DOI:
10.1039/C5SC01675D
(Edge Article)
Chem. Sci., 2015,
6, 6361-6366
A “turn on” fluorescent probe for heparin and its oversulfated chondroitin sulfate contaminant†
Received
7th May 2015
, Accepted 23rd July 2015
First published on 23rd July 2015
Abstract
Designing “turn on” fluorescent probes for heparin (Hep), a widely used anticoagulant in clinics, is of great importance but remains challenging. By introducing a Hep specific binding peptide AG73 to a typical aggregation induced emission (AIE) fluorogen, tetraphenylethene (TPE), a sensitive and selective “turn on” fluorescent probe named TPE-1 for Hep was developed. TPE-1 was able to detect Hep in a wide pH range of 3–10 without obvious interference from tested anions and biomolecules, especially Hep analogues known as chondroitin sulfate (Chs) and hyaluronic acid (HA). The detection limit of Hep sensing was 3.8 ng mL−1, which was far below the clinically demanded concentration of Hep. The probe was applicable to both unfractionated Hep and low molecular weight Hep, the two main heparin products clinically used. Besides, the fluorescence of Hep bound TPE-1 can be turned off via sequential treatment with heparinases. Importantly, this phenomenon allows us to develop an enzyme assisted strategy for “turn on” sensing of oversulfated chondroitin sulfate (OSCS) with a detection limit of 0.001% (w%), which is the main contaminant in Hep and may cause severe adverse reactions including death.
Introduction
Heparin (Hep) is a highly sulfated glycosaminoglycan (GAG) with the highest negative charge density of any known biomacromolecules.1 It can bind antithrombin with a high affinity, and the binding significantly enhances antithrombin's inhibition activity towards thrombin and other coagulation factors.2 Thus, Hep has been widely used in clinics as both prophylactic and therapeutic agents, especially as an anticoagulant in surgery.3 The Hep therapeutic dosage should be maintained within a range of 2–8 U mL−1 for cardiovascular surgery, and a range of 0.2–1.2 U mL−1 for post-operative and long-term therapy.4 An overdose of Hep could cause many adverse effects such as hemorrhage, thrombocytopenia, hyperkalemia and osteoporosis.5 As a result, the dose of Hep must be closely monitored. In clinical laboratories, the dose is determined by an activated clotting time assay (ACT) or activated partial thromboplastin time assay (aPTT).6 These well-established assays, however, are indirect methods, which are time-consuming, costly, and not sufficiently reliable. Therefore, it is urgent to develop efficient and reliable methods for selective and sensitive detection of Hep.
Considerable efforts have been devoted to the development of Hep detection methods based on various strategies, such as colorimetric assays, capillary electrophoresis, electrochemical methods, surface enhanced Raman spectroscopy, unbiased sensor arrays, etc.7 Recently, particular interests have been focused on the design of small organic molecule based fluorescent probes for sensing various analytes including Hep.8 These probes have shown distinct advantages of being low cost, easy to manipulate and having high performances. For example, a 1,3,5-triphenyl-ethynylbenzene based fluorescent probe for Hep sensing was synthesized by functionalizing it with boronic acid and positively-charged ammonium moieties.9 However, many of the reported methods were based on complicated nano-organic hybrid systems or “turn off” signal responses.9,10 For fluorescence sensing, the “turn on” probes are more reliable than the “turn off” ones, as false results may occur due to the fact that a number of factors other than the target analyte may cause fluorescence quenching.11 In this regard, the development of sensitive and selective “turn on” fluorescent probes for Hep detection is still highly desirable.
