CrossRef CAS PubMed.
- A. A. Adelodun and Y. M. Jo, Integrated basic treatment of activated carbon for enhanced CO2 selectivity, Appl. Surf. Sci., 2013, 286, 306–313 CrossRef CAS PubMed.
- M. Takaoka, H. Yokokawa and N. Takeda, The effect of treatment of activated carbon by H2O2 or HNO3 on the decomposition of pentachlorobenzene, Appl. Catal., B, 2007, 74, 179–186 CrossRef CAS PubMed.
- P. Z. Cheng and H. S. Teng, Electrochemical responses from surface oxides present on HNO3-treated carbons, Carbon, 2003, 41, 2057–2063 CrossRef CAS.
- Z. Knez, E. Markocic, M. Leitgeb, M. Primozic, M. K. Hrncic and M. Skerget, Industrial applications of supercritical fluids: a review, Energy, 2014, 77, 235–243 CrossRef CAS PubMed.
- H. Durak, Bio-oil production from Glycyrrhiza glabra through supercritical fluid extraction, J. Supercrit. Fluids, 2014, 95, 373–386 CrossRef CAS PubMed.
- J. Peng, P. Chen, H. Lou and X. M. Zheng, Catalytic upgrading of bio-oil by HZSM-5 in sub- and super-critical ethanol, Bioresour. Technol., 2009, 100, 3415–3418 CrossRef CAS PubMed.
- J. Peng, P. Chen, H. Lou and X. M. Zheng, Upgrading of bio-oil over aluminum silicate in supercritical ethanol, Energy Fuels, 2008, 22, 3489–3492 CrossRef CAS.
- W. J. Yu, Y. Tang, L. Y. Mo, P. Chen, H. Lou and X. M. Zheng, One-step hydrogenation–esterification of furfural and acetic acid over bifunctional Pd catalysts for bio-oil upgrading, Bioresour. Technol., 2011, 102, 8241–8246 CrossRef CAS PubMed.
- C. Y. Xi, H. Y. Cheng, J. M. Hao, S. X. Cai and F. Y. Zhao, Hydrogenation of o-chloronitrobenzene to o-chloroaniline over Pd/C in supercritical carbon dioxide, J. Mol. Catal. A: Chem., 2008, 282, 80–84 CrossRef CAS PubMed.
- F. Trachsel, B. Tidona, S. Desportes and P. R. von Rohr, Solid catalyzed hydrogenation in a Si/glass microreactor using supercritical CO2 as the reaction solvent, J. Supercrit. Fluids, 2009, 48, 146–153 CrossRef CAS PubMed.
- J. G. Stevens, R. A. Bourne, M. V. Twigg and M. Poliakoff, Real-time product switching using a twin catalyst system for the hydrogenation of furfural in supercritical CO2, Angew. Chem., Int. Ed., 2010, 49, 8856–8859 CrossRef CAS PubMed.
- F. Yilmaz, A. Mutlu, H. Unver, M. Kurtca and L. Kani, Hydrogenation of olefins catalyzed by Pd(II) complexes containing a perfluoroalkylated S,O-chelating ligand in supercritical CO2 and organic solvents, J. Supercrit. Fluids, 2010, 54, 202–209 CrossRef CAS PubMed.
- F. Zhao, Y. Ikushima and M. Arai, Hydrogenation of nitrobenzene with supported platinum catalysts in supercritical carbon dioxide: effects of pressure, solvent, and metal particle size, J. Catal., 2004, 224, 479–483 CrossRef CAS PubMed.
- K. Kriaa, J.-P. Serin, F. Contamine, P. Cezac and J. Mercadier, 2-Butyne-1,4-diol hydrogenation in supercritical CO2: effect of hydrogen concentration, J. Supercrit. Fluids, 2009, 49, 227–232 CrossRef CAS PubMed.
- N. Passe-Coutrin, S. Altenor, D. Cossement, C. Jean-Marius and S. Gaspard, Comparison of parameters calculated from the BET and Freundlich isotherms obtained by nitrogen adsorption on activated carbons: a new method for calculating the specific surface area, Microporous Mesoporous Mater., 2008, 111, 517–522 CrossRef CAS PubMed.
- P. Singh, M. V. Kulkarni, S. P. Gokhale, S. H. Chikkali and C. V. Kulkarni, Enhancing the hydrogen storage capacity of Pd-functionalized multi-walled carbon nanotubes, Appl. Surf. Sci., 2012, 258, 3405–3409 CrossRef CAS PubMed.
- D. J. Shu, T. J. Park and S. K. Ihm, Effect of surface oxygen groups of carbon supports on the characteristics of Pd/C catalysts, Carbon, 1993, 31, 427–435 CrossRef.
- A. Macias-Garcia, M. A. Diaz-Diez, E. M. Cuerda-Correa, M. Olivares-Marin and J. Ganan-Gomez, Study of the pore size distribution and fractal dimension of HNO3-treated activated carbons, Appl. Surf. Sci., 2006, 252, 5972–5975 CrossRef CAS PubMed.
- C. Saka, BET, TG–DTG, FT-IR, SEM, iodine number analysis and preparation of activated carbon from acorn shell by chemical activation with ZnCl2, J. Anal. Appl. Pyrolysis, 2012, 95, 21–24 CrossRef CAS PubMed.
- Q. C. Zhu, B. X. Shen, H. Ling and R. Gu, Cumene hydroperoxide hydrogenation over Pd/C catalysts, J. Hazard. Mater., 2010, 175, 646–650 CrossRef CAS PubMed.
- Y. Yoshinaga, T. Akita, I. Mikami and T. Okuhara, Hydrogenation of nitrate in water to nitrogen over Pd–Cu supported on active carbon, J. Catal., 2002, 207, 37–45 CrossRef CAS.
|
This journal is © The Royal Society of Chemistry 2015 |