Aggregation induced emission (AIE) is a promising strategy for designing “turn on” fluorescent probes, as the AIE fluorogen molecules are almost non-fluorescent in the solution state but become strongly fluorescent after analyte induced aggregation.12 Tetraphenylethene (TPE) is a widely used fluorogen for designing probes based on the AIE mechanism, and it can be facilely functionalized.13 We envisioned that the conjugation of TPE to a Hep specific binding group could afford a “turn on” probe for Hep detection with high sensitivity and selectivity. We noticed that the commonly conjugated ammonium moiety showed a limited specificity for Hep compared to its analogues such as chondroitin sulfate (Chs) and hyaluronic acid (HA). Considering this, a Hep-specific binding peptide, i.e., AG73 (sequence: RKRLQVQLSIRT), was employed to construct the TPE based probe. AG73 is a peptide from the G domain of the laminin α1 chain, which has shown a high binding affinity and specificity towards Hep.14 The probe TPE-1 was rationally designed by conjugating the TPE fluorogen to the AG73 peptide (Scheme 1). Indeed, the designed TPE-1 probe shows an excellent fluorescence “turn on” response to Hep with a detection limit of 3.8 ng mL−1. Moreover, Hep detection with the TPE-1 probe was not interfered with by biological abundant anions, biomolecules, and Hep analogues such as Chs and HA in a wide pH range of 3–10.
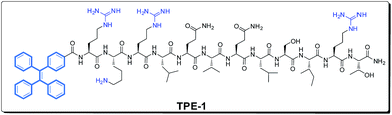 |
| Scheme 1 Structure of the “signal on” probe TPE-1. The probe was constructed by rationally combining the recognition element for Hep (i.e., the AG73 peptide) and the signalling element (i.e., the TPE fluorogen). | |
On the other hand, oversulfated chondroitin sulfate (OSCS) was reported to be the principal contaminant in Hep, which is a non-natural highly sulfated synthetic GAG.15 The presence of OSCS in Hep may lead to severe adverse reactions, such as angiodema, hypotension, swelling of the larynx, and even death.16 Orthogonal methods such as high performance liquid chromatography (HPLC) combined with nuclear magnetic resonance (NMR) and mass spectroscopy are valuable to identify OSCS contaminants in Hep. However, these methods need highly sophisticated instruments and experienced operators. It is thus urgent to develop low cost, easy to operate and highly efficient methods for OSCS detection.17 Assisted with heparinase treatment, here we also propose an approach for OSCS detection using TPE-1 as the probe (Scheme 2). We found that the fluorescence of TPE-1 can be enhanced by Hep binding and quenched by sequential treatment with heparinases. This fluorescence “off–on–off” process was influenced by OSCS, as it is an inhibitor for heparinases. The OSCS contaminants in Hep thus could be quantified by comparing the output fluorescence signals before and after the enzyme treatment (i.e., the Out2/Out1 ratio).
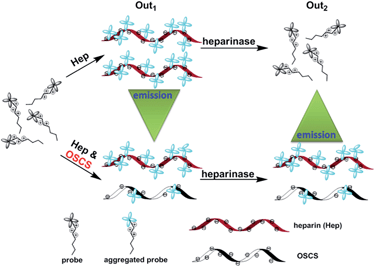 |
| Scheme 2 Fluorescent OSCS detection by TPE-1 assisted with heparinase treatment. The amount of OSCS present in Hep could be determined by the Out2/Out1 ratio. | |
Results and discussion
Design, synthesis and the AIE properties of the TPE-1 probe
As shown in Scheme 1, the molecular structure of TPE-1 consists of two parts, both of which play important roles in Hep sensing. The TPE moiety is a typical AIE fluorogen, which emits strong fluorescence only after aggregation and acts as the signal reporting group in TPE-1. While the AG73 peptide with a sequence of RKRLQVQLSIRT was reported to show a high affinity towards Hep,14a and this moiety is incorporated as the Hep recognition group. TPE-1 was synthesized via solid phase peptide synthesis with an acceptable yield of 33%, and a high purity of 99.7% (see ESI for more details, Fig. S1–S4†). The AIE properties of TPE-1 were then evaluated. As shown in Fig. 1, the fluorescence of free TPE-1 was enhanced by only 114% in 100% HEPES compared with 80% HEPES, which was attributed to its high solubility in water. On the other hand, TPE still retained its excellent AIE properties after bioconjugation, as indicated by the gradual fluorescence increase with the increase of HEPES buffer fractions. Interestingly, there was an obvious inflection point at the 95% HEPES buffer fraction. When the fraction of the HEPES buffer in the mixture was higher than 95%, TPE-1 was sharply turned on and emitted stronger fluorescence. Based on these results, a mixture of a HEPES buffer/DMSO solution with a volume ratio of 95
:
5 was chosen for Hep sensing.
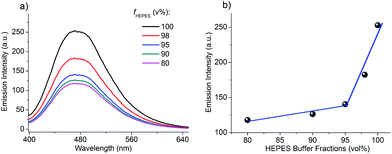 |
| Fig. 1 AIE properties of TPE-1. (a) Fluorescence spectra of TPE-1 (10 μM) in HEPES buffer/DMSO mixed solutions with different HEPES buffer fractions. (b) Plot of the fluorescence intensity at 470 nm versus the HEPES buffer fraction. λex = 340 nm. | |
Sensitive and selective fluorescence “turn on” detection of Hep
As mentioned above, no fluorescence can be observed by the naked eye when TPE-1 is dissolved in a 95% HEPES buffer solution. However, a vivid bluish green fluorescence “turn on” was observed immediately after the addition of Hep to a 10 μM TPE-1 solution (Fig. 2). Fluorescence titration experiments were carried out to investigate the sensing performance of TPE-1 towards Hep. In the absence of Hep, TPE-1 showed a very weak fluorescence. With an increasing amount of added Hep, the fluorescence intensity of the peak at around 470 nm was enhanced gradually. 5.8 μg mL−1 of Hep was needed to saturate the fluorescence enhancement by a factor of about 31 (Fig. 2a). Analysis of the titration data reveals that the emission intensity increased linearly with the increasing concentrations of Hep in a dynamic range of 0.32–5.5 μg mL−1, and the corresponding correlation coefficient R was 0.9995. The calculated detection limit of Hep based on 3σ/s was 35 ng mL−1, where σ was the standard deviation of 10 blank measurements and s was the slope of the emission intensity as a function of the Hep concentration (Fig. 2b). The detection limit can be further lowered by adjusting the amount of TPE-1 used. When the concentration of TPE-1 used was 1 μM, the detection limit of Hep was as low as 3.8 ng mL−1, which was much lower than the clinically demanded concentration of Hep (Fig. S5†). And the corresponding dynamic range was 0.064–0.32 μg mL−1 when the concentration of TPE-1 was 1 μM.
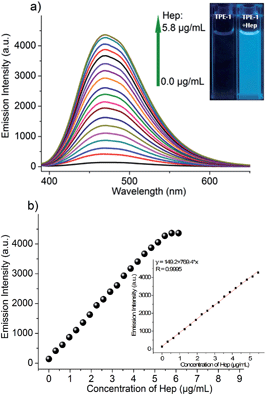 |
| Fig. 2 Hep detection with TPE-1. (a) Fluorescence titration profile of TPE-1 (10 μM) with an increasing amount of Hep in 10 mM, pH 7.4 HEPES/DMSO (95 : 5, v/v). λex = 340 nm. Inset: a photograph showing the fluorescence “turn on” behavior of TPE-1 after addition of 5.8 μg mL−1 Hep. (b) Corresponding calibration curve of Hep. Inset: linear response curve of Hep. | |
We also noticed that there are mainly two forms of Hep used in clinics, and the one tested above was unfractionated Hep (UFH) from hog intestine. Thus we also tested the Hep sensing property of TPE-1 towards the other form of Hep, i.e., low molecular weight Hep (LMWH). The TPE-1 probe also exhibited a good dynamic range and sensing sensitivity towards the two LMWHs tested (dalteparin and enoxaparin), indicating the general capability of TPE-1 for Hep detection (Fig. S6 and S7†).
Hep detection is easily interfered with by its GAG analogues, especially Chs and HA. To obtain good selectivity, highly specific recognition groups are demanded. With the Hep binding peptide AG73 incorporated as the recognition group, TPE-1 showed a high selectivity to Hep over tested anions, Hep analogues and important biomolecules. As illustrated in Fig. 3a and b, only the addition of Hep enhanced the fluorescence intensity by about 31-fold, while the addition of 0.05 mg mL−1 BSA induced a fluorescence enhancement by only about 3-fold. The addition of glucose (10 μM), ATP (10 μM), PO43− (10 μM), DNA (2 μM) and RNA (2 μM) did not produce distinguished fluorescence enhancement. It is worth pointing out that tested Hep analogues including as high as 10 μM of Chs and HA also did not significantly influence the selective detection of Hep when using TPE-1 as the probe (Fig. 3c).
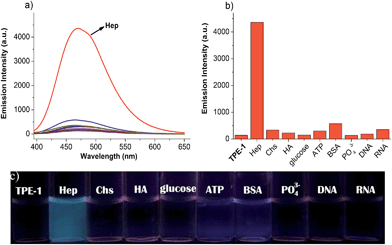 |
| Fig. 3 Selective detection of Hep with TPE-1. (a) Fluorescence spectra of TPE-1 (10 μM) upon addition of various anions and biomolecules in 10 mM, pH 7.4 HEPES/DMSO (95 : 5, v/v). λex = 340 nm. (b) Corresponding fluorescence intensity of TPE-1 at 470 nm. (c) A photograph showing the fluorescence change of TPE-1 (10 μM) upon addition of various anions and biomolecules in 10 mM, pH 7.4 HEPES/DMSO (95 : 5, v/v) under portable UV lamp irradiation. | |
High stability of the TPE-1 probe
During the investigation of using TPE-1 as the “turn on” fluorescent probe for Hep, we found that TPE-1 was very stable. Its stock solution can be stored at room temperature for more than 2 months without obvious decomposition. We then tested the photostability of TPE-1 after binding with Hep. After addition of 5.8 μg mL−1 Hep to 10 μM TPE-1, the fluorescence intensity of the obtained solution was monitored every 20 min. Note, during the monitoring, the solution was under continuous UV lamp irradiation. It was found that there was a negligible decrease in the emission intensity of TPE-1 throughout the 4 hours of UV light irradiation, indicating that TPE-1 showed high photostability after binding to Hep (Fig. 4a).
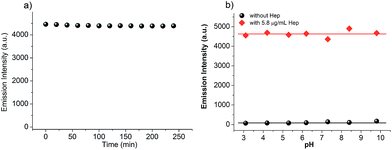 |
| Fig. 4 High stability of TPE-1. (a) Photostability of TPE-1 upon Hep binding under continuous UV light irradiation. (b) Fluorescence emission intensity of TPE-1 (10 μM, black dots) and TPE-1 + 5.8 μg mL−1 of Hep (red squares) over a pH range of 3–10. λex = 340 nm. | |
For a fluorescent probe, its sensing performance under different pHs should also be evaluated. Since the pKa value of the guanidyl group in an arginine residue is about 12.4 and the pKa of the ω-NH3+ group in a lysine residue is about 10.5, we envisioned that the guanidyl group in arginine residues and ω-NH3+ group in lysine residues of the peptide AG73 would remain positively charged within a wide pH range lower than 10. Accordingly, the electrostatic interactions between the positively-charged peptide in TPE-1 and the negatively-charged Hep would not be affected in such a wide pH range. By changing the pH values from 3 to 10, we measured the emission intensity of the TPE-1 probe (10 μM) in the absence and presence of 5.8 μg mL−1 Hep. As shown in Fig. 4b, the emission properties of TPE-1 and its Hep binding complex were not affected in the tested pH range from 3–10. This indicates that Hep sensing using TPE-1 as the probe fully meets the broad pH requirements.
OSCS detection assisted with heparinase treatment
After establishing the sensitive and selective method for Hep sensing in a wide pH range with the TPE-1 probe, we then proposed an approach for OSCS detection assisted with heparinase treatment (Scheme 2). Since the fluorescence of TPE-1 was turned on when it was assembled on Hep specifically, we envisioned that the cleavage of Hep by heparinases would disassemble TPE-1 from the cleaved Hep fragments and thus turn off the AIE fluorescence. To test our hypothesis, a cocktail solution of heparinase I, II and III was prepared and added into a solution containing 10 μM TPE-1 and 5.8 μg mL−1 Hep, and then incubated at 37 °C for fluorescence measurements. It clearly showed that the fluorescence intensity decreased sharply to 55% of the initial intensity after heparinase treatment (Fig. S8†). This result indicates that indeed the enhanced fluorescence of TPE-1 after Hep binding could be turned off by heparinase treatment.
Then the influence of OSCS on TPE-1 fluorescence before and after heparinase treatment was investigated. When TPE-1 in 10 mM, pH 7.4 HEPES/DMSO (95
:
5, v/v) was titrated with OSCS alone, the AIE fluorescence “turn on” of TPE-1 was observed (Fig. S9†). However, only 8-fold fluorescence enhancement was observed after addition of 6.6 μg mL−1 OSCS, while addition of 5.8 μg mL−1 of Hep enhanced the fluorescence by ∼31-fold. This indicates that OSCS can also bind to TPE-1 and turn on its fluorescence, but to a much lower degree than Hep. We then studied the inhibition effect of OSCS on heparinase activity. Even after 4 hours of the enzyme cocktail treatment, the fluorescence intensity of Hep with 1% OSCS was still stronger than that of Hep alone (Fig. S10†). Thus, the presence of OSCS indeed inhibited the activity of heparinases.
Based on the above interesting phenomena, a heparinase assisted strategy for detection of OSCS contaminants in Hep was developed using TPE-1 as follows (Scheme 2). The fluorescence intensities before and after the enzyme treatment were defined as Out1 and Out2, respectively. The ratio of Out2/Out1 was employed as the output signal for measuring different contents of OSCS in Hep. Different amounts of OSCS were spiked into Hep (w%: 0, 0.001, 0.01, 0.05, 0.1, 0.5, 1, 5, 10, 20, 30, 50, 70, 90 and 100), and then added to the solution of TPE-1 for fluorescence measurements, giving Out1. Then, these solutions were treated with the enzyme cocktail for 4 h, and the fluorescence intensities were recorded as Out2. The values of Out2/Out1 as a function of the amount of OSCS were plotted in Fig. 5a. It is obvious that with an increasing amount of OSCS, the value of Out2/Out1 increased accordingly. Thus the amount of OSCS contaminants in Hep can be quantified by TPE-1 with heparinase treatment in a signal “turn on” manner. The detection limit for OSCS was found to be 0.001% (w%), since the presence of 0.001% (w%) of OSCS induced a vivid change in Out2/Out1 (Fig. S11†). Besides, the value of Out2/Out1 shows a linear relationship to the % (w/w) of OSCS in Hep in a range of 1–70%, indicating that the presence of OSCS can be detected reliably with TPE-1 (Fig. 5b).
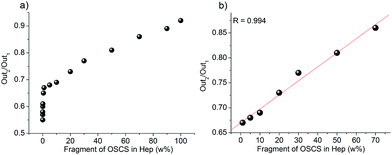 |
| Fig. 5 Heparinase assisted detection of OSCS contaminants in Hep with TPE-1. (a) Out2/Out1 as a function of the amount (w%) of OSCS in Hep. (b) The linear response curve of OSCS in Hep. Out1: fluorescence intensity measured upon addition of Hep (OSCS) to TPE-1; Out2: fluorescence intensity measured after 4 hours of the enzyme treatment of the aforementioned solution. | |
Conclusions
The conjugation of TPE, a typical AIE fluorogen, to the heparin-binding AG73 peptide afforded a “turn on” fluorescent probe TPE-1 for Hep detection. The fluorescence “turn on” behavior of TPE-1 was ascribed to the aggregation induced emission upon Hep binding. TPE-1 was sensitive towards Hep in a wide pH range with a detection limit of 3.8 ng mL−1, and showed high selectivity towards Hep over tested possible competitors, including various anions, biomolecules and especially Hep analogues such as Chs and HA. Moreover, the TPE-1 probe was applicable to sensing both UFH and LMWH. When further assisted with heparinase treatment, the OSCS contaminants in Hep were successfully determined with TPE-1. Compared with existing detection methods, TPE-1 showed an excellent sensitivity and selectivity towards Hep and OSCS (Tables S1 and S2†).18 In general, in this work we designed and constructed a fluorescent probe TPE-1 for efficient detection of both Hep and its OSCS contaminants in a reliable signal “turn on” manner. This work provides an ideal approach for designing “turn on” probes. It can be envisioned that a wider range of targets will be detected in the future when other specific biorecognition elements such as aptamers are conjugated.
Acknowledgements
We thank the National Natural Science Foundation of China (no. 21405081), the Natural Science Foundation of Jiangsu Province (no. BK20130561 and BK20140593), the 973 Program (no. 2015CB659400), the 985 Program of Nanjing University, the Priority Academic Program Development of Jiangsu Higher Education Institutions (PAPD), the Shuangchuang Program of Jiangsu Province, the Six Talents Summit Program of Jiangsu Province, Fundamental Research Funds for the Central Universities (no. 021314340052 and 021314340060), Open Funds of the State Key Laboratory for Chemo/Biosensing and Chemometrics (2014002), and the Thousand Talents Program for Young Researchers for financial support. We thank Professor Andrew G. Ewing for insightful discussions and Ms Min Zhou for nucleic acid samples preparation and measurements.
Notes and references
-
(a) I. Capila and R. J. Linhardt, Angew. Chem., Int. Ed., 2002, 41, 391–412 CrossRef;
(b) J. R. Bishop, M. Schuksz and J. D. Esko, Nature, 2007, 446, 1030–1037 CrossRef CAS PubMed;
(c) C. Guo, B. Wang, L. Wang and B. Xu, Chem. Commun., 2012, 48, 12222–12224 RSC.
-
(a) J. D. Esko and S. B. Selleck, Annu. Rev. Biochem., 2002, 71, 435–471 CrossRef CAS PubMed;
(b) C. Guo, X. Fan, H. Qiu, W. Xiao, L. Wang and B. Xu, Phys. Chem. Chem. Phys., 2015, 17, 13301–13306 RSC.
- D. L. Rabenstein, Nat. Prod. Rep., 2002, 19, 312–331 RSC.
- J. Hirsh and R. Raschke, Chest, 2004, 126, 188S–203S CrossRef CAS PubMed.
-
(a) B. Girolami and A. Girolami, Semin. Thromb. Hemostasis, 2006, 32, 803–809 CrossRef CAS PubMed;
(b) T. E. Warkentin, M. N. Levine, J. Hirsh, P. Horsewood, R. S. Roberts, M. Gent and J. G. Kelton, N. Engl. J. Med., 1995, 332, 1330–1335 CrossRef CAS PubMed.
-
(a) J. Bowers and J. J. Ferguson, Clin. Cardiol., 1994, 17, 357–361 CrossRef CAS PubMed;
(b) T. J. Cheng, T. M. Lin, T. H. Wu and H. C. Chang, Anal. Chim. Acta, 2001, 432, 101–111 CrossRef CAS.
-
(a) H. Qi, L. Zhang, L. Yang, P. Yu and L. Mao, Anal. Chem., 2013, 85, 3439–3445 CrossRef CAS PubMed;
(b) J. Guo and S. Amemiya, Anal. Chem., 2006, 78, 6893–6902 CrossRef CAS PubMed;
(c) G. Qu, G. Zhang, Z. Wu, A. Shen, J. Wang and J. Hu, Biosens. Bioelectron., 2014, 60, 124–129 CrossRef CAS PubMed;
(d) C. D. Sommers and D. A. Keire, Anal. Chem., 2011, 83, 7102–7108 CrossRef CAS PubMed;
(e) K. L. Gemene and M. E. Meyerhoff, Anal. Chem., 2010, 82, 1612–1615 CrossRef CAS PubMed;
(f) Z. L. Zhong and E. V. Anslyn, J. Am. Chem. Soc., 2002, 124, 9014–9015 CrossRef CAS PubMed;
(g) S. M. Bromfield, A. Barnard, P. Posocco, M. Fermeglia, S. Pricl and D. K. Smith, J. Am. Chem. Soc., 2013, 135, 2911–2914 CrossRef CAS PubMed;
(h) G. A. Crespo, M. G. Afshar and E. Bakker, Angew. Chem., Int. Ed., 2012, 51, 12575–12578 CrossRef CAS PubMed;
(i) T. N. Loegel, J. D. Trombley, R. T. Taylor and N. D. Danielson, Anal. Chim. Acta, 2012, 753, 90–96 CrossRef CAS PubMed;
(j) S. G. Elci, D. F. Moyano, S. Rana, G. Y. Tong, R. L. Phillips, U. H. F. Bunz and V. M. Rotello, Chem. Sci., 2013, 4, 2076–2080 RSC.
-
(a) J. Chan, S. C. Dodani and C. J. Chang, Nat. Chem., 2012, 4, 973–984 CrossRef CAS PubMed;
(b) S. Wang and Y.-T. Chang, Chem. Commun., 2008, 1173–1175 RSC;
(c) R. T. K. Kwok, J. Geng, J. W. Y. Lam, E. Zhao, G. Wang, R. Zhan, B. Liu and B. Z. Tang, J. Mater. Chem. B, 2014, 2, 4134–4141 RSC;
(d) D.-H. Kim, Y. J. Park, K. H. Jung and K.-H. Lee, Anal. Chem., 2014, 86, 6580–6586 CrossRef CAS PubMed;
(e) X. Gu, G. Zhang and D. Zhang, Analyst, 2012, 137, 365–369 RSC;
(f) H. S. Jung, X. Chen, J. S. Kim and J. Yoon, Chem. Soc. Rev., 2013, 42, 6019–6031 RSC;
(g) X. Li, X. Gao, W. Shi and H. Ma, Chem. Rev., 2014, 114, 590–659 CrossRef CAS PubMed;
(h) X. Qian and Z. Xu, Chem. Soc. Rev., 2015, 44, 4487–4493 RSC;
(i) Q. Dai, W. Liu, X. Zhuang, J. Wu, H. Zhang and P. Wang, Anal. Chem., 2011, 83, 6559–6564 CrossRef CAS PubMed;
(j) X. Sun, Q. Xu, G. Kim, S. E. Flower, J. P. Lowe, J. Yoon, J. S. Fossey, X. Qian, S. D. Bull and T. D. James, Chem. Sci., 2014, 5, 3368–3373 RSC;
(k) Y. Ding, Y. Tang, W. Zhu and Y. Xie, Chem. Soc. Rev., 2015, 44, 1101–1112 RSC.
- A. T. Wright, Z. L. Zhong and E. V. Anslyn, Angew. Chem., Int. Ed., 2005, 44, 5679–5682 CrossRef CAS PubMed.
-
(a) J. Liu, G. Liu, W. Liu and Y. Wang, Biosens. Bioelectron., 2015, 64, 300–305 CrossRef CAS PubMed;
(b) L. Cai, R. Zhan, K.-Y. Pu, X. Qi, H. Zhang, W. Huang and B. Liu, Anal. Chem., 2011, 83, 7849–7855 CrossRef CAS PubMed;
(c) Y. Cao, S. Shi, L. Wang, J. Yao and T. Yao, Biosens. Bioelectron., 2014, 55, 174–179 CrossRef CAS PubMed.
- Y. Ding, X. Li, T. Li, W. Zhu and Y. Xie, J. Org. Chem., 2013, 78, 5328–5338 CrossRef CAS PubMed.
- D. Ding, K. Li, B. Liu and B. Z. Tang, Acc. Chem. Res., 2013, 46, 2441–2453 CrossRef CAS PubMed.
- Y. Hong, J. W. Y. Lam and B. Z. Tang, Chem. Soc. Rev., 2011, 40, 5361–5388 RSC.
-
(a) M. P. Hoffman, J. A. Engbring, P. K. Nielsen, J. Vargas, Z. Steinberg, A. J. Karmand, M. Nomizu, Y. Yamada and H. K. Kleinman, J. Biol. Chem., 2001, 276, 22077–22085 CrossRef CAS PubMed;
(b) J. C. Sauceda, R. M. Duke and M. Nitz, ChemBioChem, 2007, 8, 391–394 CrossRef CAS PubMed.
- M. Guerrini, D. Beccati, Z. Shriver, A. Naggi, K. Viswanathan, A. Bisio, I. Capila, J. C. Lansing, S. Guglieri, B. Fraser, A. Al-Hakim, N. S. Gunay, Z. Zhang, L. Robinson, L. Buhse, M. Nasr, J. Woodcock, R. Langer, G. Venkataraman, R. J. Linhardt, B. Casu, G. Torri and R. Sasisekharan, Nat. Biotechnol., 2008, 26, 669–675 CrossRef CAS PubMed.
- T. K. Kishimoto, K. Viswanathan, T. Ganguly, S. Elankumaran, S. Smith, K. Pelzer, J. C. Lansing, N. Sriranganathan, G. Zhao, Z. Galcheva-Gargova, A. Al-Hakim, G. S. Bailey, B. Fraser, S. Roy, T. Rogers-Cotrone, L. Buhse, M. Whary, J. Fox, M. Nasr, G. J. Dal Pan, Z. Shriver, R. S. Langer, G. Venkataraman, K. F. Austen, J. Woodcock and R. Sasisekharan, N. Engl. J. Med., 2008, 358, 2457–2467 CrossRef CAS PubMed.
-
(a) L. Wang, S. Buchanan and M. E. Meyerhoff, Anal. Chem., 2008, 80, 9845–9847 CrossRef CAS PubMed;
(b) R. B. C. Jagt, R. F. Gomez-Biagi and M. Nitz, Angew. Chem., Int. Ed., 2009, 48, 1995–1997 CrossRef CAS PubMed;
(c) S. Bairstow, J. McKee, M. Nordhaus and R. Johnson, Anal. Biochem., 2009, 388, 317–321 CrossRef CAS PubMed;
(d) C. D. Sommers, D. J. Mans, L. C. Mecker and D. A. Keire, Anal. Chem., 2011, 83, 3422–3430 CrossRef CAS PubMed;
(e) M. Kalita, S. Balivada, V. P. Swarup, C. Mencio, K. Raman, U. R. Desai, D. Troyer and B. Kuberan, J. Am. Chem. Soc., 2014, 136, 554–557 CrossRef CAS PubMed.
-
(a) M. Wang, D. Zhang, G. Zhang and D. Zhu, Chem. Commun., 2008, 4469–4471 RSC;
(b) K.-Y. Pu and B. Liu, Adv. Funct. Mater., 2009, 19, 277–284 CrossRef CAS PubMed;
(c) H. Liu, P. Song, R. Wei, K. Li and A. Tong, Talanta, 2014, 118, 348–352 CrossRef CAS PubMed.
Footnote |
† Electronic supplementary information (ESI) available: Experimental details, synthesis and characterization of TPE-1, additional fluorescence measurement data. See DOI: 10.1039/c5sc01675d |
|
This journal is © The Royal Society of Chemistry 2015 |
Click here to see how this site uses Cookies. View our privacy policy here.